Keywords
Upright postureGravityLevityVenous valvesHydrostatic columnBaroreceptorsMedullary cardiovascular centerVestibulo-sympathetic reflexVascular siphonBrain perfusion in giraffeCerebral autoregulationBlood–brain barrierArchimedes principleIntracranial pressure curveCerebrospinal fluidChoroid plexusNegative intracranial pressureCirculation in microgravityVisual impairment intracranial pressure syndromeProlonged bed rest
Summary of principal organs and hormones involved in long-term (neurohormonal) control of total body water and arterial pressure. (Adapted from Motifolio Inc.)
The aim of this chapter is first to examine the physiologic circulatory response to upright posture and the levels of organization responsible for its maintenance.2 The controversial issue of the siphon effect on perfusion of the brain will be discussed in the context of environmental adaptations. Next, the salient features of cerebral blood supply and the dependence of brain function on buoyancy will be examined. Human exposure to microgravity removes hydrostatic gradients and causes a headward shift of blood and extracellular body fluids. Microgravity experiments suggest the existence of body’s own “buoyancy field” (upthrust) generated by total body water (60% of total body weight) which bathes the water-free elements consisting of connective tissue, fat, tendons, and bone matrix (cf. Fig. 15.2). This buoyancy vector is parallel with the long axis of the body and works in opposition to gravitational loading. The heart is positioned at the fulcrum between the forces of gravity and levity (buoyancy).
24.1 Circulatory Response to Upright Posture

Distribution of arterial (values in red) and venous (blue) pressures in upright and supine positions. (Zero reference point at the level of the right atrium.) When supine, arterial and venous pressures are evenly distributed along the horizontal axis. Upon standing, the arterial pressure is increased by the weight of blood column below the heart and decreased by equal value above the heart. Venous blood pools in lower part of the body (dark blue). Venous valves interrupt the column of blood and lessen the hydrostatic effect. Negative pressure in the chest and inside the cranium counteract the effect of gravity

Baroreceptor control of blood pressure. An increase in arterial pressure (red arrow)—sensed as static and dynamic stretch of the vessel wall and coupled to heartbeat—is detected by high-pressure receptors in the aortic arch and carotid sinus. The signal reaches the medullary cardiovascular center via the vagal and glossopharyngeal afferents. Pressure correction is achieved by slowing of the heart rate and peripheral vasodilation (solid blue arrow)
Cardiopulmonary baroreceptors are low-pressure sensors located in chambers of the heart and in pulmonary vessels. Their afferent impulses too, reach the MVC via the vagus nerve, while the efferent side of the reflex loop is mediated by the sympathetic fibers to skin and muscles [7]. According to a recent model of autonomic control, blood flow in the systemic circulation (with exception of organs with dual innervation, such as the heart, lung, eye, etc.) is governed exclusively by the sympathetic nervous system [8]. The model has provided important clues in the overall understanding of postural cardiovascular control.
Evidence accumulated over the past three decades shows that the vestibular organs, the utricle, and saccule (mediating the perception of steady, linear acceleration) and semicircular canals (transducing sudden and progressive angular acceleration) significantly modulate blood pressure during movement and changes in posture.3 These effects are integrated by vestibulo-sympathetic reflex (VSR) and are distributed via the sympathetic fibers to the skeletal muscles [9]. Direct microelectrode recordings of muscle sympathetic axons reveal that bursts of muscle sympathetic activity are coupled to the heartbeat. Significantly, arterial baroreceptors constitute an integral part of the loop. VSR differ from responses mediated by the high- and low-pressure baroreceptors in that they can be elicited before a change in blood distribution occurs in the body (anticipatory or feed-forward regulation, Chap. 23). Sympathetic nerve activity to the skin, on the other hand, is primarily involved in thermoregulation and mirrors the emotional state. Given that blood supply to the muscle and skin comprises such large part of cardiac output, anticipatory muscle and skin sympathetic activity contribute significantly to cardiovascular control during movement, the change in posture, and various emotional states [10].
Two reflex pathways appear to be responsible for optimal delivery of blood to the brain during a sudden change in posture; an immediate VSR system, and the high-pressure baroreceptor activation triggered with the above-mentioned inbuilt delay (5–15 s). A single loop with rapid resetting of high-pressure baroreceptors could be detrimental; a drop in arterial pressure might be sensed as a new “set point” leading to a downward spiral and cardiovascular collapse [7]. Of significance is the fact that VSR responses in animals are patterned in accordance to tissue type, e.g., blood vessels in the muscle, skin or viscera, as well as location (anatomical patterning) in the body (front vs. hind limbs). In humans, however, only tissue-specific patterning appears to exist [9].
While ample evidence points to the importance of the vestibular system in cardiovascular control in animals, its role in humans has been more difficult to ascertain. For example, lesions of the vestibular nerve result in profound disturbance in blood pressure regulation during head up tilts in anesthetized and paralyzed cats. However, no reports of significant blood pressure disturbances exist after surgical removal of the labyrinth in humans, suggesting that the mode of action of VSR may point to the real difference between bipedal humans and the quadrupeds [9]. Is it possible that a strong integration of the baroreceptor and vestibular reflexes in animals suggest a more complete integration into the gravitational field than is the case in humans?
24.1.1 Blood Column Informs Perception of Posture in Humans
Though it is widely acknowledged that visual and vestibular information plays a major role in maintaining orientation in space and locomotor equilibrium, the importance of extravestibular mechanoreceptors has only recently been demonstrated. Studies in patients with disturbed vestibular function (due to disease, trauma, or bilateral surgical removal of vestibular organ) showed that their ability to maintain an upright posture is virtually the same as that of normal controls, even in the absence of visual information [11]. It was traditionally assumed that these patients rely on proprioceptive information from joints, tendons and muscles and skin. Psychophysiological experiments by Mittelstaedt and coworkers on patients with labyrinthine dysfunction demonstrated, however, that somatic gravity information is mediated by the shifting column of blood in the large vessels [12]. This was confirmed by placing subjects on a sled centrifuge, rotating at constant speed, in a darkened room (to eliminate visual cues). Shifts of blood were measured by body pletysmography and controlled with application of lower body positive/negative pressure [13]. The experimental protocol was designed to neutralize the gravitational effects on vestibular organs so that the caudal and cranial shifts of blood were interpreted as a head-up or head-down tilt by the subjects, respectively. The findings suggest that in addition to vision, a separate information system for perception of posture exists in humans. It is mediated by the vertical distribution of “blood column” [14].
24.2 The Siphon Controversy

Gravity-dependent distribution of blood in a dog and in human. Due to gravitational pooling, the greater part of blood volume in the upright human is below the level of the heart. An estimated 70% of blood volume in quadrupeds is distributed above the heart. Anterior position of the heart in quadrupeds favors gravitational filling

The siphon principle. An open siphon is an inverted U-shaped tube used to deliver a flow of liquid above the surface of the reservoir without a pump, powered by the combination of gravitational pressure and suction created within the tube (a). Once primed, the uphill flow of liquid in the shorter tube of the siphon is independent of the height of the loop. Closed loop siphon (b). Proponents of the siphon effect maintain that the circulatory system consists of numerous such loops above and below the level of the heart (inverted siphon). Counterbalance of forces in the ascending (arteries) and descending limbs (veins) eliminates the need for additional energy on the part of the heart to overcome gravity
The siphon principle is based on Daniel Bernoulli’s energy conservation law (Hydrodynamica, 1738) stating that in an ideal liquid, where energy loses due to viscous resistance do not occur, the sum of hydrostatic pressure (P), gravitational potential energy (ρ.G.z), and kinetic energy (½ ρ.v2) remains constant and the values are interconvertible:

The question, however, whether the cardiovascular system operates according to the siphon principle surfaced specifically in relation to blood supply to the brain in humans and tall animals. The siphon effect has been the topic of a heated debate among the physiologists for well over a century.4 Hill and Barnard (1897) investigated the effects of gravity in anesthetized dogs in the upright posture and concluded that “the (siphon) doctrine is entirely fallacious, since the principle of the syphon is not applicable to the cardiovascular system in which arteries on the one hand and the veins on the other are of so very different distensibility and elasticity” [18]. More recently, Seymour and Johansen voiced a similar objection, maintaining that “…because of collapsible veins, gravitational pressure gradients are not matched in arterial and venous sides of circulatory loops above the heart as would be necessary for a siphon to operate” [19].
It is further argued that, if in a closed circulatory system, the heart is unable to support the hydrostatic column between the heart and the head, the flow would cease, resulting in cardiovascular collapse. This is exactly the reason that central arterial blood pressure is invariably greater than the hydrostatic pressure of the column of blood above the heart. Finally, the siphon opponents contend that the potential energy gained by the blood as it is pumped to the head is lost due to viscous resistance in the cerebral vascular beds and in partially collapsed veins of the neck. A simple laboratory model involving water flow in collapsible tubing is described by Seymour and coworkers in support of their claim [20].
Proponents of the siphon theory maintain on the contrary that the essential feature of Bernoulli’s relation is the gravitational force exerting equal, but opposite effects on a given volume of liquid in a vertical tube. As the liquid rises, the gravitational pressure decreases by ρ.G.z and the gravitational potential increases by the same value [21]. Experiments on hydraulic models demonstrate that flow in vertical collapsible tubes is no different from the flow in rigid tubes, both being equally subject to gravity [22]. What is essential, according to Hicks and Badeer, is that a distinction be made between an “open” system—in which gravity hinders uphill flow but causes the down-flow—and a “closed” system, as is the case in the circulation, where the heart provides the necessary pressure gradients to overcome viscous resistance (Fig. 24.5). A combined Bernoulli–Poiseuille equation has been proposed by Hicks and Badeer which, in addition to kinetic and potential energy losses, accounts also for heat dissipation due to frictional resistance [22].
24.2.1 Experimental Evidence in Animals

The effect of gravity on position of the heart in three types of snakes. In tree-climbing snakes, the closeness of the heart to the head and a slender body minimize gravitational pooling and assure adequate blood supply to the brain even during vertical climb (left). The effect of gravity is minimized in water snakes in which the heart is close to midline of the body (bottom, right). In terrestrial species the heart is positioned between the two extremes (top, right)
The mode of brain perfusion in an adult giraffe, with its head towering 2 or 3 m above the level of the heart, and a similar distance below the heart when stooping to drink, presents a different challenge to physiology. Despite the mean arterial pressure values being twice the value in humans, it has been claimed that cerebral perfusion in giraffe must be supported by the siphon effect [21, 22, 26]. A reference has already been made to the unique morphology of a giraffe’s heart that can maintain such high arterial pressures in the absence of left ventricular hypertrophy (cf. caption d, Fig 11.12).
Hargens and coworkers measured arterial and jugular vein pressures at different points along the giraffe’s neck in upright, sedated animals. Mean venous pressures were 16 mm Hg at the top and 7 mm Hg at the base of the neck, contrary to what would be expected in a column of blood over the same vertical distance (around 90 cm). These paradoxical values were thought to be caused by venous collapse and segmentation of the blood column. Anatomical dissection of the jugular veins showed greater density of the valves at the top of the neck and only a few at the base, suggesting the brain’s protection against the backflow of blood during grazing and drinking.5 While the arterial pressure of 110 mm Hg at the top and 190 mm Hg at the base of the neck supported the difference in hydrostatic height, the venous pressures were reversed from expected. Arterial pressures in the artery of the foot were highly variable during walking with values between 70 and 380 mm Hg. Venous and interstitial pressures ranged between −250 and +240, and −120 and +80 mm Hg, respectively. The authors ruled out the siphon effect [28].
These findings were confirmed in a recent study by Brøndum et al. who measured pressures in the aortic arch, carotid artery, and jugular vein in five anesthetized giraffes [29]. Spinal fluid pressure was determined in two animals at the level of cisterna magna. The animals were suspended in a sling in an upright position with the possibility of lowering the neck down to the level of the heart. The mean aortic pressures in the upright position was 193 ± 11 mm Hg and 118 ± 9 mm Hg in the carotid, a difference of 76 ± 4, consistent with the change in hydrostatic pressure. The jugular venous pressure at the cranial end was −7 ± 2 mm Hg and the central venous pressure 4 ± 2 mm Hg in the upright posture. The lowest recorded pressure at the cranial end of the jugular veins was −21 mm Hg.
On head lowering, the carotid pressure increased to 134 ± 7 mm Hg and the aortic pressure decreased to 131 ± 13 mm Hg. The cranial venous pressure increased to 30 ± 3 mm Hg and the central venous pressure dropped to −1 mm Hg. Carotid and jugular flows remained unchanged between the two positions as did the cross sections of the carotid artery. Jugular venous cross-section area changed from 0.14 cm2 in the upright to 3.19 cm2 in the head down position. No significant difference was found in the jugular and carotid flow between the two positions; however, the cardiac output decreased by 30% during head lowering. The spinal fluid pressures at the cisterna magna were 2 ± 0 mm Hg in the upright and 8 ± 0 mm Hg in the head down position. The authors concluded that the cerebral blood flow in an upright giraffe, though modified by gravity, is supplied by arterial pressure, without support of the siphon effect. They noted, moreover, that a siphon mechanism in a giraffe with 2 m long neck would have to generate either a negative pressures in excess of 100 mm Hg at the level of the head or a high pressure at the base of the jugular vein at the base of the neck, a value not consistent with experimental findings [29].
Even this brief review of the existing literature (many more references could be cited) shows that views on the effect of gravity on circulation are clearly divided with little prospect of resolution. It is difficult to see how the issue can be settled when physicists are yet to agree on the fundamental theory of the siphon effect. In addition to the conventional siphon theory by which gravity pulls the liquid down the descending pipe and creating suction in the ascending pipe,6 it has recently been demonstrated that siphons can operate in vacuum and to heights that exceed the so-called barometric limit of the liquid, i.e., the height when the column of fluid ruptures in the pipe (just short of 7 m for water). CO2 siphons and siphons with discontinuous flows which incorporate bubbles have also been described. Operation of these siphons clearly cannot be explained on principles based on gravity and atmospheric pressure (cf. Ref. [30] for a balanced discussion and further Refs. on the topic).
Amidst the flurry of conjectures Gisolf may have captured the core of the argument by stating that “In the siphon controversy, the role of the brain has curiously been overlooked” [31]. To obtain a better grasp of the issues involved, let us examine some unique features of the cerebral blood flow in humans.
24.3 Cerebral Circulation in Humans

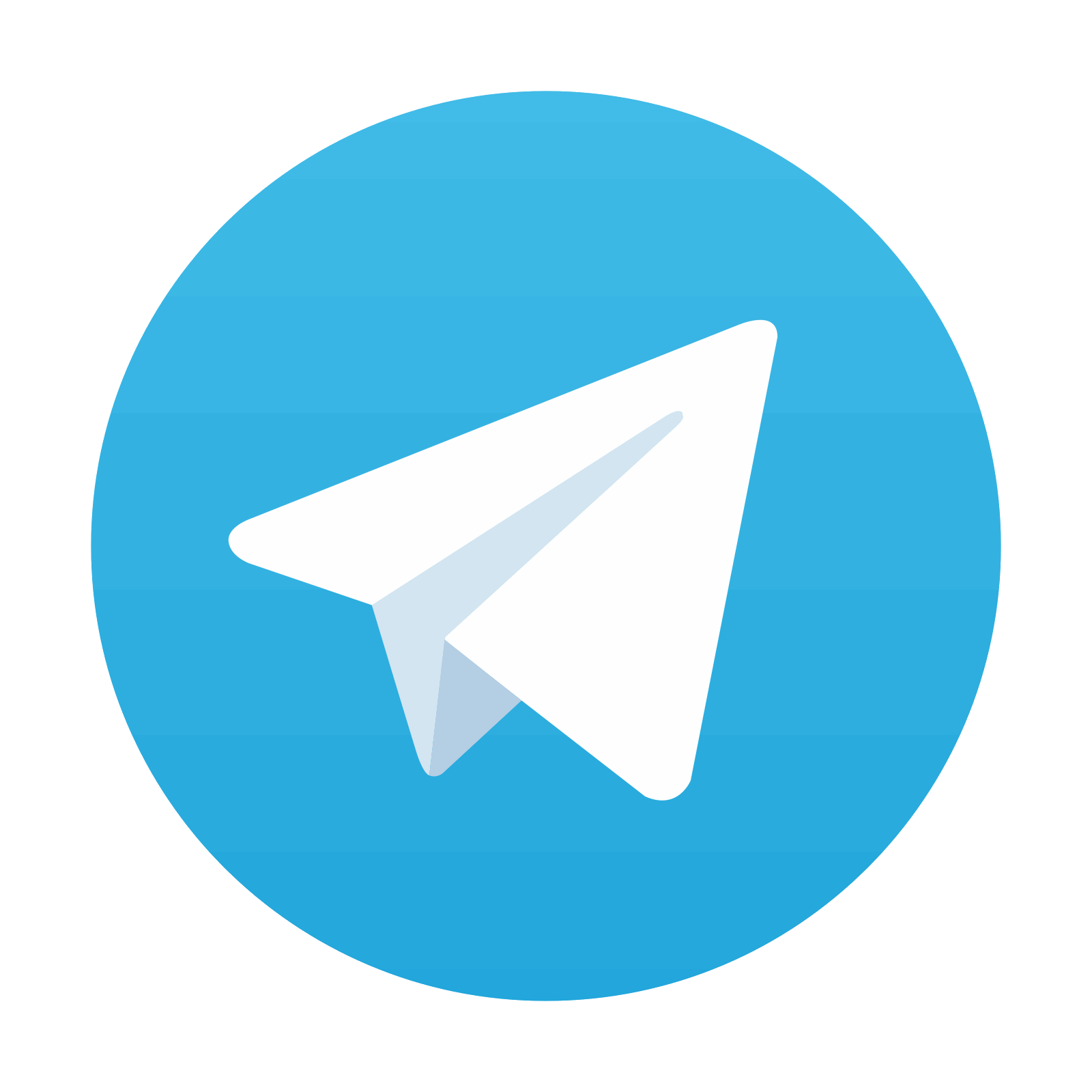
Stay updated, free articles. Join our Telegram channel

Full access? Get Clinical Tree
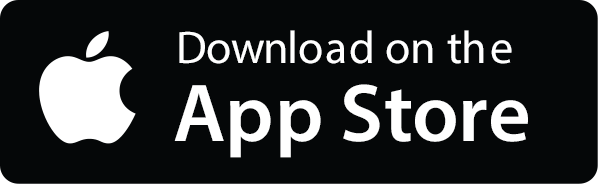
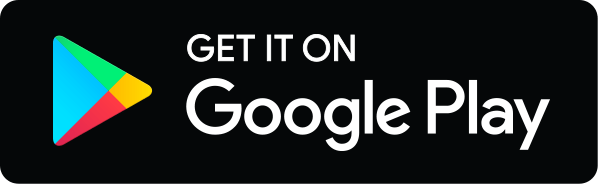