Background
Pediatric heart transplant recipients are at risk for increased left ventricular (LV) diastolic stiffness. However, the noninvasive evaluation of LV stiffness has remained elusive in this population. The objective of this study was to compare novel echocardiographic measures of LV diastolic stiffness versus gold-standard measures derived from pressure-volume loop (PVL) analysis in pediatric heart transplant recipients.
Methods
Patients undergoing left heart catheterization were prospectively enrolled. PVLs were obtained via conductance. The end-diastolic pressure-volume relationship was obtained via balloon occlusion. The stiffness constant, β, was calculated. Echocardiographic measures of diastolic function were derived from spectral and tissue Doppler and two-dimensional speckle-tracking. Ventricular volumes were measured using three-dimensional echocardiography. The novel echocardiographic estimates of ventricular stiffness included E:e′/end-diastolic volume (EDV) and E:early diastolic strain rate/EDV.
Results
Of 24 children, 18 were heart transplant recipients. Six control patients had hemodynamically insignificant patent ductus arteriosus or coronary fistula. The mean age was 9.1 ± 5.6 years. Median end-diastolic pressure was 9 mm Hg (interquartile range, 8–13 mm Hg). Lateral E:e′/EDV ( r = 0.59, P < .01), septal E:e′/EDV ( r = 0.57, P < .01), and (E:circumferential early diastolic strain rate)/EDV ( r = 0.54, P < .01) correlated with β. Lateral E:e′/EDV displayed a C statistic of 0.93 in detecting patients with abnormal LV stiffness (β > 0.015 mL −1 ). A lateral E:e′/EDV of >0.15 mL −1 had 89% sensitivity and 93% specificity in detecting an abnormal β.
Conclusions
Echocardiographic estimates of ventricular stiffness may be accurate compared with the gold standard in pediatric heart transplant recipients. The clinical usefulness of these noninvasive measures in assessing LV stiffness merits further study in children.
Highlights
- •
The objective was to validate echocardiographic measures of left ventricular stiffness.
- •
Noninvasive and invasive estimations of stiffness correlated well in children.
- •
These measures appear promising and merit further study in children.
Patients who have undergone heart transplantation are known to be at risk for increased ventricular stiffness due to prolonged ischemic time, donor-recipient size mismatch, and/or a history of rejection. Even in the presence of preserved ejection fraction, increased ventricular stiffness results in heart failure symptoms, exercise intolerance, decreased quality of life, and increased risk for mortality. However, the importance of ventricular stiffness in pediatric heart transplant recipients is unknown because of the challenges in the noninvasive assessment of diastolic function, specifically, in the noninvasive assessment of ventricular stiffness. Currently, the invasive evaluation of the end-diastolic pressure-volume relationship derived from pressure-volume loop (PVL) analysis is the reference standard method used to assess ventricular stiffness. However, PVL analysis is rarely performed for clinical purposes in children, because of the required invasive procedures and load alteration.
Current echocardiographic assessment of left ventricular (LV) diastolic function has focused on early active relaxation/diastolic suction (spectral Doppler mitral E and tissue Doppler e′ velocities, isovolumic relaxation time, etc) and the estimation of filling pressures (lateral and septal E:e′). These measures have been well validated against the reference standard in both adults and children. Although the assessment of filling pressures is an often used marker of diastolic function, it has limitations. Filling pressures are influenced by loading conditions, myocardial elastic properties, and pericardial properties. In addition, changes in ventricular stiffness often occur before an elevation in filling, making it an insensitive marker of early diastolic dysfunction. Therefore, optimally one would include a measure of ventricular stiffness in the assessment of diastolic function in addition to the more conventional assessments of early active relaxation and filling pressures.
The ability to assess for the development of increased ventricular diastolic stiffness has the potential to (1) improve clinicians’ ability to understand the natural history of the varied disease processes encountered in the pediatric heart transplantation population, (2) detect the results of therapies aimed at rejection and myocardial remodeling, and (3) improve risk stratification before interventional and surgical procedures. However, no studies have validated potential echocardiographic measures of ventricular stiffness against the gold-standard PVL measures. Therefore, the goal of this study was to assess the validity of noninvasive indices of ventricular stiffness by direct comparison with gold-standard indices derived from PVL analysis in children who have undergone heart transplantation. We hypothesized that noninvasive estimates of filling pressure divided by end-diastolic volume (EDV) would correlate well with the invasive PVL-derived ventricular stiffness constant.
Methods
Children aged 0 to 21 years undergoing clinically indicated diagnostic left heart catheterization were recruited prospectively at a single institution. We divided our cohort into two groups. The first group included children who had undergone heart transplantation. The second group included children who acted as “control subjects.” Because normal children do not undergo routine heart catheterization, these children included those with hemodynamically insignificant patent ductus arteriosus or coronary fistula (Qp:Qs < 1.5 with normal LV EDV for body size). Exclusion criteria included (1) medical status for which participation in the study presented more than minimal risk as determined by the attending physician, (2) non–sinus rhythm, (3)right-sided heart disease, and (4) rejection episode (biopsy grade ≥ 2R, pathologic antibody-mediated rejection ≥ 2, or clinical or echocardiographic changes consistent with rejection that were accompanied by an augmentation in immunosuppression) within the past 6 months, as these patients were deemed at too high risk to participate in the procedures involved in the protocol. The protocol was approved by our institutional review board. Informed consent was obtained from the parents or legal guardians of minors or from participants aged ≥ 18 years.
Study Catheterization and PVL Analysis Protocol
All patients underwent general anesthesia per the institution’s protocol. All study data were collected following the patient’s primary diagnostic and interventional procedures. A 4-Fr high-fidelity microconductance catheter (CD Leycom, Hengelo, the Netherlands) was calibrated in normal saline for 15 sec and then placed in the apex of the left ventricle via the femoral arterial approach. PVLs were volume-calibrated using hypertonic saline to account for parallel conductance. Cardiac output was determined by thermodilution. Conductance electrodes located outside the ventricle were excluded from analysis. Microconductance data were recorded at a sampling rate of 250 Hz. PVL data were recorded in triplicate over 10 sec during an expiratory breath hold. Invasive data were obtained using standard equipment approved for use in human subjects (INCA intracardiac analyzer; CD Leycom). PVL analysis was performed offline using specialized software (LabChart version 8; ADInstruments, Colorado Springs, CO).
The end-diastolic pressure-volume relationship was obtained via balloon occlusion of the venae cavae and fitted to the equation α e βEDV , where β is the chamber stiffness constant, and α is the curve fitting constant. β represents the rightward, exponential aspect of the slope of the end-diastolic pressure-volume relationship and increases as ventricular stiffness increases. Increased ventricular stiffness is considered present if β > 0.015 mL −1 in adults, but abnormal stiffness has not been defined in the pediatric population, because of the lack of data in children. In this pilot study, we used the adult cutoff value and indexed β to body surface area to account for differences in body sizes. Early active relaxation was evaluated using the isovolumic relaxation time constant (τ), calculated using the logistic method. End-diastolic pressure (EDP) was recorded. End-systolic elastance (Ees), a measure of contractility, was assessed as the slope as the end-systolic pressure-volume relationship as we have previously described in this population. Arterial elastance (Ea), a measure of afterload, was assessed as the ratio of end-systolic pressure to stroke volume. Ventriculoarterial coupling was calculated as Ea:Ees.
Echocardiographic Protocol
Echocardiograms were obtained immediately after PVL analysis under the same anesthetic conditions using a Phillips iE33 system (Philips, Amsterdam, the Netherlands). Ventricular volumes were derived from three-dimensional (3D) echocardiography (QLAB version 10.0; Philips). Three-dimensional echocardiographic volumes were stitched together after four-beat acquisition. Conventional echocardiographic measures of diastolic function included spectral Doppler E- and A-wave velocities and their respective ratio, E deceleration time, A-wave duration, tissue Doppler e′ and a′ and their respective ratios, tissue Doppler–derived isovolumic relaxation time, and pulmonary vein a-wave velocity and duration. Measurement methods conformed to recommendations by the American Society of Echocardiography and were averaged over three beats.
Two-dimensional speckle-tracking measures of deformation were obtained by tracing the endocardial border of the left ventricle using Cardiac Performance Analysis version 3.0 (TomTec Imaging Systems, Chicago, IL). Analysis was performed on Digital Imaging and Communications in Medicine images at their native frame rate. The minimum frame rate for acquisition was 60 frames/sec. The mean frame rate for the analyzed studies was 94 ± 11 frames/sec. Longitudinal early diastolic strain rate (LEDSR) was obtained by averaging six segments from the apical four-chamber view. Circumferential early diastolic strain rate (CEDSR) was obtained by averaging six segments from the parasternal short-axis view. Studies where more than two segments could not be adequately traced were excluded.
Echocardiographic Measures of Stiffness
Assessment of the end-diastolic pressure-volume relationship and calculation of the ventricular stiffness constant can only be done by acutely altering loading conditions. This is not feasible in a noninvasive evaluation. To assess stiffness by echocardiography, we assessed operating stiffness. Conceptually, operating ventricular stiffness is described as the relationship between ventricular EDP and EDV in a patient’s baseline physiologic state. If one assumes that the LV volume is 0 mL when LV pressure reaches 0 mm Hg, one can calculate operating stiffness by simply dividing EDP by EDV. As β increases, operating stiffness also increases. This is conceptually displayed in Figure 1 . We used echocardiographic surrogates of EDP divided by EDV derived from 3D echocardiography to assess ventricular stiffness. Surrogates of LV EDP included lateral E:e′, septal E:e′, E:LEDSR, and E:CEDSR. Therefore, the novel echocardiographic estimates of LV operating stiffness included lateral and septal E:e′/EDV and their average, E:LEDSR/EDV, and E:CEDSR/EDV.

Statistical Analysis
The distribution of data as parametric or nonparametric was assessed using the Shapiro-Wilk test. Differences between patient groups were assessed using independent t tests or Mann-Whitney U tests, as appropriate, for continuous variables and χ 2 tests or Fisher exact tests for categorical variables. The relationship between invasive and echocardiographic measures were assessed using Pearson correlation. Receiver operating characteristic curves were created, and the area under the curve was determined to assess the discriminatory ability of each echocardiographic variable to predict the presence of increased ventricular stiffness. All studies were reassessed for intra- and interobserver variability using intraclass correlation coefficients for absolute agreement. P values < .05 were considered to indicate statistical significance. Statistical analysis were performed using SPSS version 23 (IBM, Armonk, NY).
Results
A total of 24 patients were enrolled; 18 patients were status post orthotopic heart transplantation, five patients had hemodynamically insignificant patent ductus arteriosus, and one had a hemodynamically insignificant coronary fistula (six control patients). We have previously reported associations between PVL and echocardiographic measures of contractility in this cohort. All control patients had successful interventions before PVL and echocardiographic acquisition. A representative PVL during preload reduction and the resulting end-diastolic pressure-volume relationship is shown in Figure 2 . Intra- and interobserver variability showed intraclass correlation coefficients of r = 0.91 and r = 0.88, respectively, for lateral E:e′/EDV; r = 0.92 and r = 0.86, respectively, for septal E:e′/EDV; r = 0.80 and r = 0.72, respectively, for E:LEDSR/EDV; and r = 0.71 and r = 0.59, respectively, for E:CEDSR/EDV.
Transplantation versus Control Patients
Demographic, clinical, and catheterization data from these patients are presented in Table 1 . There were no differences in EDP between groups. However, transplantation patients did have higher LV stiffness compared with control subjects. Echocardiographic data comparing the two groups are presented in Table 2 . Transplantation patients had higher LV mass/volume Z scores, lateral E:e′/EDV, and septal E:e′/EDV compared with control subjects. There were no differences in E:LEDSR/EDV or E:CEDSR/EDV between groups. In all patients, β displayed a correlation with Ea ( r = 0.50, P = .01).
Heart transplant ( n = 18) | Control ( n = 6) | P | |
---|---|---|---|
Age (y) | 10.0 ± 5.9 | 8.4 ± 5.6 | .56 |
Female | 9 (50%) | 3 (50%) | .64 |
Height (cm) | 125 ± 33 | 138 ± 29 | .40 |
Weight (kg) | 22.6 (35.8) | 35.2 (43.3) | .54 |
BSA (m 2 ) | 1.03 ± 0.47 | 1.16 ± 0.45 | .58 |
SBP (mm Hg) | 89 ± 9 | 87 ± 9 | .75 |
DBP (mm Hg) | 46 ± 7 | 51 ± 8 | .23 |
Cardiac index (L/min/m 2 ) | 3.4 ± 1.0 | 3.7 ± 1.7 | .69 |
MvO 2 (%) | 74 ± 4 | 79 ± 7 | .09 |
Rp (Wood units) | 1.9 (0.9) | 1.1 (0.3) | .02 |
Qp:Qs | 1.0 (0) | 1.1 (0.3) | .45 |
EDP (mm Hg) | 10.9 ± 3.5 | 9.7 ± 3.0 | .45 |
Ees (mm Hg/mL) | 2.2 (3.3) | 1.0 (2.1) | .12 |
Ea (mm Hg/mL) | 2.4 ± 0.9 | 1.6 ± 0.4 | <.01 |
Ea/Ees | 1.0 (0.9) | 1.5 (1.5) | .63 |
τ (msec) | 24 (5) | 30 (13) | .16 |
β × BSA (m 2 /mL) | 0.009 (0.024) | 0.005 (0.006) | .04 |
Heart transplant ( n = 18) | Control ( n = 6) | P | |
---|---|---|---|
EDV/BSA (mL/m 2 ) | 57.3 ± 10.0 | 71.0 ± 7.3 | <.01 |
EF (%) | 58.2 (7.6) | 59.4 (6.8) | .82 |
FS (%) | 30.4 (9.8) | 31.1 (14.9) | .77 |
LV mass/volume Z score | −1.2 ± 0.9 | −2.3 ± 1.2 | .04 |
Mitral E velocity (cm/sec) | 95 ± 21 | 83 ± 29 | .32 |
Mitral A velocity (cm/sec) | 39 ± 10 | 57 ± 21 | .09 |
Mitral E:A | 2.6 ± 0.6 | 1.6 ± 0.6 | <.01 |
Mitral E deceleration time (sec) | 0.14 ± 0.04 | 0.21 ± 0.06 | .04 |
Mitral A duration (sec) | 0.11 ± 0.03 | 0.12 ± 0.01 | .59 |
Lateral e′ velocity (cm/sec) | 12.6 ± 2.7 | 11.8 ± 2.3 | .50 |
Septal e′ velocity (cm/sec) | 8.9 ± 2.4 | 9.3 ± 1.5 | .70 |
LEDSR (sec −1 ) | 1.4 ± 0.4 | 1.2 ± 0.3 | .11 |
CEDSR (sec −1 ) | 1.7 ± 0.4 | 1.4 ± 0.4 | .16 |
Lateral E:e′ | 7.9 ± 2.5 | 7.2 ± 2.0 | .56 |
Septal E:e′ | 11.4 ± 3.8 | 9.2 ± 3.1 | .20 |
E:LEDSR (cm/sec 2 ) | 47 (26) | 47 (19) | .67 |
E:CEDSR (cm/sec 2 ) | 38 (20) | 43 (14) | .52 |
Lateral E:e′/EDV/BSA (m 2 /mL) | 0.14 ± 0.04 | 0.10 ± 0.02 | .04 |
Septal E:e′/EDV/BSA (m 2 /mL) | 0.19 (0.09) | 0.11 (0.10) | .02 |
E:LEDSR/EDV/BSA (cm/sec 2 /mL/m 2 ) | 0.80 (0.43) | 0.64 (0.29) | .20 |
E:CEDSR/EDV/BSA (cm/sec 2 /mL/m 2 ) | 0.73 ± 0.18 | 0.61 ± 0.15 | .16 |
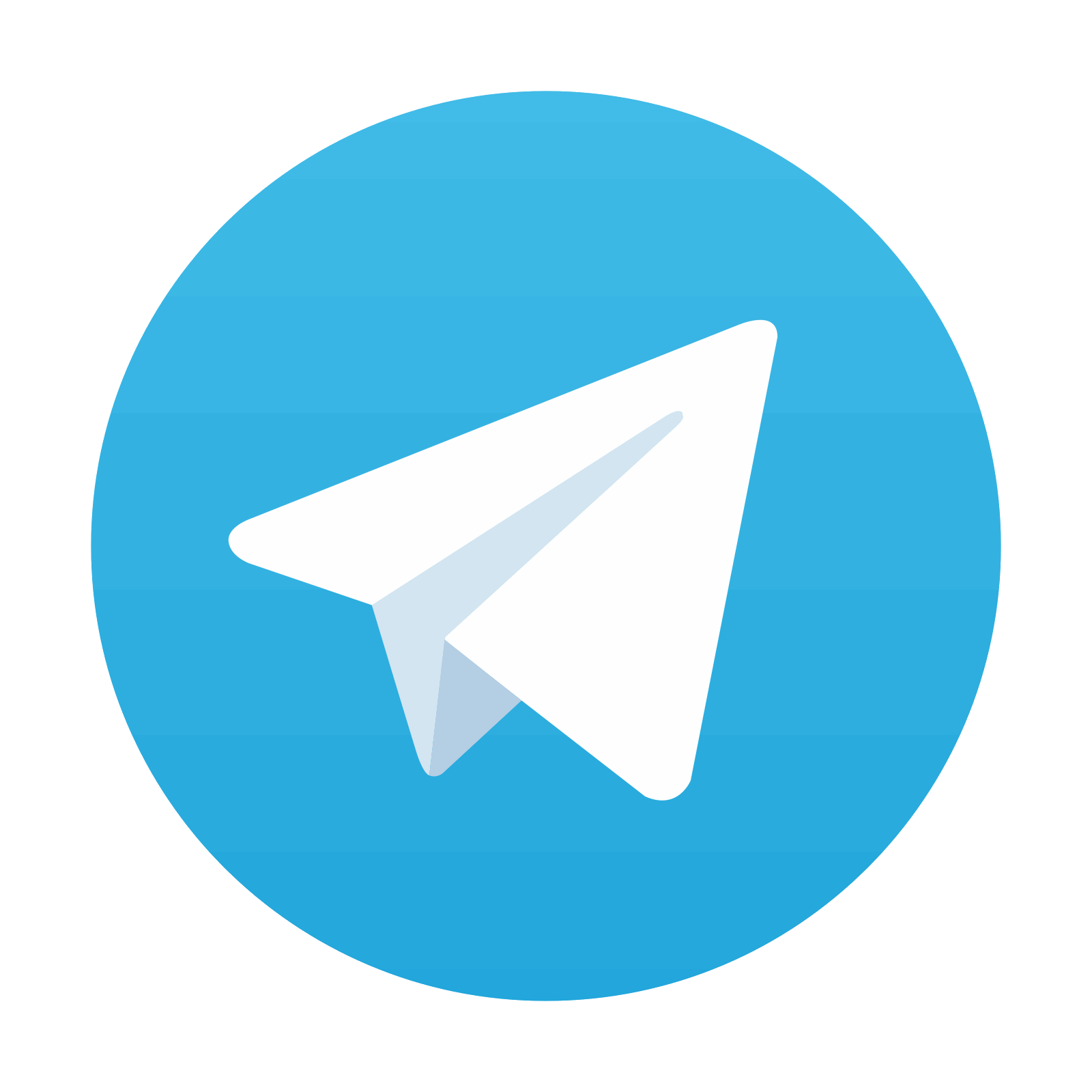
Stay updated, free articles. Join our Telegram channel

Full access? Get Clinical Tree
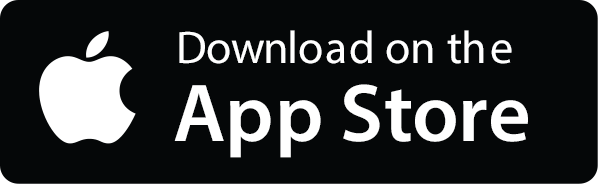
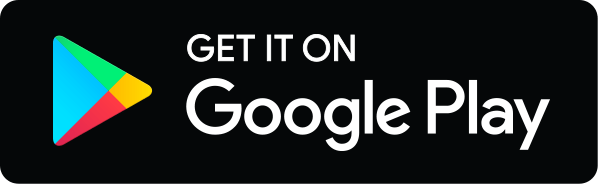
