Given the high prevalence of coronary artery disease (CAD), the evaluation of patients with suspected or documented ischemic heart disease is one of the most common indications for echocardiography. History taking and physical examination in a patient suspected of having CAD remain central to the diagnostic process. Echocardiography, as a rapid, noninvasive, ambulatory, and inexpensive modality, has a unique role, however, in diagnosing CAD, assessing the extent and severity of the disease, and providing important prognostic information that can decisively affect the therapeutic approach. The echocardiographic evaluation focuses on the functional outcome of CAD, global and segmental wall function, and complications of myocardial infarction (MI). This chapter focuses on the use of echocardiography for the diagnosis and management of suspected or proven acute coronary syndrome (ACS).
Sequence of Events in Myocardial Ischemia
Ischemia results from an abnormal myocardial oxygen supply-to-demand ratio. Blood flow is usually adequate for myocardial oxygen demands at rest, unless there is critical (>90% diameter stenosis) coronary artery narrowing. Imbalance occurs in the presence of a physiologically significant stenosis when oxygen demand is increased, as with exercise, mental stress, or pharmacologic interventions, or when myocardial perfusion is reduced by subtotal or total coronary occlusion secondary to atherothrombosis. The first abnormalities to take place ( Fig. 14-1 ) when coronary blood flow is insufficient to meet myocardial demand are cellular biochemical changes, followed by a perfusion defect, diastolic dysfunction (characterized by abnormalities of ventricular relaxation or compliance) and, shortly afterward, impairment of regional systolic wall thickening and motion. The ischemic electrocardiogram (ECG) changes and clinical symptoms of angina (if they appear) are late manifestations of ischemia. Given this sequence of events, echocardiography represents a unique and sensitive tool for early detection of myocardial ischemia, particularly by its ability to identify regional wall motion abnormalities.

Evaluation of Systolic Function in Acute Coronary Syndromes
Qualitative and Semiquantitative Evaluation of Regional and Global Systolic Function
Global and regional ventricular function can be evaluated with echocardiography. Global systolic function can be qualitatively classified as normal or mildly, moderately, or severely reduced, but this information is considered incomplete by most clinicians. With ventricular contraction, a target point chosen along the endocardial surface moves inward, toward the center of the ventricle (endocardial excursion); the cavity area decreases (area shrinkage), and the distance between the endocardial and epicardial interfaces increases (wall thickening). A few seconds after coronary occlusion, a decrease in the amplitude of endocardial excursion and wall thickening becomes apparent in the area supplied by the obstructed artery. The abnormality is defined as hypokinesis when contraction is normally directed but reduced in magnitude, akinesis when it is absent, or dyskinesis when there is systolic bulging.
Semiquantitative assessment of regional left ventricular contraction is provided by the wall motion score index (WMSI). The left ventricle is divided into 16 or 17 (for perfusion studies) segments ( Fig. 14-2 ), as suggested by the American Society of Echocardiography. A score is assigned to each segment according to its contractility, as follows: normal or hyperkinesis = 1, hypokinesis = 2, akinesis (negligible thickening) = 3, dyskinesis (paradoxic systolic motion) = 4, and aneurysmal (diastolic deformation) = 5. There is no specific score for compensatory hyperkinesis. The WMSI is equal to the sum of the regional scores divided by the number of evaluable segments and can vary between 1 (for normal ventricular contraction) and 3.9 (for severe systolic dysfunction; Fig. 14-3 ). A WMSI of 1.7 usually suggests dysfunction involving more than 20% of the left ventricle after acute myocardial infarction (AMI). The prognostic value of the left ventricular (LV) WMSI has been shown in clinical studies. Among a group of patients admitted with AMI, patients with favorable indices (best quintile) had an incidence of cardiovascular death of 8% at 1 year, whereas patients with the worst quintile had 51% mortality at 1 year. The increase in the odds ratio for in-hospital complications (e.g., death, arrhythmias, heart failure) was 1.20 for each 0.2-unit increase in the WMSI (95% confidence interval [CI], 1.10 to 1.27; P < .001). Similar results have been published by Kan and colleagues with a significantly higher mortality rate in the group with the most abnormal score compared with patients with favorable ones (61% versus 3%). In addition, another study in post-MI patients has shown a good correlation between the echocardiographic WMSI and the left ventricular ejection fraction measured by radionuclide ventriculography.


Because CAD causes segmental dysfunction, which can be accompanied by compensatory hyperkinesis of nonischemic segments, regional assessment of systolic function is more sensitive for the detection of ischemia than global approaches. Nevertheless, determination of the left ventricular ejection fraction (LVEF) is part of a standard examination. The correlation between the visual echocardiographic estimation and radionuclide determination is good, especially in patients with an impaired ejection fraction. The eyeball method requires experience, however, and clinicians should validate their own performance with quantitative methods. All echocardiographic approaches for the assessment of regional and global ventricular function also need to take into account the quality of endocardial border definition, asynchronous contraction patterns observed with pacemakers or conduction defects, abnormal postoperative septal motion, and heterogeneity of normal ventricular function.
Tei has described a Doppler-derived index combining systolic and diastolic time intervals called the myocardial performance index (MPI), or Tei index. This index is defined as the sum of isovolumic contraction and relaxation time divided by the ejection time ( Fig. 14-4 ). In a multivariate analysis, Poulsen and associates have found that an MPI of 0.60 in post-AMI patients was an independent predictor of heart failure or cardiac death. Similar findings and MPI cutoff values have been reported in AMI patients by others.

The M-mode mitral annulus motion toward the apex also can be used as an indirect measure of left ventricular systolic function. It is usually measured in the apical four-chamber view, where the movement of the mitral annulus is parallel to the ultrasound beam and is normally 12 ± 2 mm. A motion of less than 8 mm has been reported to have a sensitivity of 98% and a specificity of 82% for the identification of an ejection fraction less than 50%. Pai and coworkers have examined the mitral annular systolic excursion in 57 patients with a wide range of LVEFs (13% to 84%) and found a good correlation ( r = 0.95; P < 0.001) with the ejection fraction measured by a radionuclide approach. This method is seldom currently used, but may be helpful in patients with poor echogenicity. A modern version of this method relies on Doppler tissue imaging (DTI) of the mitral annulus and is discussed later in this chapter.
Quantitative Evaluation of Global Left Ventricular Systolic Function
Quantitative evaluation of global left ventricular systolic function is based on endocardial border tracing, with or without epicardial border tracing, at end-diastole and end-systole in one or preferably several views. Assessment is based on analysis of wall motion (endocardial excursion) or wall thickening (interface separation). Evaluation of regional wall thickening is not influenced by cardiac translation or rotation, as opposed to wall motion, but requires excellent definition of the endocardial and epicardial borders, which constitutes its major limitation.
Quantitative assessment of global systolic function requires the determination of the LV cavity dimensions. Volume estimations are based on geometric assumptions about ventricular shape, which range from a simple ellipsoid to a complex hemicylindrical, hemiellipsoid shape. Descriptions of each geometric shape and the corresponding formula and requirements are beyond the scope of this chapter. The American Society of Echocardiography (ASE) recommendations for chamber quantification favors the modified Simpson’s biplane method for LV volumes and ejection fraction. The Simpson biplane method involves tracing of the endocardial borders in the apical four-chamber and two-chamber views, at end-systole and end-diastole. Determination of the LV end-diastolic volume (EDV) and end-systolic volume (ESV) allows calculation of the stroke volume (EDV − ESV = SV), cardiac output (SV × heart rate), and ejection fraction ([SV/EDV] × 100).
Validation studies suggest that real-time three-dimensional echocardiography (3DE) is superior to standard two-dimensional echocardiography (2DE) in the determination of LV and right ventricular (RV) volumes and mass, using cardiac magnetic resonance imaging (MRI) as the gold standard. In a study comparing 55 AMI patients with LVEF < 40%, 3DE-derived EDV, ESV, and ejection fraction (EF) had better correlation with MRI ( r = 0.96; 95% CI, 0.93 to 0.97) than 2DE ( r = 0.88; 95% CI, 0.84 to 0.92).
Quantitative Evaluation of Regional Left Ventricular Systolic Function and Assessment of Myocardial Ischemia
Notable advances in recent years have been done in the field of quantitative evaluation of regional wall motion abnormalities. Tethering creates a movement distortion that renders qualitative or eyeball evaluation of regional LV dysfunction somewhat inaccurate. Reproducibility between physicians and different echocardiographic laboratories is not high in less experienced hands. A myriad of new technologies are being developed and it is beyond the scope of this chapter to consider all emerging methods. Here we will discuss the most commonly used approaches in clinical echocardiography laboratories.
Doppler Tissue, Strain, and Strain Rate Imaging
Doppler Tissue Imaging
Myocardial DTI uses the pulsed Doppler method (modified to record the low-velocity, high-amplitude signals from tissue) to measure the velocity and timing of myocardial motion. Because Doppler signals are angle-dependent, the apical views are usually chosen. Different velocity profiles are obtained, called peak systolic (S 1 m, S 2 m), peak diastolic rapid filling (Em), and atrial contraction (Am). Although reasonably well correlated with global LV function, these measures are limited by preload and afterload dependence, and are sensitive to inotropic stimulation and ischemia. Furthermore, complete characterization of myocardial motion requires correction for radial, circumferential, and longitudinal movements, which is not currently being performed in clinical practice. Mitral annular sites are easy to identify, even in patients with poor imaging quality, so assessment of long-axis systolic function by DTI may enable estimation of the LVEF in these patients. Yuda and colleagues have measured the peak systolic velocity of the septal, lateral, anterior, and inferior sites of the mitral annulus in patients with poor echogenicity. An averaged S m < 7.1 cm/s in these patients has a sensitivity and specificity of 64% and 89%, respectively, for LVEF < 50%.
Strain and Strain Rate Imaging (SRI)
The strain rate (SR) is defined as the difference in myocardial velocity between two axial points (usually 12 mm apart) along the path of the echocardiographic beam per unit time as measured by DTI; it reflects the speed of myocardial deformation. Strain (ϵ), is the integral of strain rate over time. Two equations may be used to calculate strain and strain rate, as follows:
ε = Δ L / Δ L 0
SR = ( Δ L / Δ L 0 ) / Δ t = [ v ( I 0 ) − v ( Δ L + I 0 ) ] / L 0
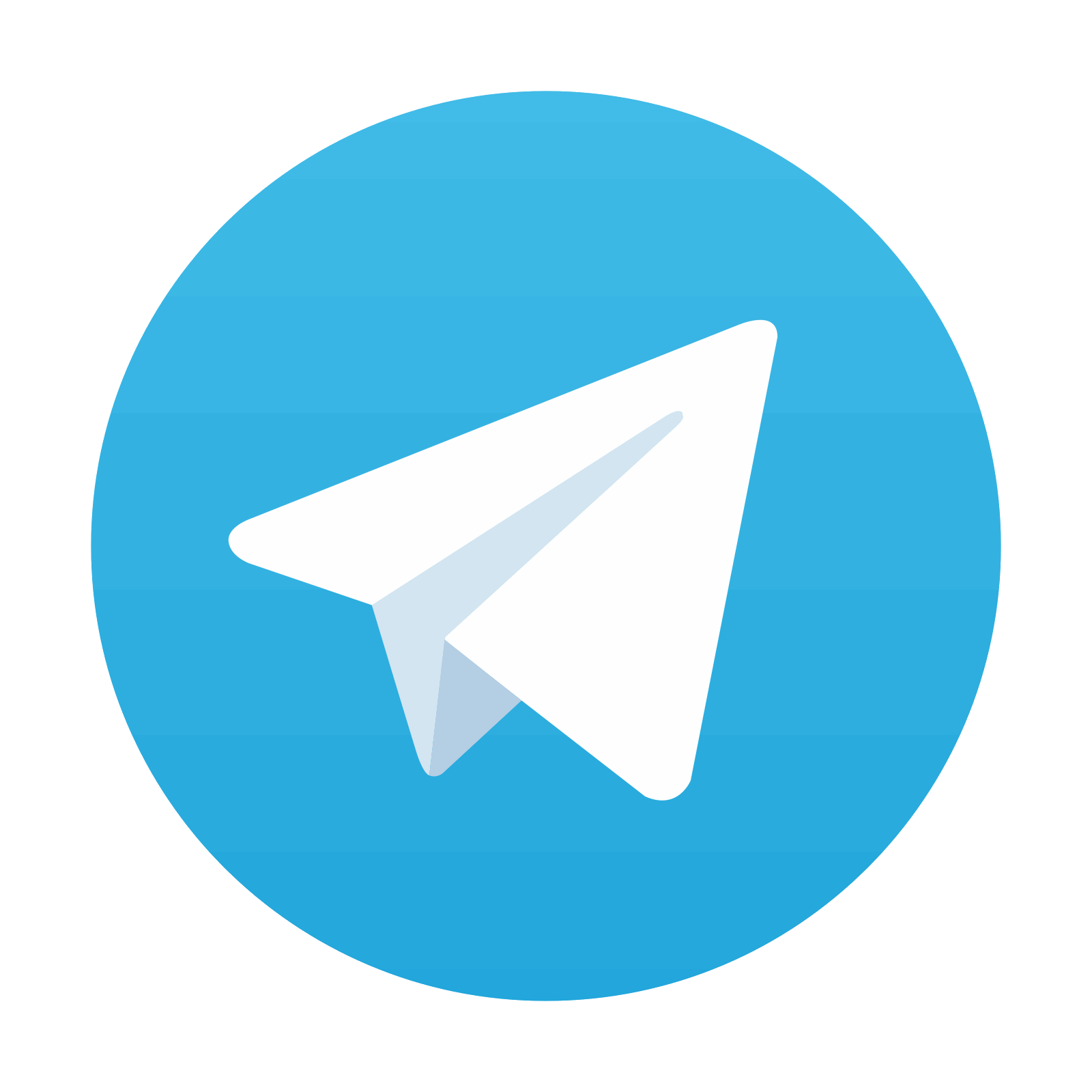
Stay updated, free articles. Join our Telegram channel

Full access? Get Clinical Tree
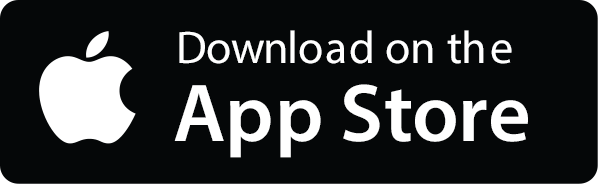
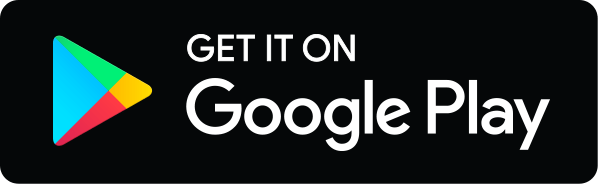