Increased chemosensitivity to hypoxia and hypercapnia, together with a prolonged circulatory time, are the main determinants of Cheyne-Stokes (C-S) respiration in heart failure. To evaluate the effect of acetazolamide, a carbonic anhydrase inhibitor, on chemosensitivity and respiratory dynamics in patients with heart failure with C-S respiration, 12 patients (mean age 62 ± 9 years, mean left ventricular ejection fraction 24 ± 9%) and C-S respiration (mean apnea-hypopnea index 23 ± 13) who underwent 4 consecutive days of oral acetazolamide treatment (250 mg twice daily) were enrolled in this study. Assessment of chemosensitivity to hypoxia and hypercapnia, cardiopulmonary stress testing, 24-hour cardiorespiratory polygraphy, and neurohormonal characterization were performed at baseline and at the end of treatment. Acetazolamide improved central apneas (apnea-hypopnea index 23 ± 13 to 15 ± 9, p = 0.012) and the percentage of time spent below an arterial oxyhemoglobin saturation of 90% (16 ± 23% to 10 ± 18%, p = 0.005). Chemosensitivity to hypoxia was blunted (1.03 ± 0.69 to 0.78 ± 0.55 L/min/mm Hg, p = 0.032), while chemosensitivity to hypercapnia increased after acetazolamide (1.27 ± 0.71 to 1.54 ± 0.78 L/min/% arterial oxygen saturation, p = 0.023); patients achieved a lower workload (90 ± 30 to 81 ± 30 W, p <0.001), with no differences in peak oxygen consumption, while there was an increment in the regression slope relating minute ventilation to carbon dioxide output (39 ± 10 to 43 ± 9, p = 0.010). In conclusion, in patients with heart failure, acetazolamide diminishes C-S respiration and improves oxyhemoglobin saturation, likely by decreasing chemosensitivity to hypoxia. However, it is associated with reduced maximal workload achieved during effort and increased chemosensitivity to hypercapnia, inducing a reduction in the ventilatory efficiency.
Some studies have reported a beneficial effect of acetazolamide, a carbonic anhydrase inhibitor, in the treatment of central sleep apnea at high altitude and in idiopathic central sleep apnea. However, only 1 study has demonstrated acetazolamide to be effective also in patients with heart failure (HF) with Cheyne-Stokes (C-S) respiration, although the exact mechanisms through which this occurred remain unclear. Increased chemosensitivity to hypercapnia and hypoxia contributes to trigger the onset and perpetuate C-S respiration in patients with chronic HF. Furthermore, chemosensitivity is thought to exert a direct and independent action on the adrenergic drive, leading to ventricular arrhythmias, ventilatory inefficiency during exercise, and, most important, overall worse prognosis. Studies in healthy subjects suggest that acetazolamide may directly affect chemosensitivity, increasing sensitivity to hypercapnia and decreasing sensitivity to hypoxia. We hypothesized that acetazolamide may affect chemosensitivity to hypoxia and hypercapnia in patients with HF, a hypothesis that has never been investigated in this setting, this being 1 possible mechanism for its efficacy in reducing the incidence of C-S respiration. We therefore evaluated the effect of acetazolamide on chemosensitivity to hypoxia and hypercapnia, as well as its effect on respiratory pattern at rest and during exercise.
Methods
From September 2008 to February 2010, we screened 48 ambulatory patients with HF from our clinic with left ventricular systolic dysfunction (left ventricular ejection fraction <50%) and C-S respiration on ambulatory cardiorespiratory polygraphy (apnea-hypopnea index, defined as the number of apnea or hypopnea episodes per hour of recording, >15 per hour). Exclusion criteria were New York Heart Association class IV, acute coronary syndromes within the previous 6 months, glomerular filtration rate <30 ml/min, pulmonary disease defined as vital capacity and total lung capacity <50% of predicted value, forced expiratory volume in 1 second <50% of predicted value, and forced vital capacity <70%. Patients with obstructive sleep apnea syndrome, defined as a number of obstructive events >50% of total hypopnea episodes, and current treatment with morphine or derivatives, theophylline, oxygen, benzodiazepines, or acetazolamide were also excluded. Twelve patients matched the entry criteria and were enrolled in the study. In addition to the standard therapeutic regimen, patients were treated with oral acetazolamide 250 mg twice daily for 4 days. A 4-day treatment regimen was chosen to assess the “long-term” effect of acetazolamide administration, while the double administration was chosen to study the effect of the drug on both nighttime and daytime C-S respiration.
At baseline and at the end of treatment, all patients underwent (1) evaluation of chemosensitivity; (2) blood sampling for N-terminal–pro-brain natriuretic peptide, epinephrine, norepinephrine, plasma renin activity, and aldosterone; (3) arterial blood gas analysis; (4) cardiopulmonary exercise stress testing; and (5) 24-hour ambulatory cardiorespiratory polygraphy.
The protocol was approved by the local ethics committee. Informed consent was obtained from all subjects.
Chemosensitivity was assessed using the rebreathing technique, as previously described. Chemosensitivity to hypoxia, or hypoxic ventilatory response (HVR), was calculated as the linear regression slope between minute ventilation and arterial oxygen saturation during the hypoxic-normocapnic trial. Chemosensitivity to hypercapnia, or hypercapnic ventilatory response (HCVR), was calculated as the linear regression slope between minute ventilation and end-tidal carbon dioxide during the hypercapnic-normoxic trial. As cut-off values for defining increased chemosensitivity, we considered 0.77 L/min/mm Hg for HVR and 0.79 L/min/% arterial oxygen saturation for HCVR (i.e., 2 times the standard deviation over the mean value of a control group of healthy subjects). All patients were familiar with chemosensitivity testing.
Neurohormonal assays were performed as described elsewhere. Symptom-limited cardiopulmonary exercise tests were conducted on a bicycle ergometer according to a ramp protocol (V max ; Sensormedics, Milan, Italy). Circulatory power was calculated as peak oxygen uptake (V o 2 ) multiplied by peak systolic blood pressure, and peak oxygen pulse was calculated as peak V o 2 divided by heart rate. All cardiopulmonary exercise tests were performed by the same physician, blinded to the results of the chemosensitivity tests. All patients were familiar with cardiopulmonary exercise testing. All subjects underwent a 24-hour continuous ambulatory polygraphic recording that included thoracic and abdominal respiratory inductance plethysmography, airflow (using a nasal-oral thermocouple and nasal pressure recording), finger pulse oximetry, and electrocardiography (Somte; Compumedics, Abbotsford, Australia). An episode of apnea was defined as the cessation of respiratory flow for ≥10 seconds, whereas hypopnea was defined as a reduction in respiratory flow lasting ≥10 seconds and associated with a ≥4% decrease in arterial oxyhemoglobin saturation. Apnea and hypopnea were considered as central or obstructive according to the combined analysis of ribcage and abdominal excursions and airflow signal.
Statistical analysis was performed using SPSS version 13.0 (SPSS, Inc., Chicago, Illinois). Data are expressed as mean ± SD if normally distributed or as median (interquartile range) otherwise. Neurohormones were logarithmically transformed to normalize a skewed distribution when needed. Paired Student’s t tests or McNemar’s tests were performed to compare the effect of acetazolamide with baseline status within subjects. The sample size was determined for the principal question, namely, changes in chemoreflex sensitivity after acetazolamide administration. In a pilot study with 3 patients, we found that 12 patients would be sufficient to reach 80% power to detect changes in HCVR and HVR. A p value <0.05 was considered statistically significant.
Results
The study population consisted of 12 patients with HF (11 men) receiving optimal medical therapy. The mean age was 62 ± 9 years, the mean body mass index 29 ± 4 kg/m 2 , and the mean left ventricular ejection fraction 24 ± 9%. On the whole, patients showed at baseline a moderate degree of neurohormonal activation: median N-terminal–pro-brain natriuretic peptide was 1,216 ng/L (interquartile range 863 to 1,844), median norepinephrine 509 ng/L (interquartile range 461 to 736), median plasma renin activity 2.49 ng/L/hour (interquartile range 1.71 to 4.03), and median aldosterone 264 ng/L (interquartile range 122 to 279).
At baseline, 8 patients demonstrated elevated chemosensitivity with increases in HVR and HCVR, 1 patient demonstrated an increase solely in HVR, and 3 patients had normal HVR and HCVR.
Administration of acetazolamide had different effects on chemosensitivity to hypoxia or hypercapnia ( Figures 1 and 2 ). Indeed, while chemosensitivity to hypoxia was blunted by acetazolamide by 24% (HVR 1.03 ± 0.69 to 0.78 ± 0.55 L/min/mm Hg, p = 0.03; Figure 2 ), chemosensitivity to hypercapnia increased by 21% (HCVR 1.27 ± 0.71 to 1.54 ± 0.78 L/min/% arterial oxygen saturation, p = 0.02; Figure 2 ). With respect to arterial blood gas analysis, acetazolamide resulted in a mild metabolic acidosis, with a nonsignificant trend toward a reduction of the partial arterial pressure of carbon dioxide and a significant improvement in the partial arterial pressure of oxygen and oxyhemoglobin saturation ( Table 1 ).
Patient | Age (years) | AHI (n/hour) | Diurnal AHI (n/hour) | Nocturnal AHI (n/hour) | S a O 2 <90% (%) | pH | P a O 2 (mm Hg) | P a CO 2 (mm Hg) | |||||||
---|---|---|---|---|---|---|---|---|---|---|---|---|---|---|---|
Baseline | ACZ | Baseline | ACZ | Baseline | ACZ | Baseline | ACZ | Baseline | ACZ | Baseline | ACZ | Baseline | ACZ | ||
1 | 43 | 10 | 5 | 4 | 2 | 18 | 12 | 3 | 1 | 7.46 | 7.42 | 87 | 106 | 35 | 30 |
2 | 55 | 30 | 12 | 29 | 11 | 33 | 13 | 8 | 3 | 7.50 | 7.46 | 72 | 93 | 29 | 26 |
3 | 58 | 6 | 5 | 2 | 2 | 15 | 8 | 8 | 7 | 7.46 | 7.42 | 83 | 100 | 29 | 33 |
4 | 59 | 27 | 30 | 15 | 19 | 49 | 48 | 15 | 14 | 7.40 | 7.31 | 87 | 93 | 36 | 38 |
5 | 60 | 21 | 18 | 11 | 10 | 32 | 19 | 9 | 7 | 7.46 | 7.45 | 64 | 85 | 35 | 33 |
6 | 61 | 20 | 15 | 10 | 10 | 37 | 23 | 10 | 4 | 7.46 | 7.36 | 83 | 92 | 43 | 33 |
7 | 63 | 18 | 14 | 15 | 4 | 24 | 26 | 11 | 5 | 7.49 | 7.40 | 97 | 103 | 29 | 33 |
8 | 64 | 54 | 26 | 43 | 16 | 74 | 38 | 12 | 0 | 7.45 | 7.45 | 74 | 99 | 37 | 31 |
9 | 68 | 26 | 8 | 20 | 1 | 34 | 15 | 7 | 2 | 7.44 | 7.43 | 103 | 98 | 37 | 34 |
10 | 71 | 27 | 13 | 17 | 8 | 45 | 23 | 13 | 10 | 7.44 | 7.42 | 72 | 85 | 34 | 31 |
11 | 73 | 34 | 32 | 22 | 24 | 46 | 41 | 88 | 67 | 7.47 | 7.37 | 65 | 95 | 34 | 33 |
12 | 74 | 8 | 7 | 1 | 3 | 26 | 13 | 4 | 0 | 7.43 | 7.38 | 78 | 111 | 36 | 29 |
Mean ± SD | 23 ± 13 | 15 ± 9 | 16 ± 12 | 10 ± 18 | 36 ± 16 | 23 ± 13 | 16 ± 23 | 10 ± 18 | 7.45 ± 0.03 | 7.40 ± 0.04 | 80 ± 12 | 97 ± 8 | 34 ± 4 | 32 ± 3 | |
p value | 0.012 | 0.049 | <0.001 | 0.005 | <0.001 | 0.004 | 0.153 |
All patients had central sleep apnea, but 3 patients also had from obstructive events (relative percentage of obstructive events 43%, 22%, and 2%). Acetazolamide 250 mg twice daily significantly reduced central sleep apnea ( Table 1 ). This stabilization of ventilation with acetazolamide was not confined solely to central sleep apnea at night; there was also a significant reduction in daytime C-S respiration, with a 41% reduction of diurnal apnea-hypopnea index ( Figure 3 ). The observed improvement in apnea-hypopnea index was due to a reduction in central events, because there was no decrease in the number of obstructive events in the 3 patients with episodes of obstructive sleep apnea. The reduction in central events was associated with a significant improvement in arterial oxyhemoglobin saturation, as reflected by a 36% decrease of the total time spent with a saturation <90% ( Table 1 ) and by an increase in the minimal oxygen saturation achieved during apneas (82 ± 5 to 86 ± 3, p = 0.001).
At baseline, the main limitation to exercise was dyspnea, as reported by 9 patients, whereas after acetazolamide administration, leg fatigue became the predominant limiting symptom, which was reported by 9 patients. Exertional oscillatory ventilation was demonstrated in 6 of 12 patients; its prevalence was unchanged by 4 days of treatment with acetazolamide. Exercise capacity after acetazolamide administration was reduced by 10%, with no change in peak V o 2 reached. Acetazolamide was associated with a 13% increase in the regression slope relating ventilation to carbon dioxide production (minute ventilation/carbon dioxide production slope), despite no difference in peak ventilation ( Table 2 ). Maximal heart rate and blood pressure were lower at peak exercise on acetazolamide, with a corresponding significant reduction in the rate-pressure product (all p values <0.05), while there was no difference in the circulatory power and in the peak oxygen pulse (data not shown).
Patient | Peak Work Rate (W) | Peak V o 2 (Predicted %) | Peak V o 2 /kg (ml/min/kg) | V o 2 /Work (ml/min/kg/W) | Rest Ventilation (L/min) | Peak Ventilation (L/min) | VE/V co 2 Slope | |||||||
---|---|---|---|---|---|---|---|---|---|---|---|---|---|---|
Baseline | ACZ | Baseline | ACZ | Baseline | ACZ | Baseline | ACZ | Baseline | ACZ | Baseline | ACZ | Baseline | ACZ | |
1 | 100 | 86 | 42 | 43 | 10.3 | 10.4 | 11 | 12 | 21 | 16 | 71 | 70 | 36 | 42 |
2 | 59 | 50 | 32 | 26 | 11.4 | 9.4 | 8 | 8 | 16 | 16 | 56 | 48 | 55 | 63 |
3 | 50 | 41 | 33 | 36 | 7.2 | 7.8 | 9 | 10 | 12 | 12 | 34 | 35 | 38 | 43 |
4 | 130 | 126 | 59 | 66 | 12.0 | 13.6 | 8 | 10 | 10 | 10 | 60 | 72 | 26 | 31 |
5 | 100 | 87 | 52 | 52 | 15.5 | 15.4 | 11 | 11 | 15 | 13 | 66 | 71 | 36 | 36 |
6 | 133 | 124 | 73 | 70 | 15.6 | 15.0 | 10 | 9 | 14 | 14 | 75 | 82 | 28 | 38 |
7 | 55 | 48 | 36 | 41 | 9.2 | 9.7 | 10 | 10 | 12 | 15 | 45 | 43 | 42 | 43 |
8 | 102 | 92 | 58 | 51 | 14.4 | 12.8 | 10 | 10 | 16 | 16 | 60 | 50 | 34 | 36 |
9 | 70 | 60 | 37 | 36 | 7.8 | 7.7 | 7 | 9 | 12 | 12 | 53 | 53 | 59 | 58 |
10 | 74 | 66 | 44 | 40 | 11.9 | 10.8 | 9 | 9 | 10 | 10 | 39 | 33 | 32 | 38 |
11 | 72 | 71 | 31 | 20 | 6.6 | 4.3 | 6 | 9 | 10 | 8 | 33 | 25 | 44 | 40 |
12 | 130 | 117 | 63 | 67 | 13.7 | 14.7 | 7 | 8 | 11 | 15 | 56 | 62 | 33 | 44 |
Mean ± SD | 90 ± 30 | 81 ± 30 | 47 ± 15 | 46 ± 16 | 11.3 ± 3.1 | 11.0 ± 3.4 | 8.9 ± 1.6 | 9.7 ± 1.2 | 13 ± 3 | 13 ± 3 | 54 ± 14 | 54 ± 18 | 39 ± 10 | 43 ± 9 |
p value | <0.001 | 0.375 | 0.363 | 0.029 | 0.866 | 0.928 | 0.01 |
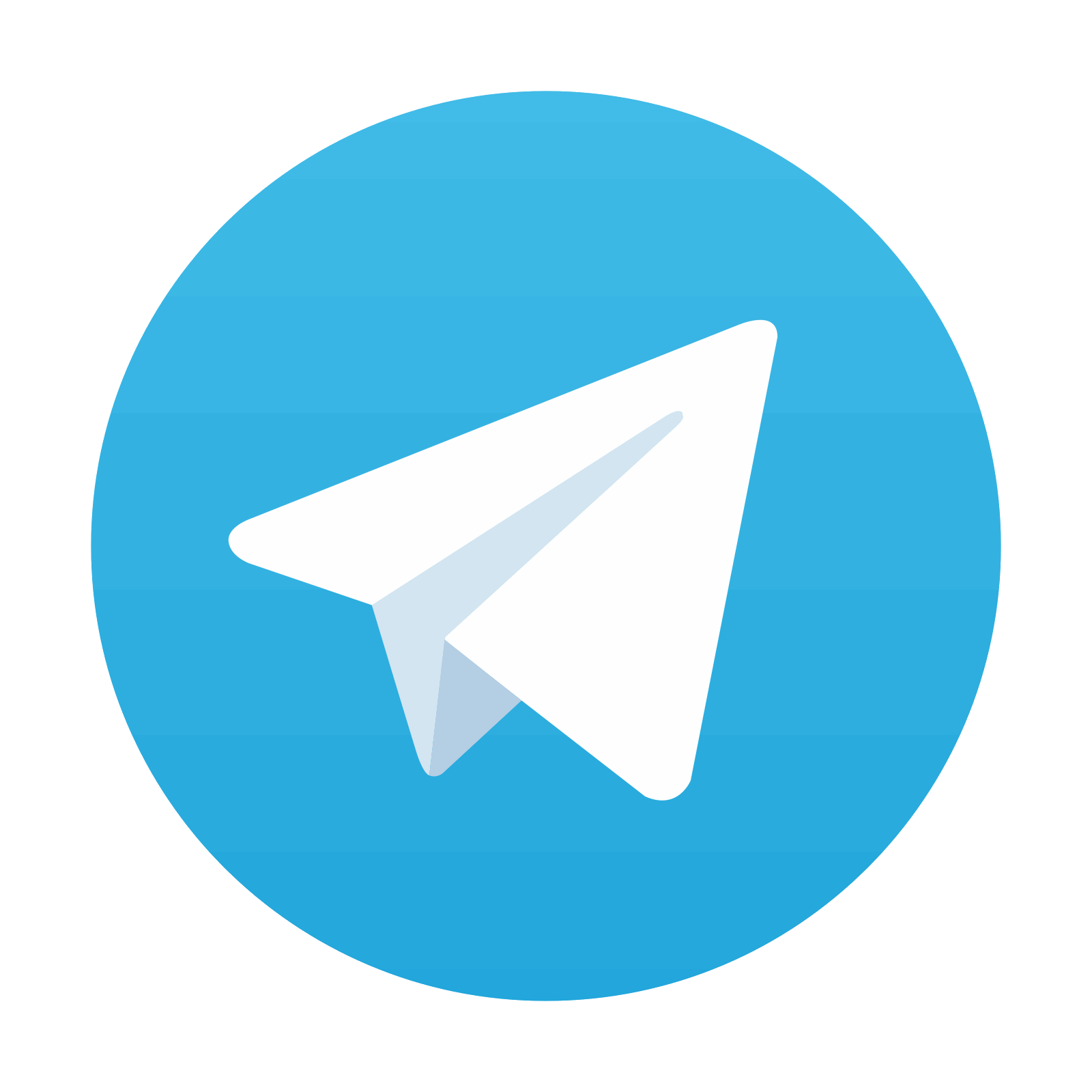
Stay updated, free articles. Join our Telegram channel

Full access? Get Clinical Tree
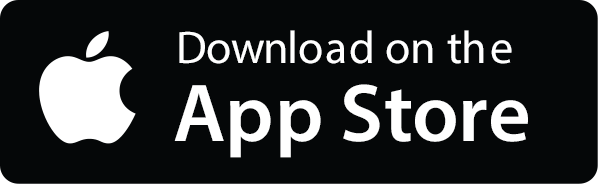
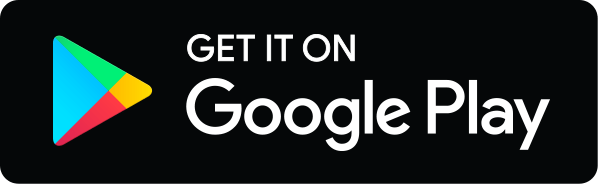
