Intense lifestyle modifications can change the high-density lipoprotein (HDL) cholesterol concentration. The aim of the present study was to analyze the early effects of short-term exercise training, without any specific diet, on the HDL cholesterol plasma levels and HDL functional characteristics in patients with the metabolic syndrome (MS). We studied 30 sedentary subjects, 20 with and 10 without the MS. The patients with the MS underwent moderate intensity exercise training for 3 months on bicycle ergometers. Blood was sampled before and after training for biochemical analysis, paraoxonase-1 activity, and HDL subfraction composition and antioxidative capacity. Lipid transfer to HDL was assayed in vitro using a labeled nanoemulsion as the lipid donor. At baseline, the MS group had greater triglyceride levels and a lower HDL cholesterol concentration and lower paraoxonase-1 activity than did the controls. Training decreased the plasma triglycerides but did not change the low-density lipoprotein or HDL cholesterol levels. Nonetheless, exercise training increased the HDL subfractions’ antioxidative capacity and paraoxonase-1 activity. After training, the MS group had compositional changes in the smallest HDL subfractions associated with increased free cholesterol and cholesterol ester transfers to HDL, reaching normal values. In conclusion, the present investigation has added relevant information about the dissociation between the quantitative and qualitative aspects of HDL after short-term exercise training without any specific diet in those with the MS, highlighting the importance of evaluating the functional aspects of the lipoproteins, in addition to their plasma levels.
We investigated the changes in high-density lipoprotein (HDL) cholesterol plasma levels and in the HDL functional characteristics induced by short-term exercise training in patients with the metabolic syndrome (MS).
Specifically, we investigated the changes in HDL composition, antioxidative capacity, and ability to accept lipids. To focus on exercise effects and thus avoid interference from other variables such as dietary modifications, the participants did not receive any specific nutritional counseling.
Methods
The ethics committee of the University of São Paulo Medical School approved the present investigation, and all participants provided written informed consent. The study cohort included 30 subjects (10 healthy controls and 20 with the MS) selected from the outpatient clinic of the Heart Institute, Hospital das Clínicas of the University of São Paulo Medical School. Their age range was 21 to 70 years, and they were nonsmokers and abstainers or only moderate alcohol consumers (<25 g/day). None had cardiovascular, renal, liver, gastrointestinal, pulmonary, endocrine, or oncologic diseases. None was receiving antioxidant vitamin supplementation or drugs known to affect lipoprotein metabolism.
The presence of the MS was defined according to the American Heart Association/National Heart, Lung, and Blood Institute scientific statement. The criteria for the clinical diagnosis of the MS was the presence of ≥3 of the following characteristics: elevated waist circumference (≥102 cm in men and ≥88 cm in women), elevated triglycerides (TG) (≥150 mg/dl) or receiving drug treatment for elevated TG, reduced HDL cholesterol (≤40 mg/dl in men, ≤50 mg/dl in women) or receiving medication for reduced HDL cholesterol, elevated blood pressure (≥130 mm Hg systolic blood pressure or ≥85 mm Hg diastolic blood pressure) or receiving antihypertensive drug treatment with a history of hypertension, elevated fasting glucose (≥100 mg/dl) or receiving medication for elevated glucose.
Patients with the MS underwent exercise training for 3 months. A bicycle cardiopulmonary exercise test was performed by healthy controls at baseline and by the MS group at baseline and after the 3-month exercise period. The peak oxygen uptake was considered at the end of the test (ramp protocol with 10- to 15-W increments every minute until exhaustion) when the subject no longer maintained the bicycle velocity at 60 rpm. The heart rates corresponding to the anaerobic threshold and the respiratory compensation point were determined. The training program consisted of a 3-times/week supervised and controlled training load (45 min/day) on a bicycle ergometer, as previously described. The exercise was considered of moderate intensity and compared to brisk walking. The participants began their training at the heart rate corresponding to the anaerobic threshold. The exercise was then progressively intensified, according to each patient’s subjective effort perception, up to the heart rate corresponding to 10% less than the respiratory compensation point.
Blood samples were obtained after the patient had fasted for ≥12 hours, always at the same point of the menstrual cycle in the women, and ≥16 hours after the last exercise session. The total cholesterol (TC), HDL cholesterol, TG, and plasma glucose levels were measured using commercially available kits and standardized techniques. Low-density lipoprotein (LDL) cholesterol was calculated using the Friedewald formula.
Apolipoprotein A-I was determined using a commercial kit (Wako Pure Chemical, Osaka, Japan), and the serum samples were assayed for paraoxonase-1 activity using paraoxon as a substrate, as previously described.
The preparation of a lipidic nanoemulsion labeled with 14 C-phospholipid, 3 H-triglycerides, 14 C-cholesterol, or 3 H-cholesteryl ester (all from Amersham International, Amersham, United Kingdom) was performed, as previously described. The incubation of the radioactive nanoemulsion with the patient’s whole plasma, the chemical precipitation of the apolipoprotein B-containing lipoproteins and the nanoemulsion, and the measurements of the radioactivity transferred from the nanoemulsion to the supernatant containing the HDL fraction were performed as previously described. Venous blood collected in tubes containing ethylenediaminetetraacetic acid was kept on ice and immediately centrifuged at 4°C. The plasma was then mixed with sucrose (final concentration 0.6% wt/vol) as a cryoprotectant for lipoproteins and frozen at −80°C in plastic cryovials.
LDL and HDL were fractionated using 2 different methods. LDL was isolated using an adaptation of the single vertical spin discontinuous density gradient ultracentrifugation method. In brief, the plasma density was adjusted to 1.400 kg/L by the addition of solid potassium bromide. This solution was then overlaid with saline and ultracentrifuged for 120 minutes at 70,000 rpm at 4°C. The LDL was then removed by aspiration of the orange band from the top of the tube. HDL was fractionated using single spin isopycnic density gradient ultracentrifugation. Five major HDL subfractions were obtained: large, light HDL2b and HDL2a and small, dense HDL3a, HDL3b, and HDL3c. The lipoproteins were stored at 4°C and used within 10 days. Before being used in the experiments, ethylenediaminetetraacetic acid and potassium bromide were removed from the LDL and HDL solutions by exhaustive dialysis with phosphate-buffered saline and Chelex 100 (Bio-Rad Laboratories, California) for 24 hours at 4°C, with the buffer changed 3 times.
The content of protein, TC, and TG in the HDL subfractions was analyzed using commercially available kits and standardized techniques. Free cholesterol and phospholipids were determined using commercial kits (Wako Pure Chemical, Osaka, Japan), and the cholesterol ester content was calculated by the difference between the TC and free cholesterol.
The oxidizability of a reference LDL, retrieved from a control subject and used throughout the study, was analyzed by the in vitro resistance to oxidation with copper sulfate, using standardized methods. The antioxidative activities of the HDL subfractions were evaluated by the influence of the HDL subfractions on the resistance of the reference LDL to oxidation with copper sulfate, slightly modified, as described previously. In brief, the reference LDL (100 μg of protein/ml) was incubated at 37°C in phosphate-buffered saline in the presence of copper sulfate (5 μm) for 4 hours. The accumulation of conjugated dienes was continuously measured as the increment in absorbance at 234 nm. Two characteristic phases were identified: the lag phase and the propagation phase. Individual HDL subfractions (80 μg of protein/ml) were added to the LDL directly before oxidation. We used the HDL2a and HDL3b subfractions as representatives of HDL2 and HDL3. HDL2a was obtained from the patients with the MS before and after the exercise training, was co-incubated with the reference LDL, and the resistance to oxidation with copper sulfate was evaluated. The percentage of variation in the lag phase time for oxidation (after vs before training) was determined. The same procedure was performed with HDL3b.
Statistical analyses were performed using the Statistical Package for Social Sciences for Windows, version 10 (SPSS, Chicago, Illinois). All values are expressed as the mean ± SD, unless otherwise indicated. An α probability of p <0.05 was required for statistical significance. Between-group differences in the continuous variables were analyzed using the Student t test or Wilcoxon nonparametric test for dependent samples, as applicable. The chi-square test was used to compare the proportions between the controls and the MS group.
Results
Compared to the controls, the MS group had a greater body mass index (32.4 ± 3.3 vs 24.3 ± 2.6 kg/m 2 , p <0.05), waist circumference (110 ± 10 vs 88 ± 7 cm, p <0.05), blood pressure (141 ± 10/92 ± 4 vs 120 ± 6/75 ± 5 mm Hg, p <0.05), TC (203 ± 20 vs 192 ± 25 mg/dl, p <0.05), LDL cholesterol (123 ± 21 vs 116 ± 22 mg/dl, p <0.05), and plasma glucose (112 ± 19 vs 81 ± 6 mg/dl, p <0.05).
The effectiveness of exercise training in the MS group was demonstrated by an approximately 20% increase in the peak oxygen uptake on the cardiopulmonary test (from 19.9 ± 4.3 to 23.7 ± 4.6 ml/kg min, p <0.05). Exercise did not affect the body mass index (32.4 ± 3.3 vs 32.4 ± 3.2 kg/m 2 before and after training, respectively, p >0.05) but did reduce the waist circumference (from 110 ± 10 to 107 ± 9 cm, p <0.05) and blood pressure (from 141 ± 10/92 ± 4 to 135 ± 7/89 ± 5 mm Hg, p <0.05).
Exercise training did not significantly change the HDL cholesterol ( Table 1 ), TC (203 ± 20 vs 196 ± 27 mg/dl before and after training, respectively, p >0.05), LDL cholesterol (123 ± 21 vs 122 ± 27 mg/dl before and after training, respectively, p >0.05), or apolipoprotein A-I (1.43 ± 0.25 vs 1.45 ± 0.21 g/L before and after training, respectively, p >0.05) plasma levels. However, a significant increase occurred in the LDL resistance to oxidation with the addition of HDL2a or HDL3b subfraction from the MS group after training compared to the addition of the respective HDL2a or HDL3b subfraction before training ( Figure 1 ).
Pt. No. | Gender | Age (years) | Lipid Concentration (mg/dl) | Transfer of Labeled Lipids to HDL (%) | ||||||||||
---|---|---|---|---|---|---|---|---|---|---|---|---|---|---|
HDL Cholesterol | TG | 14 C-PL | 3 H-TG | 14 C-C | 3 H-CE | |||||||||
Before | After | Before | After | Before | After | Before | After | Before | After | Before | After | |||
MS | ||||||||||||||
1 | Female | 45 | 40 | 39 | 157 | 154 | 21.4 | 27.2 | 4.3 | 3 | 10 | 9.3 | 4.1 | 3.9 |
2 | Female | 58 | 41 | 41 | 193 | 208 | 21.5 | 25.9 | 3.4 | 3.1 | 8.1 | 8.6 | 3.4 | 4 |
3 | Female | 29 | 34 | 36 | 230 | 210 | 22.8 | 26.7 | 4.4 | 3.4 | 8.8 | 8.4 | 4.1 | 5.4 |
4 | Female | 49 | 41 | 44 | 177 | 179 | 28.2 | 25.3 | 3.7 | 2.8 | 7 | 7.7 | 2.4 | 3.6 |
5 | Female | 61 | 40 | 39 | 282 | 234 | 30.3 | 26.1 | 3.4 | 3.3 | 8.1 | 8.6 | 3.3 | 4.1 |
6 | Female | 54 | 40 | 43 | 326 | 111 | 27.9 | 27.1 | 4.8 | 2.7 | 8.1 | 7.8 | 2.5 | 4 |
7 | Female | 49 | 49 | 53 | 162 | 91 | 25.2 | 26.4 | 3.1 | 2.7 | 8 | 8.5 | 2.4 | 4.2 |
8 | Female | 42 | 39 | 34 | 230 | 213 | 25.4 | 25.7 | 3.2 | 4.3 | 7.8 | 8.3 | 2.3 | 3.3 |
9 | Female | 57 | 34 | 30 | 370 | 330 | 29.4 | 26.2 | 3 | 5.4 | 7.5 | 7.6 | 2.1 | 3.4 |
10 | Female | 56 | 40 | 36 | 276 | 191 | 27.4 | 25.6 | 3.2 | 4.5 | 8.7 | 8.9 | 2.9 | 2.8 |
11 | Male | 69 | 34 | 37 | 155 | 95 | 25 | 25.3 | 3.8 | 6.1 | 8.6 | 10.1 | 6.9 | 3.1 |
12 | Male | 47 | 31 | 34 | 159 | 156 | 32.2 | 24.7 | 4.6 | 5.5 | 8.5 | 8.9 | 3.3 | 3.7 |
13 | Male | 57 | 26 | 27 | 273 | 270 | 26 | 23.2 | 4.4 | 5.1 | 6.4 | 7.1 | 4.1 | 4.7 |
14 | Male | 40 | 35 | 27 | 166 | 104 | 30.3 | 24.9 | 3.4 | 3.3 | 7 | 8.3 | 3.3 | 4.1 |
15 | Male | 56 | 32 | 43 | 164 | 98 | 28.2 | 24.8 | 3.7 | 2.8 | 7.4 | 7.7 | 2.4 | 3.5 |
16 | Male | 50 | 36 | 37 | 265 | 200 | 26 | 23.2 | 4.4 | 5.1 | 6.1 | 7.1 | 4.1 | 4.2 |
17 | Male | 52 | 31 | 31 | 233 | 301 | 25.4 | 25.7 | 3.2 | 4.3 | 7.8 | 8.3 | 2.3 | 3.3 |
18 | Male | 47 | 36 | 44 | 195 | 206 | 32.2 | 25.8 | 4.6 | 5.5 | 7.7 | 8.6 | 3.3 | 3.7 |
19 | Male | 31 | 33 | 34 | 139 | 129 | 23.7 | 23.7 | 3.3 | 5.2 | 8.6 | 7.5 | 2.1 | 3.4 |
20 | Male | 55 | 37 | 40 | 195 | 192 | 30.2 | 26.5 | 4.4 | 2.9 | 6.8 | 9.1 | 4.4 | 4.5 |
Mean ± SD | 50 ± 10 ⁎ | 36 ± 5 † | 37 ± 6 † | 217 ± 64 † | 184 ± 68 † ‡ | 26.9 ± 3.2 ⁎ | 25.5 ± 1.2 ⁎ | 3.8 ± 0.6 † | 4.1 ± 1.2 † | 7.9 ± 0.9 † | 8.3 ± 0.8 ⁎ ‡ | 3.3 ± 1.1 † | 3.8 ± 0.6 ⁎ ‡ | |
Controls | ||||||||||||||
1 | Female | 41 | 51 | 73 | 31.1 | 6.4 | 7 | 4.8 | ||||||
2 | Female | 43 | 60 | 114 | 27 | 6.6 | 9.3 | 4.1 | ||||||
3 | Female | 37 | 56 | 75 | 24.6 | 4.8 | 7 | 3.6 | ||||||
4 | Female | 43 | 47 | 146 | 28 | 6.1 | 9.8 | 5 | ||||||
5 | Male | 46 | 57 | 150 | 27.4 | 8.3 | 10.3 | 3.4 | ||||||
6 | Male | 46 | 48 | 148 | 28.6 | 6.4 | 9.4 | 3.2 | ||||||
7 | Male | 63 | 43 | 146 | 23.9 | 6.1 | 9.3 | 4.6 | ||||||
8 | Male | 42 | 44 | 142 | 24.2 | 7.1 | 10.5 | 5.3 | ||||||
9 | Male | 44 | 69 | 128 | 27.1 | 8.5 | 9.7 | 5.5 | ||||||
10 | Male | 45 | 47 | 76 | 25.4 | 6.9 | 6.9 | 4.1 | ||||||
Mean ± SD | 45 ± 7 | 52 ± 8 | 120 ± 33 | 26.7 ± 2.3 | 6.7 ± 1.1 | 8.9 ± 1.4 | 4.4 ± 0.8 |
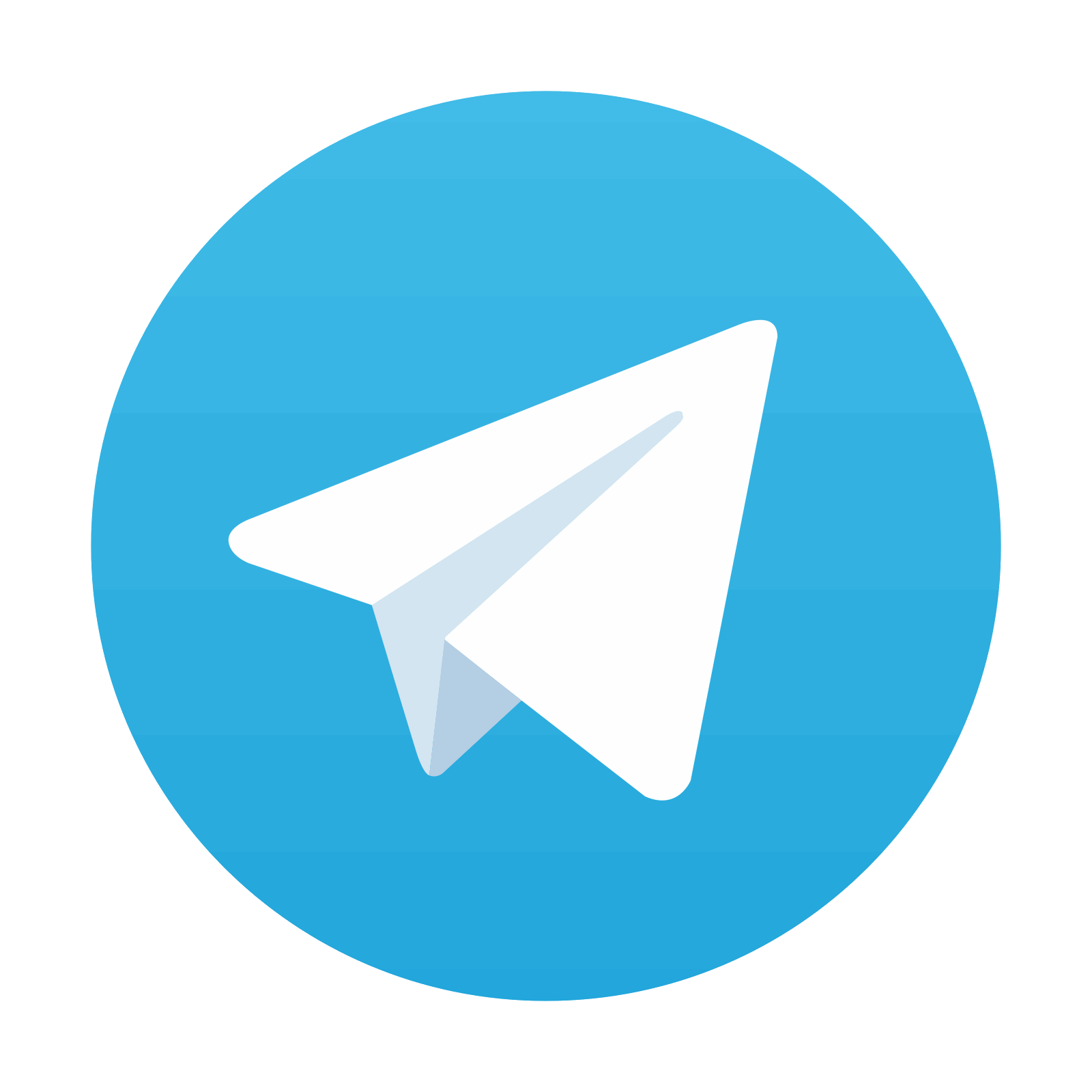
Stay updated, free articles. Join our Telegram channel

Full access? Get Clinical Tree
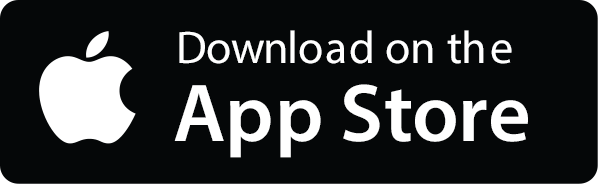
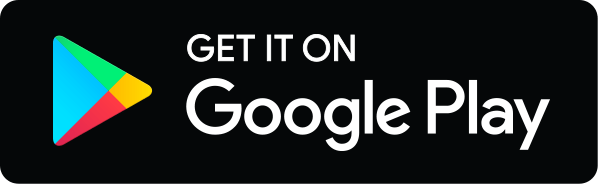
