In this study, we aim to assess whether remote ischemic preconditioning (RIPC) reduces platelet activation during coronary angiography (CA) and/or percutaneous coronary interventions. We studied 30 patients who underwent CA because of a suspect of stable angina. Patients were randomized to RIPC (3 short episodes of forearm ischemia) or sham RIPC (controls) before the procedure. Blood samples were collected at baseline, at the end of the procedure, and 24 hours later. Monocyte–platelet aggregate (MPA) formation and platelet CD41 in the MPA gate and CD41 and CD62 expression in the platelet gate were assessed by flow cytometry, in the absence and in the presence of adenosine diphosphate (ADP) stimulation. A significant increase in platelet activation occurred during the invasive procedure in controls, which persisted at 24 hours. However, compared with controls, RIPC group showed no or a lower increase in platelet variables, including MPA formation (p <0.0001) and CD41 (p = 0.002) in the MPA gate and CD41 (p <0.0001) and CD62 (p = 0.002) in the platelet gate. ADP increased platelet activation at baseline, but did not further increase platelet reactivity during the invasive procedure in either groups. Percutaneous coronary interventions, performed in 10 patients (6 in the RIPC group and 4 in controls), did not have any further significant effect on platelet activation and reactivity compared with CA alone. In conclusion, RIPC reduces platelet activation occurring during CA. In contrast, no effects were observed on platelet response to ADP stimulation, probably related to the administration of an ADP antagonist in all patients.
Acute stent thrombosis is a rare but severe complication of percutaneous coronary interventions (PCIs) and may also occur during elective coronary procedures, despite preventive full antithrombotic treatment. Plaque disruption during PCI is in fact a strong stimulus for activation of prothrombotic events at the site of the procedure, which include strong platelet activation. Yet, catheter manipulation and use of contrast dye during simple diagnostic coronary angiography (CA) might already lead to platelet activation and a prothrombotic state, which might contribute to periprocedural adverse events. Remote ischemic preconditioning (RIPC) consists in a reduction of myocardial damage caused by prolonged ischemia when the latter is preceded by the application of intermittent episodes of ischemia to organs or tissues other than the heart, usually arms or legs. We have recently shown that upper arm intermittent ischemia is also able to reduce the increase in platelet activation and reactivity that typically occurs in some conditions as physical exercise and radiofrequency ablation for atrial fibrillation. In this study, we aimed at investigating whether RIPC also limits platelet activation occurring during diagnostic CA and PCI.
Methods
We studied 30 consecutive patients who were admitted to our Cardiology department to undergo elective CA because of suspected stable angina. Patients were excluded if they had any evidence of some other cardiovascular disease or relevant systemic disease, including acute or chronic inflammatory or allergic diseases. A detailed clinical history was collected from all patients, including assessment of cardiovascular risk factors. Written informed consent for participation in the study was obtained from all patients. The study was approved by our institutional ethical review board.
Patients were randomized with a 1:1 ratio to receive RIPC (n = 15) or sham intermittent ischemia (n = 15) immediately before starting the invasive procedure, using a computer-generated table of random numbers. RIPC was induced following a method described in detail elsewhere. Shortly, three 5-minute episodes of forearm ischemia were induced by cuff sphygmomanometer inflation, separated by 5 minutes of reperfusion. The cuff of the sphygmomanometer was placed in the standard position to the right arm and inflated to a pressure value 50 mm Hg greater than the systolic blood pressure of the patient. In the sham control group, the cuff was inflated for 3 times at 10 mm Hg for 5 minutes, with 5-minute intervals.
All patients received aspirin 100 mg and clopidogrel 300 mg on the day before CA, that was performed by a radial artery approach. Unfractionated heparin was administered throughout the procedure with dosing adjusted to achieve a target activated clotting time of >250 seconds. Patients only underwent CA when either no significant coronary stenosis or severe coronary artery disease indicating bypass surgery were found, whereas a PCI with one or more drug-eluting stent implantation was performed when indicated and feasible.
The occurrence of complications was carefully monitored during the coronary procedure and in the 24 to 48 hours after the procedure. High-sensitivity troponin T serum levels were measured at baseline and 6 hours and 24 hours after the invasive procedure using a widely available commercial kit (Roche Diagnostics, Mannheim, Germany).
Platelet activation and reactivity were assessed by flow cytometry measurements of monocyte–platelet aggregates (MPAs) and the expression of platelet receptors glycoprotein (GP) IIb/IIIa (CD41) and P-selectin (CD62), following methods described in detail elsewhere. Shortly, blood samples of 15 ml were collected from the right brachial vein of patients by a 6F venous sheath: (1) at baseline, before RIPC/sham intermittent ischemia; (2) immediately after the end of the invasive procedure (CA only or PCI); and (3) 24 hours after the end of the procedure. Blood was drawn directly into plastic tubes containing 3.8% buffered sodium citrate, after discarding the first 5 ml to minimize the formation of platelet aggregates. Blood was kept at room temperature and immune-labeled within 10 minutes of collection for analyses by flow cytometry. Appropriate fluorochrome-conjugated isotype-matched monoclonal antibody, obtained from the different manufactures, were used as control for background staining.
To assess the MPAs, blood (100 μL) was labeled within 10 minutes of collection with a saturating concentration of peridinin chlorophill protein complex-conjugated CD14 (lipopolysaccharide protein receptor) and fluorescein isothiocyanate-conjugated Glycoprotein IIb/IIIa (GP IIb, CD41; Becton-Dickinson, Milan, Italy) for 15 minutes at room temperature. After incubation, erythrocytes were lysed with buffered ammonium chloride and analyzed by a flow cytometer (FACScan; Becton-Dickinson). MPAs were identified using the logical gating facility by combination of binding characteristics of anti-CD14 (monocyte marker) and of anti-CD41 (platelet marker) antibodies. A minimum of 3,000 monocytes were counted for each test. MPAs were measured as percentage (%) of monocytes binding platelets and as platelet CD41 mean fluorescence intensity (mfi) in the monocyte–platelet gate.
To assess platelet surface receptors, blood was diluted 1:10 in phosphate-buffered saline and labeled within 10 minutes of collection by incubation with specific antibodies. Blood aliquots (5 ml) were incubated for 15 minutes at room temperature with saturating concentrations of phycoerythrin-conjugated CD41 and fluorescein isothiocyanate-conjugated P-selectin (CD62P; Becton-Dickinson).
After incubation, samples were diluted with 200 ml of phosphate-buffered saline and immediately analyzed by a flow cytometer Becton-Dickinson FACScan. An acquisition gate was first established on forward-scatter/side-scatter signals. These were collected in a logarithmic mode to improve discrimination between viable platelets and unwanted events (erythrocytes, white blood cells, debris, and aggregates). The purity of the gate was always confirmed by backgating on CD41 staining. A low flow rate was used to minimize coincident events. A minimum of 10,000 platelets were counted for each test. Platelet receptor expression was expressed as mfi of CD41 and of CD62P.
To assess platelet reactivity to agonistic stimulation, samples were incubated with adenosine diphosphate (ADP; 10 −7 M) for 15 minutes at room temperature and labeled and analyzed as previously described.
Based on data and results of our previous study, we calculated that we need 10 patients per group to have an 80% power to detect as significant at p <0.01 a difference between the 2 groups of 2.5% for MPA and 2.5 mfi for any other platelet receptor expression, assuming a common SD of variables of 1.5. To further increase the statistical power of the study, even considering the need for multiple comparisons, the number of patient to enroll was established in 15 per group.
Kolmogorov–Smirnov test revealed that the distribution of variables did not significantly differ from normal. Comparisons between groups of baseline continuous variables were done by t test. A generalized linear model for repeated measures was applied to compare the curves of platelet activation markers throughout the CA procedure between the 2 groups. In case of global significant differences, post hoc multiple between-group comparisons were performed using unpaired t test, with Bonferroni correction, to obtain evidence of where significant changes did occur. Differences were also adjusted for potentially confounding variables (smoking, diabetes, and kind of interventional procedure). Differences were considered significant when p <0.05. The same kind of analysis was performed to compare platelet response between patients who underwent CA only versus CA with PCIs. Continuous data are reported as mean ± SD. The SPSS, version 12.02, statistical software (SPSS Inc., Florence, Italy) has been used for statistical analyses.
Results
The main clinical characteristics of the 2 groups (RIPC and controls) are summarized in Table 1 . The 2 groups were comparable with regard to the main clinical and laboratory findings, as well as drug therapy and CA findings. The invasive procedure was undertaken without any clinically relevant complication in all patients. Overall, 15 patients (50%) had one or more significant coronary stenoses at angiography, 8 (53%) in the RIPC group and 7 (47%) in the control group (p = 0.70). Of the latter patients, 6 (40%) in the RIPC group and 4 (27%) in the control group (p = 0.70) underwent PCIs, receiving one or more drug-eluting stents, whereas the other 5 patients were judged to have either not treatable stenosis or indication to bypass surgery. Plasma troponin T did not differ between the 2 groups both at baseline and after the procedure.
Variable | RIPC (n=15) | Controls (n=15) | p |
---|---|---|---|
Age (years) | 66.3±12 | 67.7±10 | 0.73 |
Male/female | 15/0 | 12/3 | 0.22 |
Body mass index (Kg/m 2 ) | 26.8±3.2 | 27.5±2.7 | 0.50 |
Smoker | 8 (53%) | 10 (67%) | 0.71 |
Hypertension | 9 (60%) | 10 (67%) | 1.00 |
Hypercholesterolemia | 10 (67%) | 9 (60%) | 1.00 |
Diabetes mellitus | 4 (27%) | 1 (7%) | 0.33 |
Drug therapy | |||
ß-blockers | 11 (73%) | 12 (80%) | 1.00 |
Ca 2+ channel blockers | 4 (27%) | 2 (13%) | 0.65 |
Angiotensin converting enzyme-inhibitors/Angiotensin receptor blockers | 12 (80%) | 10 (67%) | 0.68 |
Statins | 10 (67%) | 6 (40%) | 0.27 |
Diuretics | 5 (33%) | 1 (7%) | 0.17 |
Antidiabetic drugs | 4 (27%) | 1 (7%) | 0.33 |
Coronary angiography | |||
Totally normal coronary arteries | 3 (20%) | 2 (13%) | |
Non obstructive stenosis | 4 (27%) | 6 (40%) | 0.18 |
Obstructive stenosis | 8 (53%) | 7 (47%) | |
Number of coronary arteries narrowed | |||
1 | 1 (13%) | 1 (14%) | |
2 | 2 (25%) | 2 (29%) | 0.98 |
3 | 5 (62%) | 4 (57%) | |
Percutaneous coronary intervention | 6 (40%) | 4 (27%) | 0.70 |
Troponin T (ng/mL) | |||
Basal | 0.02±0.01 | 0.03±0.06 | 0.45 |
Peak after coronary procedure | 0.09±0.17 | 0.06±0.13 | 0.67 |
The results of platelet markers in the absence of agonist ADP stimulation are summarized in Table 2 . There were no differences between the 2 groups at baseline in spontaneous MPA formation and platelet CD41 expression in the monocyte–platelet gate and in CD41 and CD62P platelet expression in the platelet gate.
Variable | RIPC (n=15) | Controls (n=15) | p ∗ |
---|---|---|---|
MPA (%) | |||
Baseline | 16±4.9 | 15.7±6.5 | |
End-procedure | 17±5.9 | 21.6±7.3 ¶ | 0.009 |
24-h after procedure | 17.9±6.3 | 25.0±11.0 † ¶ | |
CD41 in MPA gate (mfi) | |||
Baseline | 19.5±3.1 | 20.4±3.8 | |
End-procedure | 21.0±7.5 | 28.9±7.6 ‡ ¶ | 0.005 |
24-h after procedure | 22.8±7.6 | 32.1±6.7 ‡ ¶ || | |
CD41 in platelet gate (mfi) | |||
Baseline | 20.6±3.1 | 22.4±3.9 | |
End-procedure | 23.4±4.8 § | 27.4±5.9 ¶ | 0.05 |
24-h after procedure | 25.2±5.1 ¶ | 31.0±5.7 ‡ ¶ || | |
CD62 in platelet gate (mfi) | |||
Baseline | 9.7±1.7 | 10. 8±1.3 | |
End-procedure | 10.8±2.6 § | 14.5±4.2 † ¶ | 0.014 |
24-h after procedure | 11.1±2.3 | 15.3±4.5 ‡ , ¶ |
∗ p Values for differences between groups in group–variable interaction.
At the end of the procedure a significant increase of all markers of platelet activation was detected in controls, which persisted after 24 hours (p <0.05 for all). The increase, however, was absent or significantly blunted in the RIPC group compared with the control group ( Figures 1–4 ), with the differences persisting after adjustment for possible confounders, including diabetes, smoking, and kind of interventional procedure (angiography only or PCI).
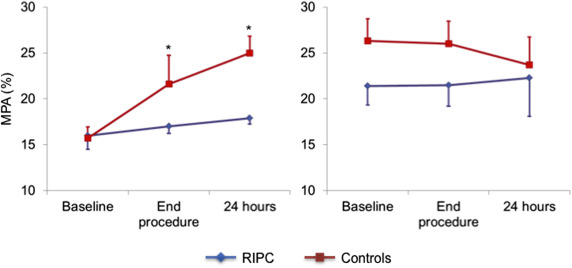
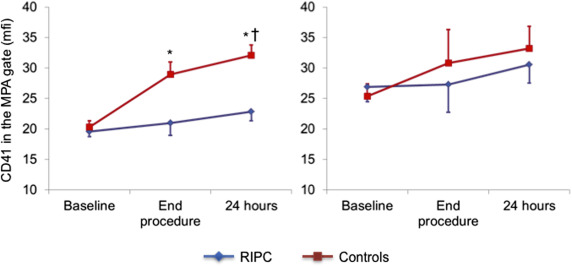
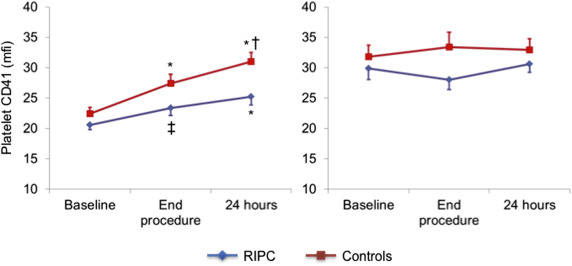
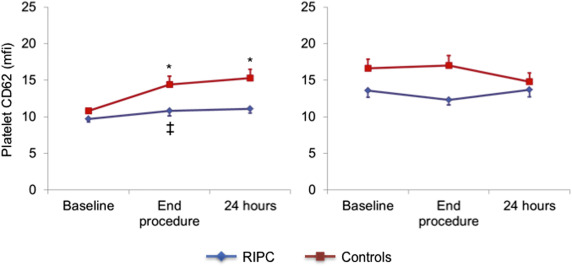
Platelet markers measured after ADP stimulation are summarized in Table 3 . ADP increased the expression of platelet markers in both groups, compared with the respective basal reference values. No significant further increase in platelet reactivity to ADP was found during the interventional procedure, compared with basal values, in both groups. Accordingly, there were no differences between the 2 groups in the trend of platelet reactivity in response to the interventional procedure.
