Background
Accurate assessment of global and regional right ventricular (RV) systolic function is challenging. The aims of this study were to confirm the reliability and feasibility of a three-dimensional (3D) speckle-tracking echocardiography (STE) system, using comparison with cardiac magnetic resonance imaging (CMR), and to assess the contribution of regional RV function to global function.
Methods
In a retrospective, cross-sectional study setting, RV volumetric data were studied in 106 patients who were referred for both CMR and 3D echocardiography within 1 month. Three-dimensional STE-derived area strain, longitudinal strain, and circumferential strain were assessed as global, inlet, outflow, apical, and septal segments.
Results
Seventy-five patients (70%) had adequate 3D echocardiographic data. RV measurements derived from 3D STE and CMR were closely related (RV end-diastolic volume, R 2 = 0.84; RV end-systolic volume, R 2 = 0.83; RV ejection fraction [RVEF], R 2 = 0.70; P < .001 for all). RVEF and RV end-diastolic volume from 3D STE were slightly but significantly smaller than CMR values (mean differences, −2% and −10 mL for RVEF and RV end-diastolic volume, respectively). Among conventional echocardiographic parameters for RV function (tricuspid annular plane systolic excursion, fractional area change, S′ of the tricuspid annulus, RV free wall two-dimensional longitudinal strain), only fractional area change was significantly related to RVEF ( r = 0.34, P = .003). Among segmental 3D strain variables, inlet area strain ( r = −0.56, P < .001) and outflow circumferential strain ( r = −0.42, P < .001) were independent factors associated with CMR-derived RVEF.
Conclusions
RV volume and RVEF determined by 3D STE were comparable with CMR measurements. Regional RV wall motion showed that heterogeneous segmental deformations affect global RV function differently; specifically, inlet area strain and outflow circumferential strain were significant factors associated with RVEF in patients with underlying heart diseases.
Highlights
- •
We present a novel 3D speckle-tracking echocardiographic measurement system for the assessment of right ventricular systolic function.
- •
This system provides a reliable measurement of right ventricular systolic function using an echocardiographic methodology.
- •
The right ventricular regional strain derived by this system was revealed to contribute differently to the right ventricular ejection fraction.
Right ventricular (RV) systolic dysfunction, especially RV ejection fraction (RVEF), is crucial in the management of patients with heart diseases, including heart failure, pulmonary arterial hypertension, and structural heart disease. Cardiac magnetic resonance imaging (CMR) remains the gold-standard imaging tool for assessing RV volume and RVEF.
Three-dimensional (3D) echocardiographic imaging of the right ventricle has a theoretical advantage over the two-dimensional (2D) approach because 3D imaging is not affected by the through-plane phenomenon and can provide real information on volume and wall deformation with no assumptions regarding the complex RV shape. Several studies have reported comparisons between 3D echocardiography and CMR on RVEF and RV volume measurements. Recently, we developed novel RV 3D speckle-tracking echocardiography (STE) software validated using open-chest animal heart models with ultrasonic crystal implantations. This novel RV 3D speckle-tracking software provides three separate RV directional strain measurements, including global longitudinal, circumferential, and area strain and regional inflow, apex, and outflow functions. The advantage of this new software is that it enables measurements of regional strain in the inflow, outflow, and apical portions of the right ventricle, which is different from that of the left ventricle.
In this study, we sought to investigate several hypotheses: that this new 3D STE software specifically developed for the right ventricle would provide accurate quantitative assessment of RV end-diastolic volume (RVEDV), RV end-systolic volume (RVESV), and RVEF in a wide range of clinical entities, compared with CMR-derived measurements; that compared with conventional 2D echocardiographic indices, regional 3D RV strain would more strongly relate to CMR-derived RVEF; and that regional longitudinal, circumferential, and area strain would contribute differently to CMR-derived RVEF, reflecting intrinsic or acquired functional heterogeneity of the RV wall.
Methods
Study Population
We included 106 consecutive adult patients referred for clinically indicated CMR and echocardiography at Tsukuba University Hospital between May 2012 and April 2016 in a retrospective study setting. Transthoracic 3D echocardiography, focused on the right ventricle, was performed within 1 month of CMR. Serum brain natriuretic peptide (BNP) level within 1 month of CMR was measured. The study was approved by a local institutional review committee, and all subjects provided informed consent.
Conventional Echocardiography and 2D Strain
Echocardiographic examinations were performed with an ARTIDA ultrasound system (Toshiba Medical Systems, Tochigi, Japan). A 5-MHz transducer was used for conventional 2D echocardiography. Our echocardiography laboratory is maintained according to guidelines established by the Japanese Society of Echocardiography. Left ventricular volume and ejection fraction were measured using the modified Simpson rule. RV diameter and function were also measured using a standard method that assesses basal RV linear dimension, midcavity RV linear dimension, fractional area change, tricuspid annular plane systolic excursion (TAPSE), and peak systolic velocity of the tricuspid annulus (S′) using a tissue Doppler method. Furthermore, 2D speckle-tracking for RV free wall longitudinal contraction was performed using an RV-focused four-chamber view (2D Wall Motion Tracking; Toshiba Medical Systems).
Three-Dimensional RV Echocardiographic Acquisition
Full-volume electrocardiographically gated 3D RV images with six subvolumes were acquired using an appropriate duration of breath holding with a matrix-array 3D transducer. Echocardiographers were trained to obtain 3D images of the entire right ventricle, including the RV inlet, apex, and outflow, using a dedicated bed with an adjustable cut-out section located at the left chest area for optimal probe access when obtaining high lateral apical views. The size of the ultrasonic scanning angle was set as small as possible to obtain a high temporal resolution of >20 volumes/sec. Data were stored and transferred to a computer (Inspiron 1300; Dell, Round Rock, TX) for offline analysis. RV 3D speckle-tracking echocardiographic images were analyzed using prototype software for dedicated RV assessment (Toshiba Medical Systems).
Three-Dimensional Wall Motion Tracking Algorithm for the Right Ventricle
Details of the 3D wall motion tracking algorithm for the right ventricle were reported previously. Five cross-sectional images were obtained: a four-chamber RV inlet-to-apex view, coronal apex-to-outflow view, and three axial views for the tricuspid annular plane, mid-RV level, and apical level, respectively ( Figure 1 , top ). The end-diastolic frame was chosen at the beginning of the QRS complex of the electrocardiographic monitoring signal. Endocardial borders were traced manually in the coronal plane and the three axial planes of the end-diastolic frame. RV trabeculations and papillary muscles were included in the intraventricular blood volume. For anatomically faithful segmentation, the attachment sites of the moderator band in the ventricular septum and anterior papillary muscle in the free wall, which are the landmarks that divide the inlet, outflow, and apex segments, can be adjusted manually. The software automatically tracks the voxel pattern of the speckles frame by frame in 3D space ( Figure 1 , bottom ). Ultimately, the software produces a complete data set comprising RVEDV, RVESV, RVEF, longitudinal strain, circumferential strain, and area strain ( Figure 2 ). Longitudinal strain was defined as the percentage change in regional length in the direction of the longitudinal axis of the endocardium, circumferential strain was defined as the percentage change in regional circumference of the endocardium, and area strain was defined as the percentage change of the regional area of the endocardium, which can be regarded as the product of both longitudinal strain and circumferential strain. Manual correction of the endocardial border was prohibited after tracking was completed. Each segmental data set could be deleted from the results of strain analysis if tracking quality was determined to be inappropriate by eyeball judgment. Temporal changes in strain data were obtained as time-strain curves ( Figure 2 ). We defined each strain measurement as the peak systolic strain value globally and in seven segments: inlet lateral, inlet inferior, inlet septum, outflow septum, outflow free wall, apical free wall, and apical septum. The SD of the time to peak area strain of the seven segments was measured as the RV dyssynchrony parameter.


In the validation study of 3D STE-derived RV volume and systolic function, RVEDV, RVESV, and RVEF were compared with those derived from CMR. To evaluate the significance of echocardiographic measurements, correlations between the CMR-derived RVEF and conventional echocardiographic parameters or 3D STE-derived strain measurements were assessed.
CMR for RV Volume and Function
CMR examinations were performed with a 1.5-T superconducting unit (Philips, Best, the Netherlands) with a phased-array cardiac coil. Electrocardiographically gated cine mode images using a steady state were obtained in a short-axis view with 10-mm slice thickness without an intersection gap. The acquisition time was 10 to 16 sec while patients held their breath. CMR images were analyzed using offline software (ViewForum; Philips). From short-axis images, we selected the slice that contained the tricuspid valve and the slice that contained the apex of the right ventricle. Subsequently, we manually traced the endocardial contour of the right ventricle for each slice from the tricuspid level to the apex level, respectively, in the end-diastolic phase. An automatic trace function was used to determine the RV endocardial line of all phases. All lines were checked by an observer and manually corrected where necessary. The software automatically calculated RV volume data in each phase. Minimum volume was defined as the RVESV. RVEF was calculated using RVEDV and RVESV as follows: RVEF = (RVEDV − RVESV) × 100/RVEDV.
Reproducibility of 3D Echocardiography
RVEDV, RVESV, RVEF, and global longitudinal, circumferential, and area strain from 3D STE were used to assess intra- and interobserver reproducibility. To test intraobserver variability, a single observer analyzed 10 selected RV data sets on two occasions separated by an interval of >1 month. To test interobserver variability, a second observer analyzed the data without knowledge of measurements made by the first observer. We expressed intraobserver and interobserver variability using the coefficient of variation, which was defined as the SD of the difference between intra- and interobserver measurements and the mean value of that measurement.
Statistical Analysis
Data are shown as mean ± SD, number (percentage), median (interquartile range), or mean with 95% CI. Comparisons between 3D speckle-tracking echocardiographic and CMR data were performed using paired t tests. Data were statistically analyzed using linear regression and Bland-Altman analysis to determine the bias and limits of agreement between the two modalities. Comparisons between the two groups were performed using Student’s t test for continuous variables and the χ 2 test for categorical variables. Logarithmic transformation was performed for BNP analysis. The area under the receiver operating characteristic curve was calculated to determine the capability of echocardiographic parameters to detect CMR-derived RVEF impairment. A CMR-derived RVEF value of 30% has been shown to correlate with poor prognosis in previous studies and was therefore chosen as the cutoff value. Multivariate models were developed using stepwise regression with the Akaike information criterion to determine CMR-derived RVEF with regional RV strain. A P value < .05 was considered to indicate statistical significance. All statistical analyses were performed using JMP version 11 (SAS Institute, Cary, NC).
Results
Baseline Characteristics
Analysis of RV volumes and function was feasible in 75 patients (70%). The remaining 31 patients (30%) were excluded because of suboptimal 3D echocardiographic image quality. Reasons for exclusion included an absence of RV outflow tract (RVOT) echo contours in 26 patients, mildly unclear images from the apex to anterior wall in three patients, and an incomplete scanning angle with which to obtain the entire right ventricle in two patients. Among the 31 patients who were excluded from the 3D echocardiographic analysis, 25 (80%) had satisfactory 2D apical acoustic windows for measurement of TAPSE, S′, fractional area change, and 2D RV strain. RV contour assessment took 3 to 5 min for a trained analyzer but only 9 sec for 3D speckle-tracking by computer. The background characteristics of excluded and included patients were similar in terms of age, sex, height, and weight. The volume rate on 3D full-volume echocardiographic imaging was 31.6 ± 3.2 volumes/sec (range, 22.1–37.2 volumes/sec). Of these 75 patients, 42% were men, the mean age was 40 ± 18 years (range, 18–70 years), and the mean body surface area was 1.59 ± 0.19 m 2 ( Table 1 ). Thirty-nine patients (52%) had cardiomyopathy or pulmonary arterial hypertension, and the remaining 36 (48%) had congenital heart diseases, consisting of repaired tetralogy of Fallot (TOF) in 14, unrepaired TOF in two, and transposition of the great arteries with atrial switch in four and with arterial switch in two patients. A summary of RV size and function parameters measured by CMR, conventional 2D echocardiography, and the new 3D speckle-tracking echocardiographic analysis is presented in Table 2 .
Variable | Value |
---|---|
Age (y) | 40 ± 18 |
Men | 42 (57) |
Systolic blood pressure (mm Hg) | 117 ± 20 |
Diastolic blood pressure (mm Hg) | 65 ± 15 |
Heart rate (beats/min) | 64 ± 18 |
Height (cm) | 163 ± 9 |
Weight (kg) | 56 ± 12 |
Body surface area (m 2 ) | 1.59 ± 0.19 |
BNP (pg/mL) | 42 (24–118) |
Underlying heart disease | |
Noncongenital structural heart disease | |
Dilated cardiomyopathy | 24 (32) |
Hypertrophic cardiomyopathy | 11 (13) |
Arrhythmogenic cardiomyopathy | 3 (4) |
Pulmonary arterial hypertension | 1 (1) |
Congenital structural heart disease | |
TOF | 16 (22) |
TGA | 6 (8) |
ASD | 4 (5) |
VSD | 4 (5) |
Pulmonary stenosis | 4 (5) |
Ebstein’s anomaly | 2 (3) |
Variable | All ( N = 75) | Patients with RVEF > 30% ( n = 52) | Patients with RVEF ≤ 30% ( n = 23) | P ∗ |
---|---|---|---|---|
CMR | ||||
RVEDV (mL) | 127 ± 69 | 122 ± 58 | 140 ± 90 | .283 |
RVEDVI (mL/m 2 ) | 81 ± 44 | 77 ± 36 | 90 ± 57 | .236 |
RVESV (mL) | 84 ± 54 | 72 ± 37 | 111 ± 74 | .003 |
RVESVI (mL/m 2 ) | 54 ± 34 | 46 ± 23 | 71 ± 47 | .002 |
RVEF (%) | 35 ± 12 | 41 ± 8 | 21 ± 8 | <.001 |
2D echocardiography | ||||
LVEF (%) | 61 ± 14 | 60 ± 11 | 58 ± 16 | .531 |
TRPG (mm Hg) | 31 ± 22 | 28 ± 14 | 37 ± 32 | .139 |
Pulmonary flow velocity (m/sec) | 1.5 ± 1.0 | 1.5 ± 1.0 | 1.4 ± 1.1 | .710 |
RV linear dimension | ||||
Midcavity RV (mm) | 32 ± 13 | 31 ± 13 | 35 ± 14 | .209 |
Midcavity RV index (mm/m 2 ) | 20 ± 8 | 19 ± 8 | 22 ± 9 | .203 |
Basal RV (mm) | 43 ± 12 | 42 ± 13 | 46 ± 12 | .174 |
Basal RV index (mm/m 2 ) | 27 ± 8 | 26 ± 7 | 29 ± 8 | .232 |
RV DTI S′ (cm/sec) | 10 ± 4 | 10.5 ± 3.0 | 9.8 ± 3.4 | .487 |
TAPSE (mm) | 17.0 ± 5.4 | 16.6 ± 5.1 | 17.0 ± 6.0 | .263 |
Fractional area change (%) | 44 ± 15 | 47 ± 13 | 37 ± 19 | .007 |
RV free wall LS (%) | −16.0 ± 6.0 | −16.7 ± 5.2 | −14.2 ± 6.4 | .075 |
3D echocardiography | ||||
RVEDV (mL) | 118 ± 71 | 107 ± 55 | 142 ± 92 | .043 |
RVEDVI (mL/m 2 ) | 74 ± 43 | 68 ± 35 | 87 ± 55 | .069 |
RVESV (mL) | 81 ± 55 | 67 ± 36 | 112 ± 76 | <.001 |
RVESVI (mL/m 2 ) | 51 ± 34 | 42 ± 22 | 68 ± 46 | .001 |
RVEF (%) | 32 ± 11 | 37 ± 8 | 22 ± 8 | <.001 |
3D strain | ||||
Global LS (%) | −6.7 ± 3.5 | −7.7 ± 3.2 | −4.7 ± 3.3 | <.001 |
Global CS (%) | −8.0 ± 3.9 | −8.9 ± 3.8 | −6.2 ± 3.6 | .007 |
Global AS (%) | −17.5 ± 5.3 | −20 ± 4.5 | −13 ± 4.5 | <.001 |
SD of time to peak AS (msec) | 80 ± 46 | 74 ± 41 | 95 ± 54 | .031 |
Validation of CMR-Derived RV Volumetric Data and RVEF with 3D STE
RVEDV, RVESV, and RVEF showed strong relationships between CMR and 3D STE ( Figure 3 , left ; r = 0.94, r = 0.94, and r = 0.84, respectively, P < .001 for all). The Bland-Altman plot showed significant proportional underestimation bias for RVEDV (−9.1 mL, P = .002) and RVEF (−2.3%, P = .002) but not for RVESV (−1.7 mL, P = .099). The 95% limits of agreement between the two methods were −56.9 and 38.7 mL for RVEDV, −39.6 and 33.3 mL for RVESV, and −14.7% and 9.9% for RVEF ( Figure 3 , right ).

RV Function Measures Using CMR and Echocardiographic Methods
Moderate to modest correlations were found with respect to CMR-derived RVEF for global area strain ( r = −0.60, P = .004), global longitudinal strain ( r = −0.43, P < .001), global circumferential strain ( r = −0.32, P = .004), and fractional area change ( r = 0.34, P = .003). On the other hand, significant correlations were not observed for RV tissue Doppler imaging S′ ( r = 0.06, P = .75), TAPSE ( r = 0.02, P = .85), or RV free wall 2D strain ( r = −0.19, P = .07).
In patients with severely impaired right ventricles (CMR-derived RVEF ≤ 30%), RV strain was universally reduced for all vectors. The SD of time to peak area strain was significantly prolonged in the group with RV impairment compared with the group without RV impairment ( Table 2 ). Among the conventional echocardiographic parameters, only fractional area change decreased in the patients with severely impaired RV. On receiver operating characteristic analysis to determine the factors associated with severely impaired CMR-derived RVEF ( Table 3 ), only fractional area change and 3D strain for all vectors showed significant results. Among these parameters, 3D STE-derived RVEF showed the largest area under the curve (0.93, P < .001).
Variable | AUC | P |
---|---|---|
2D echocardiography | ||
RV DTI S′ | 0.57 | .481 |
TAPSE | 0.51 | .789 |
Fractional area change | 0.69 | .011 |
RV free wall LS | 0.64 | .069 |
3D echocardiography | ||
RVEF | 0.93 | <.001 |
3D global strain | ||
Global LS | 0.74 | <.001 |
Global CS | 0.70 | .005 |
Global AS | 0.84 | <.001 |
SD of time to peak AS | 0.62 | .068 |
Association Between Regional RV Strain and CMR-Derived RVEF
Most regional RV strain values, except for apical longitudinal strain and septal circumferential strain, were associated with severe RV dysfunction as defined by RVEF ≤ 30% on CMR ( Table 4 ). By using stepwise variable selection in both longitudinal and circumferential strain, the inlet longitudinal strain and inlet circumferential strain, and the RVOT circumferential strain were selected as the independent variables ( Table 4 , middle row ). Among all strain values, inlet area strain and outflow circumferential strain were selected ( Table 4 , right row ).
Strain | Univariate | Model 1 ( R 2 = 0.38) | Model 2 ( R 2 = 0.49) | |||
---|---|---|---|---|---|---|
R 2 | P | F | P | F | P | |
LS | ||||||
Inlet | 0.08 | .012 | 4.32 | .040 | .601 | |
Apex | .112 | |||||
Outflow | 0.07 | .036 | .606 | .689 | ||
Septum | 0.10 | .007 | .361 | .421 | ||
CS | ||||||
Inlet | 0.17 | <.001 | 8.73 | .004 | .326 | |
Apex | 0.08 | .021 | .464 | .521 | ||
Outflow | 0.25 | <.001 | 14.00 | <.001 | 17.70 | <.001 |
Septum | .239 | |||||
AS | ||||||
Inlet | 0.31 | <.001 | 26.23 | <.001 | ||
Apex | 0.08 | .002 | 3.16 | .080 | ||
Outflow | 0.27 | <.001 | .852 | |||
Septum | 0.11 | .004 | .801 |
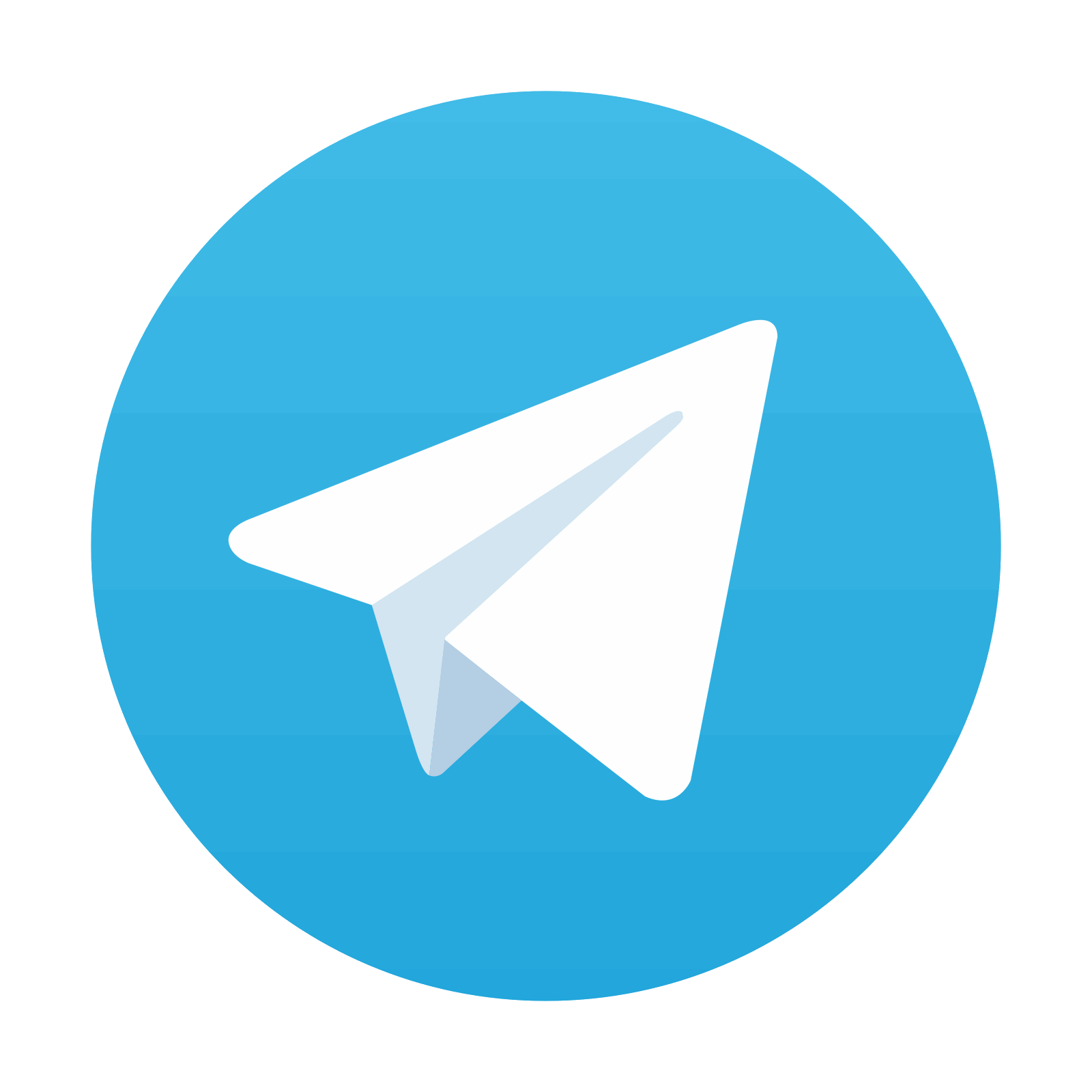
Stay updated, free articles. Join our Telegram channel

Full access? Get Clinical Tree
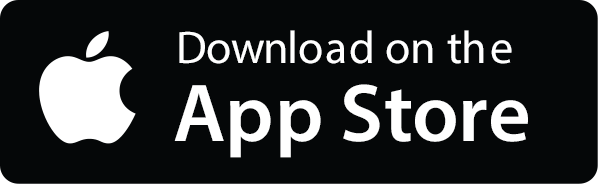
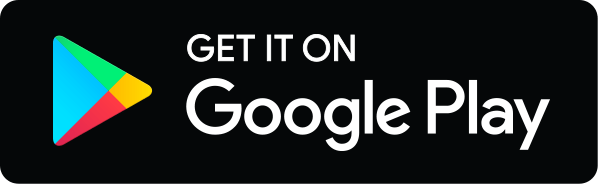
