Keywords
Aortic occlusionCardiac output during aortic occlusionThe role of venous reservoir and of peripheral circulationHemodynamics of aortic occlusionCardiac effects of aortic occlusionProximal and distal aortic occlusionSuprarenal and infrarenal aortic occlusionMechanical occlusion of the aorta has been considered one of the classical interventions in cardiovascular physiology in the study of models of circulation. It is characterized by a complex hemodynamic response of great interest not only to cardiovascular physiologists but also to clinicians in the fields of vascular surgery and anesthesia. According to the pressure-propulsion model of the circulation, one would expect that a break of continuity between the pump and the system of vessels would result in an immediate decrease in flow. This, however, is not the case. Goodyer et al. showed that a progressive occlusion of the proximal aorta in a dog results in increase in peak ventricular systolic pressures (PVP) and a concomitant increase in diastolic pressures and volume (LVEDP and LVEDV), without change in CO (the phase of complete compensation). During the subsequent stage (partial compensation), there is a further increase in PVP, LVEDP, and LVEDV and only a slight drop in CO. Only during the final phase is there a sharp drop of CO and a maximal increase in PVP pressure (200–250 mmHg) and LVEDV (25 mmHg) (phase of decompensation) [1]. It is little surprising that in spite of the large number of animal and clinical studies, information regarding the underlying pathophysiology and regulation of cardiac output during aortic occlusion remains contradictory, with some reporting increase, decrease, or no change (For a review, see [2, 3]). We will now briefly examine some of the major circulatory effects of aortic occlusion on the peripheral circulation and on the heart.
18.1 Systemic Circulation
Historically, as early as 1886 De Jager ascribed the rise in carotid and central venous pressures in response to aortic occlusion in anesthetized dogs to the shift of blood from arterial to venous compartments, caused by “elastic energy accumulated in the coats of arteries.” De Jager also noted that occlusion of the inferior vena cava results in a drop of arterial pressure to about half of normal values and of the central venous pressure to zero. He thought it particularly significant that after a temporary release and re-occlusion of the IVC, there was a rise in CVP which he interpreted as venous stasis due to weakening and inadequate diastolic suction of the right ventricle [4].
Arterial pressures, systemic flows, and right atrial pressures in dogs at control conditions and after sequential occlusions of thoracic aorta, superior vena cava, inferior vena cava, and brachiocephalic artery
Control | Thoracic aorta occluded | Superior vena cava occluded | Inferior vena cava occluded | Brachiocephalic artery occluded | |
---|---|---|---|---|---|
Arterial pressure (mmHg) | 70 | 140 | 50 | 32 | 100 |
Systemic flow (mL min−1) | 792 | 990 | 360 | 125 | 792 |
Right atrial pressure (mmHg) | 5.1 | 5.8 | 4.1 | 3.2 | 4.1 |
The findings showed that in spite of profound changes in flows and arterial pressures affected by occlusion of the great vessels, there was relatively little variation in the corresponding RAPs [7]. In order to confirm that the heart indeed “reacts to all changes in the peripheral circulation by maintaining the central venous pressure constant”—as corroborated by Starling and coworkers only a few years earlier on the heart-lung preparation—Barcroft repeated the vessel-occlusion experiments in animals whose heart had been replaced by a mechanical pump [8]. The results indeed showed that, during aortic occlusion, the pump flows had to be increased by up to 60% above baseline to maintain constant RAPs. The pump flows during occlusion of other great vessels, on the other hand, were comparable to baseline flows before occlusion. In view of these findings, Barcroft made the following comment: “The striking thing is that the healthy heart maintained the venous pressure practically constant in the face of flow changes from 990 to 125 c.c. per min., and arterial blood pressure changes of 140 to 32 mm of mercury. Broadly speaking this suggested that, in these experiments, the heart maintained the venous pressure at zero, pumping away what returned to it irrespective of arterial pressure” [8].
Barcroft therefore concluded that the increase in CO following aortic occlusion results from factors related to the peripheral circulation, citing the model proposed by Krogh by which the blood is transferred from a large, highly compliant hepatosplenic reservoir into the low-compliance, upper body compartment with concomitant increase in venous return [9].
These experiments were repeated almost half a century later on anesthetized, atropinized dogs by Stokland and coworkers [10]. In addition to implanting snares around the descending thoracic aorta and IVC, the internal mammary artery and the azygos vein were ligated to prevent major vascular communication between the upper and lower parts of the body. Left ventricular dimension, i.e., the myocardial chord length (MCL), was monitored via a pair of miniaturized ultrasonic probes implanted on the anterior aspect of the LV wall. The results showed that occlusion of the descending aorta results in doubling of SVC flows, while the flow through IVC stabilized at 10% of control. Unlike in Barcroft’s experiments where aortic occlusion resulted in a marked increase in CO, the combined flow through SVC and IVC reached only about 80% of control value. The left ventricular systolic pressure (LVP) increased from 102 ± 7 to 188 ± 11 mmHg and LVEDP rose from 3 to 8 mmHg, the latter corresponding to an increase in MCL by 10%.
Simultaneous occlusion of the descending aorta and IVC resulted in cessation of flow in the IVC and caused MCL to diminish by an average of 38%, while LVP and flows in the SVC remained the same as during the aortic occlusion. Remarkably, after reaching a “steady-state” (usually after 2–3 min), the systolic LVPs and SVC flows during combined aortic and SVC occlusion were virtually the same as during control (pre-occlusion) state.
A heightened “sensitivity to blood volume” during combined aortic and SVC occlusion was demonstrated by infusion of 50 mL aliquots of blood into the jugular vein to the total cumulative volume which restored myocardial dimensions and SVC flows to pre-IVC occlusion levels. Subsequent withdrawal of blood from the upper body compartment to pre-infusion control values suggested a poor correlation between hemodynamics and perturbation in blood volumes. They proposed that about 7–8% of total blood volume was transferred from the lower to upper body vascular compartment, presumably via venous communications in the spinal canal. On the basis of their data Stokland and coworkers concluded that, according to this model, redistribution of blood volume due to “vascular collapse” in the lower body—and subsequent activation of the Frank-Starling mechanism via increase in LV end-diastolic volume—“completely accounts for hemodynamic response” during descending thoracic aortic occlusion [10].

Right heart bypass preparation used for descending aortic occlusion experiments in a dog by Stene and coworkers. Blood returning to the right atrium flows via Starling resistor to control right atrial pressure and is delivered into the pulmonary artery, bypassing the right ventricle. Snares show levels of aortic and brachiocephalic artery occlusion. RA right atrium, PA pulmonary artery, CA carotid artery, FA femoral artery. (Reproduced from ref. [11], used with permission of the American Physiological Society)
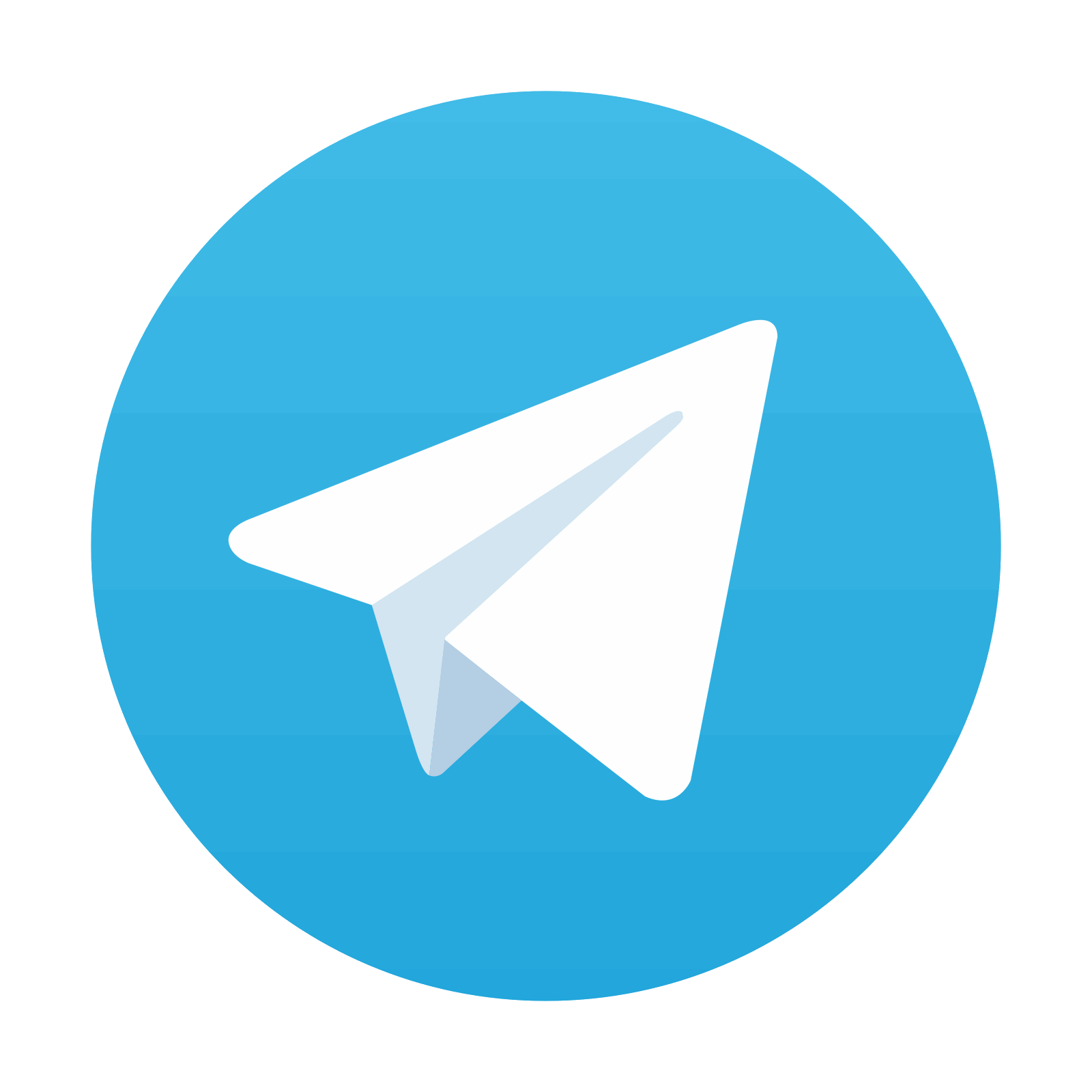
Stay updated, free articles. Join our Telegram channel

Full access? Get Clinical Tree
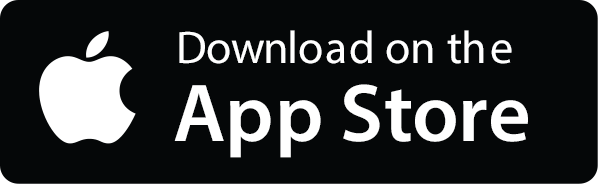
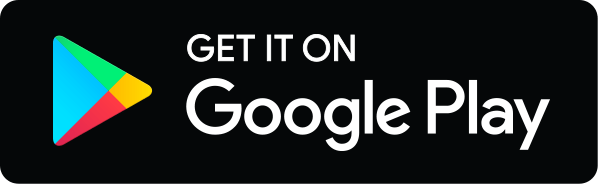