Background
Gravity affects every aspect of cardiac performance. When gravitational gradients are at their greatest on Earth (i.e., during upright posture), orthostatic intolerance may ensue and is a common clinical problem that appears to be exacerbated by the adaptation to spaceflight. We sought to elucidate the alterations in cardiac performance during preload reduction with progressive upright tilt that are relevant both for space exploration and the upright posture, particularly the preload dependence of various parameters of cardiovascular performance.
Methods
This was a prospective observational study with tilt-induced hydrostatic stress. Echocardiographic images were recorded at four different tilt angles in 13 astronauts, to mimic varying degrees of gravitational stress: 0° (supine, simulating microgravity of space), 22° head-up tilt (0.38 G, simulating Martian gravity), 41° (0.66 G, simulating approximate G load of a planetary lander), and 80° (1 G, effectively full Earth gravity). These images were then analyzed offline to assess the effects of preload reduction on anatomical and functional parameters.
Results
Although three-dimensional end-diastolic, end-systolic, and stroke volumes were significantly reduced during tilting, ejection fractions showed no significant change. Mitral annular e’ and a’ velocities were reduced with increasing gravitational load ( P < .001 and P = .001), although s’ was not altered. Global longitudinal strain (GLS; from −19.8% ± 2.2% to −14.7% ± 1.5%) and global circumferential strain (GCS; from −29.2% ± 2.5% to −26.0% ± 1.8%) were reduced significantly with increasing gravitational stress (both P < .001), while the change in strain rates were less certain: GLSR ( P = .049); GCSR ( P = .55). End-systolic elastance was not consistently changed ( P = .53), while markers of cardiac afterload rose significantly (effective arterial elastance, P < .001; systemic vascular resistance, P < .001).
Conclusions
Preload modification with gravitational loading alters most hemodynamic and echocardiographic parameters including e’ velocity, GLS, and GCS. However, end-systolic elastance and strain rate appear to be more load-independent measures to examine alterations in the cardiovascular function during postural and preload changes, including microgravity.
Highlights
- •
Gravity exerts important forces on the cardiovascular system, which must be countered in order to maintain homeostasis.
- •
In going from supine to vertical, there is a significant reduction in LV end-diastolic and end-systolic volumes, stroke volumes, and left atrial volume.
- •
Although GLS is sometimes touted as being load independent, we showed a significant fall in GLS from -19.8% to -14.7% in going from lying to standing.
- •
Clinical application of GLS should consider the impact of loading conditions.
Assessment of cardiac function is one of the most common indications for echocardiography. The manner by which cardiovascular performance determines stroke volume (SV) in any position is essentially dictated by preload, afterload, and left ventricular (LV) systolic and diastolic function. LV systolic function is often measured by ejection fraction (EF), but the EF is affected directly by preload and inversely by afterload.
Recently, myocardial deformation indices, such as strain and strain rate, have been proposed as accurate and relatively load-independent quantitative measures of cardiac function and a better prognostic marker than EF. However, the stability of these indices has been incompletely evaluated during preload-altering stress.
Gravitational forces play an essential role in determining the distribution of pressure and volume within the cardiovascular system. As part of National Aeronautics and Space Administration (NASA)-conducted Flight Study E377, detailed imaging studies were conducted on astronauts before space flights with various tilt angle settings to simulate changes with gravitational stress.
We hypothesized that preload reduction during gravitational gradient modifications with upright tilt would lead to alterations in cardiovascular performance in healthy individuals such as astronauts. We sought to elucidate the subsequent changes in hemodynamics and echocardiographic parameters including deformation indices during variations in preload induced by upright tilt. Such an understanding is critical to interpreting changes in a large majority of the echocardiographic measures including deformation indices.
Methods
Subjects
We studied 13 crew members who agreed to participate in Flight Study E377, “Cardiac Atrophy and Diastolic Dysfunction During and After Long Duration Spaceflight: Functional Consequences for Orthostatic Intolerance, Exercise Capacity, and Risk of Cardiac Arrhythmias,” conducted by NASA. As part of this investigation, detailed imaging studies were prospectively designed and conducted on astronauts before space flight with various tilt angle settings to simulate changes with gravitational stress. All astronauts were in excellent health, as determined by comprehensive NASA class III physical examinations, but were not elite athletes. No subject smoked or used any cardiovascular medication regularly. This research conformed to the Declaration of Helsinki, and all subjects signed an informed consent form approved by the NASA Human Subjects Review Committee and institutional review boards of the principal investigators’ institutions.
Tilt Study
Experiments were performed 79 ± 19 days (median, 78 [interquartile range, 66, 94]) prior to the launching day. Subjects refrained from high-intensity exercise and took no over-the-counter medication within 24 hours of all studies; no exercise of any sort was allowed 4 hours prior to study. Subjects were studied at least 2 hours after a meal; no alcohol was allowed for 24 hours or caffeine for 12 hours prior to study, and subjects were encouraged to get at least 6 hours of sleep the previous night. Experiments were conducted in a quiet, environmentally controlled laboratory with an ambient temperature of 25°C at Johnson Space Center, Houston, TX. Four different tilt angles were used to mimic varying degrees of gravitational stress relevant for spaceflight and given by the sine of the tilt angle ( Figure 1 ): 0° (supine, simulating microgravity of space), 22° head-up tilt (0.38 G, simulating Martian gravity), 41° head-up tilt (0.66 G, simulating the approximate peak G load predicted to occur during landing on a planetary surface or near-Earth object), and 80° head-up tilt (1G, effectively full Earth gravity). Following instrumentation, subjects rested at least 15 minutes in the supine position, after which supine echocardiography was performed. At each angle above 0°, echocardiography was begun 2 minutes after reaching that position and lasted for no more than 11 minutes. Based on our pilot data, using invasive measures of cardiac filling pressure during changes in hydrostatic gradients and monitoring reflex responses like muscle sympathetic nerve activity, we have observed that filling pressure (right atrial and pulmonary capillary wedge pressure) stabilizes within 30 seconds to 1 minute, and reflex responses (blood pressure [BP], heart rate, and muscle sympathetic nerve activity) are stable within 1-2 minutes. Images from all participants were acquired in the same, progressive order (0°, 22°, 41°, and 80° head-up tilt) without returning to zero angle prior to the next set of images ( Figure 2 ). BPs were assessed at the brachial artery using a commercially available oscillometric cuff monitor (Dynamap, Critikon, Tampa, FL).


Echocardiography
Echocardiographic examinations were performed by an experienced sonographer (D.S.M.) using a commercially available ultrasound system (iE33, Philips Medical Systems, Andover, MA), equipped with two-dimensional (2D) and three-dimensional (3D) transducers. At least three cardiac cycles were acquired in each of the standard echocardiography views and stored in digital imaging and communications in medicine (DICOM) format and raw data for analysis. Analysis was done by an experienced core lab technician, who was blinded to astronaut and condition. Two-dimensional LV end-diastolic (EDV) and end-systolic (ESV) volumes and EF were determined from the biplane method of disks. Three-dimensional EDV, ESV, and EF were analyzed using commercially available software (QLAB, Philips Medical Systems). The off-line software automatically identified the cavity wall interface in the 3D space throughout the cardiac cycle. Manual adjustments were performed, when necessary, to include the papillary muscles and trabeculae in the LV cavity. Biplane left atrial (LA) volume was measured at end systole.
SV was determined from the velocity time integral of the LV outflow tract (LVOT) pulsed-wave Doppler. The early filling (E-wave), the peak late diastolic (A-wave) velocities, and deceleration time were assessed from transmitral flow. The peak systolic (s′), early diastolic (e′), and late diastolic (a′) mitral annular velocities were measured at both the septal and lateral annulus using spectral Doppler and averaged. The ratio of early mitral diastolic inflow velocity to early diastolic mitral annular velocity (E/e′) was calculated. The averaged values from three cardiac beats were reported.
End-systolic BP (0.9 × systolic BP) was calculated as previously described. Total afterload was defined by the effective arterial elastance (Ea; 0.9 × systolic BP/SV). Systemic vascular resistance (SVR), the nonpulsatile component of afterload, was determined by dividing mean arterial pressure/cardiac output × 79.9. Total arterial compliance (TAC) was estimated from the ratio of SV to pulse pressure. Load-independent contractility was assessed using modified single-beat method end-systolic elastance (Ees; determined from BP, SV, and preejection and total systolic periods determined on LV outflow Doppler, EF, and an estimated normalized ventricular elastance at arterial end diastole). Ventricular-arterial coupling was assessed by the coupling ratio (Ea/Ees). Stroke work was defined as SV times mean arterial pressure, and pressure-volume area was estimated as previously reported. The pressure-volume area consists of stroke work and potential energy and correlates linearly with myocardial oxygen consumption.
Myocardial Strain Analysis
Myocardial deformation analysis was performed using a commercially available speckle-tracking software (Cardiac Performance Analysis version 1.1, TomTec Imaging Systems, Unterschlessheim, Germany). DICOM images at native acoustic frame rates (48-86 frames/sec) were analyzed. Three apical views were used to obtain both peak systolic global longitudinal strain (GLS) and strain rate (GLSR), while short-axis views at the papillary muscle level were used for peak systolic global circumferential strain (GCS) and systolic strain rate (GCSR). Analyses of deformation indices were performed as described elsewhere. Briefly, the endocardial border was manually traced, and the software subsequently traced the border in the other frames automatically. In segments with poor tracking, the border was readjusted manually until adequate tracking was achieved. In fact, minor adjustment was required for the majority of the images to achieve optimal tracking quality. The deformation parameters from three cardiac cycles were averaged.
Observer Variability
To assess inter- and intraobserver variability, we randomly chose six subjects. Each of two observers (Z.B.P. and A.B.) obtained two separate measurements on each subject, for a total of four measurements per subject. Absolute intraobserver variability was calculated as a difference between the first and second measurement performed by the same observer on the same subject. Intraclass correlation coefficient was also calculated. The analogous procedure was used to assess interobserver variability, except that the two measurements obtained on the same subject were taken by different observers. Observer variabilities were expressed as average ± SD. To assess variability in volumetric assessment, we chose subjects who underwent similar exams some months apart and had 2D volumes ( n = 13) or 3D volumes ( n = 6) measured. From these, mean ± SD of the absolute difference in the volumes and the Pearson correlation coefficient are reported.
Statistical Analysis
All continuous variables are presented as mean ± SD, and categorical data are expressed as percentages, unless otherwise specified. Linear mixed model was used to handle the dependencies in repeated measurements within the same subject. Unstructured covariance matrix was chosen because it had the lowest Akaike information criterion. Missing values in biplane LA volumes were imputed from LA area from four-chamber view, systolic and diastolic BPs, and E/e’ using multiple imputation. Two-sided P < .05 was accepted as indicating statistical significance. All data were analyzed using IBM SPSS statistics version 20 (IBM, Chicago, IL) and STATA version 12 (StataCorp LP, College Station, TX).
Results
Among 13 crew, nine (69%) were male and their mean age was 49 ± 4 years, height 174 ± 8 cm, and weight 72 ± 12 kg (body surface area 1.9 ± 0.2 m 2 ). Their systolic, diastolic, and mean BPs remained stable throughout the tilt study ( P = .90, .10, and .29, respectively).
Significant volume alterations were observed, as tilt angle increased. Changes in individual crew members along with the average trend are shown in Figures 3 and 4 , whereas whole population means of each echocardiographic parameter are summarized in Table 1 . Both 3D and 2D EDV and ESV were gradually and significantly reduced between 0 G and 1 G (3D EDV by 14%, P < .001; 3D ESV by 12%, P = .002, respectively). Although SVs fell significantly (3D SV by 14% and LVOT SV by 37%, both P < .001), both 2D and 3D EFs showed no change ( P = .26 and .35). LA volume fell by 39% ( P < .001, >50% volume change in some), reflecting reduction in venous return and presumably LA pressure. Consequently, mitral E wave velocity fell ( P < .001), but there was no change in E/A ratio ( P = .37). Similar changes were demonstrated in mitral annular e’ and a’ velocities ( P < .001 and P = .001), although s’ was not altered during tilting ( P = .79). E/e’ did not change despite clear changes in loading conditions and if anything tended to increase with increasing gravitational stress.


Variable | Tilt stages | P value ∗ | |||
---|---|---|---|---|---|
0°, 0 G, space (microgravity) | 22°, 0.37 G, Mars | 41°, 0.66 G, intermediate | 80°, 0.99 G, Earth | ||
Systolic BP, mm Hg | 118 ± 10 | 108 ± 7 | 112 ± 11 | 117 ± 11 | .90 |
Diastolic BP, mm Hg | 72 ± 6 | 66 ± 7 | 69 ± 7 | 75 ± 6 | .10 |
Mean BP, mm Hg | 87 ± 7 | 80 ± 6 | 84 ± 7 | 90 ± 8 | .29 |
Heart rate | 56 ± 9 | 58 ± 11 | 59 ± 10 | 70 ± 13 | <.001 |
3D EDV, mL | 96 ± 24 | 91 ± 21 | 88 ± 21 | 83 ± 20 | <.001 |
3D ESV, mL | 33 ± 9 | 32 ± 7 | 32 ± 8 | 29 ± 6 | .021 |
3D SV, mL | 63 ± 15 | 59 ± 15 | 55 ± 14 | 54 ± 16 | <.001 |
3D EF, % | 65.8 ± 3.5 | 65.0 ± 3.3 | 63.8 ± 2.5 | 64.7 ± 4.3 | .35 |
2D EDV, mL | 110 ± 26 | 112 ± 30 | 101 ± 23 | 84 ± 27 | <.001 |
2D ESV, mL | 37 ± 11 | 35 ± 11 | 36 ± 11 | 28 ± 9 | .002 |
2D EF, % | 67 ± 5 | 69 ± 5 | 65 ± 6 | 65 ± 5 | .26 |
LVOT SV, mL | 78 ± 16 | 71 ± 14 | 57 ± 11 | 49 ± 14 | <.001 |
E wave, cm/sec | 81 ± 14 | 70 ± 22 | 62 ± 16 | 59 ± 9 | <.001 |
A wave, cm/sec | 53 ± 16 | 52 ± 14 | 46 ± 14 | 42 ± 13 | .015 |
E/A ratio | 1.6 ± 0.5 | 1.4 ± 0.4 | 1.4 ± 0.3 | 1.5 ± 0.5 | .37 |
Deceleration time, msec | 200 ± 44 | 226 ± 50 | 247 ± 59 | 256 ± 35 | <.001 |
2D LA volume, mL | 44 ± 17 | 37 ± 10 | 34 ± 11 | 27 ± 15 | .005 |
s’, cm/sec | 8.6 ± 1.1 | 8.5 ± 1.5 | 8.4 ± 1.2 | 8.5 ± 0.8 | .79 |
e’, cm/sec | 10.8 ± 1.4 | 9.4 ± 1.9 | 8.4 ± 1.0 | 6.6 ± 1.0 | <.001 |
a’, cm/sec | 8.4 ± 1.6 | 7.8 ± 1.4 | 7.2 ± 0.8 | 6.7 ± 1.2 | .001 |
E/e’ | 7.5 ± 1.2 | 7.4 ± 1.6 | 7.3 ± 1.4 | 9.1 ± 2.5 | .056 |
GLS, % | −19.8 ± 2.2 | −17.8 ± 2.5 | −16.2 ± 1.6 | −14.7 ± 1.5 | <.001 |
GLSR, /sec | −1.2 ± 0.2 | −1.2 ± 0.2 | −1.1 ± 0.2 | −1.1 ± 0.1 | .049 |
GCS, % | −29.2 ± 2.5 | −28.5 ± 2.4 | −27.5 ± 2.1 | −26.0 ± 1.8 | <.001 |
GCSR, /sec | −2.2 ± 0.3 | −2.2 ± 0.2 | −2.1 ± 0.3 | −2.3 ± 0.3 | .55 |
Ees, mm Hg/mL | 1.9 ± 0.5 | 1.7 ± 0.3 | 2.1 ± 0.5 | 2.6 ± 0.9 | .53 |
Ea, mm Hg/mL | 1.4 ± 0.3 | 1.4 ± 0.3 | 1.8 ± 0.4 | 2.3 ± 0.8 | <.001 |
Ventricular arterial coupling (=Ea/Ees) | 0.75 ± 0.12 | 0.84 ± 0.14 | 0.90 ± 0.10 | 0.97 ± 0.19 | .003 |
Stroke work, mm Hg mL | 6,832 ± 1,617 | 5,686 ± 1,166 | 4,789 ± 920 | 4,345 ± 1,316 | <.001 |
PVA, mm Hg mL | 10,689 ± 2,700 | 9,228 ± 1,832 | 8,655 ± 3,032 | 8,722 ± 4,562 | .17 |
SVR, dyne·s·cm −5 | 1,655 ± 259 | 1,609 ± 275 | 2,008 ± 293 | 2,158 ± 539 | .002 |
TAC, mL/mm Hg | 1.7 ± 0.3 | 1.7 ± 0.3 | 1.4 ± 0.4 | 1.3 ± 0.4 | <.001 |
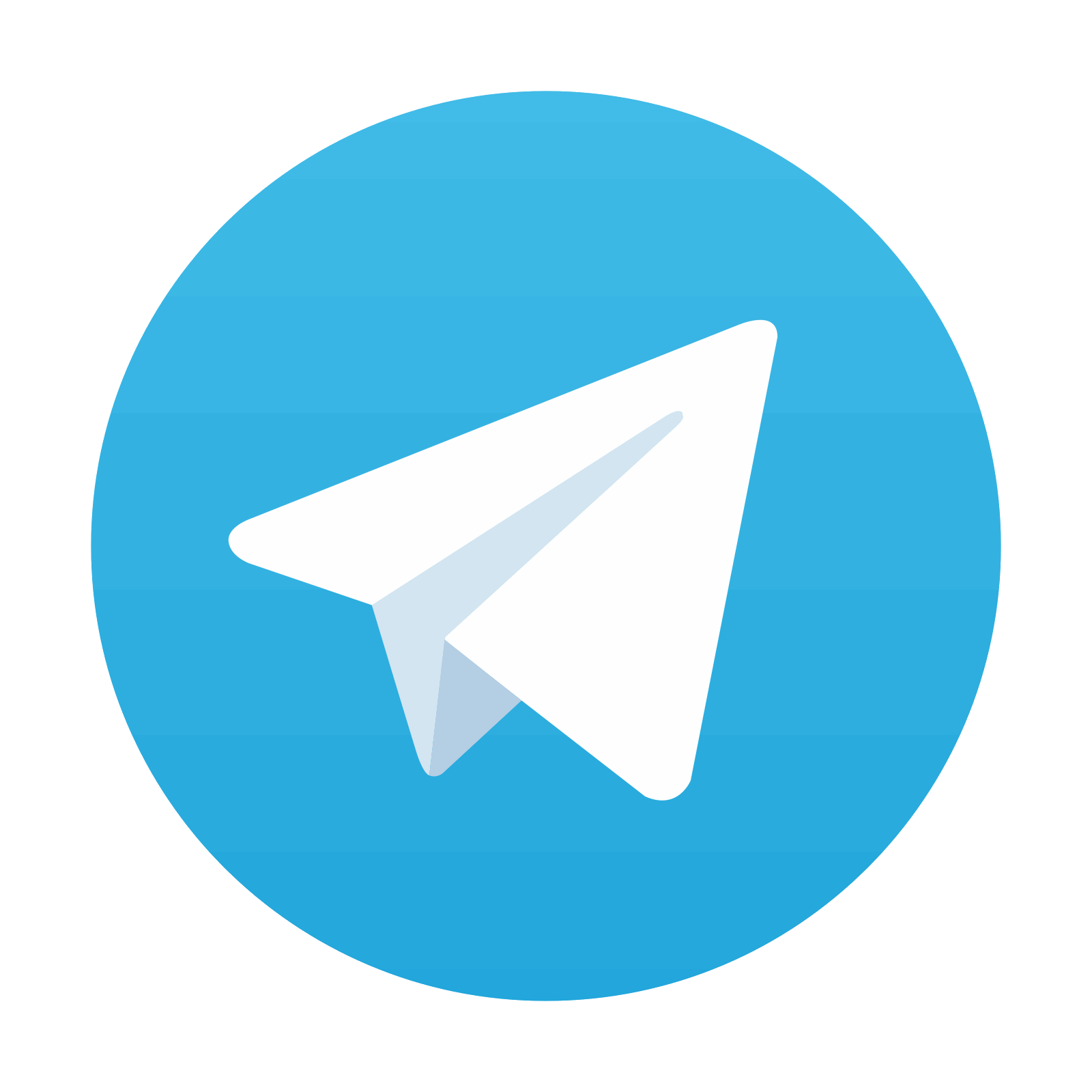
Stay updated, free articles. Join our Telegram channel

Full access? Get Clinical Tree
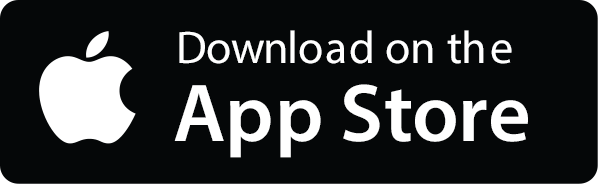
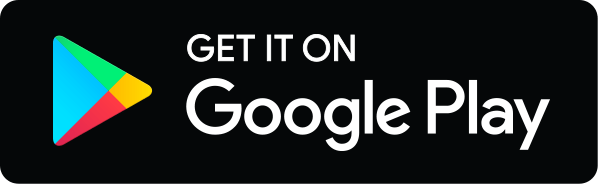
