Background
The pulsed-wave Doppler recording in the descending aorta (PWD DAO ) is one of the parameters used in grading aortic regurgitation (AR) severity. The aim of the present study was to investigate the assessment of chronic AR by PWD DAO with insights from cardiovascular magnetic resonance (CMR).
Methods
This prospective study comprised 40 patients investigated with echocardiography and CMR within 4 hours either prior to valve surgery ( n = 23) or as part of their follow-up ( n = 17) due to moderate or severe AR. End-diastolic flow velocity (EDFV) and the diastolic velocity time integral (dVTI) were measured. The appearance of diastolic forward flow (DFF) was noted. Phase-contrast flow rate curves were obtained in the DAO.
Results
Twenty-five patients had severe and eight had moderate AR by echocardiography (seven were indeterminate). The EDFV was below the recommended threshold (>20 cm/sec) in 13 patients (52%) with severe AR. Lowering the EDFV threshold (>13 cm/sec) and with a dVTI threshold >13 cm showed negative likelihood ratios of 0.27 and 0.09, respectively. Detection of DFF with PWD DAO identified a nonuniform velocity profile by CMR with positive and negative likelihood ratios of 7.0 and 0.19, respectively. The relation between EDFV and DAO regurgitant volume (DAO-RVol CMR ) was strong in patients without ( R = 0.88) and weak in patients with DFF ( R = 0.49). The DAO-RVol CMR as a percent of the total RVol CMR decreased with increasing ascending aorta (AAO) size and increased with increasing AR severity.
Conclusions
Our findings suggest that PWD DAO provides semiquantitative parameters useful to assess chronic AR severity. The limitations are related to nonuniform velocity contour and variable degree of lower body contribution, which depends on AR severity but also on the AAO size.
Highlights
- •
The ability of PW Doppler in the descending aorta in grading aortic regurgitation is controversial.
- •
We investigated the PW Doppler assessment with insights from cardiovascular magnetic resonance.
- •
We found that semiquantitative PW Doppler parameters can assess aortic regurgitation severity.
- •
The limitations are related to the nonuniform velocity contour in the descending aorta.
- •
The contribution from the lower body depends on regurgitation severity and ascending aorta size.
A comprehensive transthoracic echocardiography investigation is a cornerstone in the management of patients with valvular heart disease. Grading of aortic regurgitation (AR) severity by echocardiography is known to be difficult and is achieved by an “integrative approach” using several qualitative, semiquantitative, and quantitative parameters. There are basically three contributors to the regurgitant volume (RVol) in AR: (1) the lower body, (2) the upper body, and (3) the systolic volume expansion of the ascending aorta (AAO) and aortic arch (Windkessel effect). The contribution from the lower body has been used in echocardiography for the grading of AR severity during the past four decades based on the concept that the diastolic backward flow (DBF) in the proximal descending aorta (DAO) mirrors the RVol. Typically, the normal flow profile in the proximal DAO consists of an early diastolic flow reversal followed by mid-diastolic forward flow (DFF). In patients with chronic AR, the diastolic flow reversal is more prominent and becomes holodiastolic in moderate or severe AR. The velocity integrals in diastole (dVTI) and systole (sVTI) are surrogates for the volume passing thorough the vessel at the level of measurement. Previously, investigators used the ratio between dVTI and sVTI obtained by either continuous Doppler or pulsed-wave Doppler (PWD) for assessment of AR severity. Later, the end-diastolic flow velocity (EDFV) was introduced as a useful index in the assessment of AR severity. The good diagnostic performance of the VTI ratio and EDFV in identifying severe AR is based on studies using angiography with a four-point scale as reference. Angiography is no longer considered as the gold standard, and echocardiographic grading of regurgitation severity is performed instead using a three-point scale. The diagnostic usefulness of the PWD in the DAO (PWD DAO ) is a matter of debate. In the recent European recommendations, EDFV is considered a semiquantitative parameter, and a threshold indicating severe AR is defined (>20 cm/sec). On the other hand, the American Society of Echocardiography recommendations consider PWD DAO a qualitative parameter by emphasizing that a prominent holodiastolic flow reversal indicates severe AR. In our clinical experience, patients with severe AR can have relatively low EDFVs, and we observe that the spectral Doppler curve in diastole can have both backward and forward flow velocities, indicating complex flow. This finding questions the concept of a uniform flow profile in the DAO in patients with AR. Furthermore, dilatation of the AAO is a frequent finding in patients with AR, and it is conceivable that this might influence the contribution from the Windkessel effect and the relation between the PWD DAO findings and the RVol. In the present study we hypothesized that (1) a nonuniform velocity profile influences the relation between PWD DAO findings and AR severity and (2) the size of the AAO influences the contribution from the lower body in patients with AR. To study this we used cardiovascular magnetic resonance (CMR), since this method has unique abilities compared with angiography as it can quantify flow with high accuracy and low variability and can provide information on the spatial distribution of velocity and the complexity of flow across the vessel area. The overall aim of the present study was to investigate the assessment of chronic AR severity by PWD DAO with insights from CMR.
Methods
Study Population
This prospective study comprised 40 patients with AR who were either investigated prior to valve surgery ( n = 23) or as part of their follow-up due to moderate ( n = 14) or severe regurgitation ( n = 3). The indication for surgery was based on clinical assessment and the grading of severity by echocardiography without knowledge of the CMR findings. We did not include patients with acute AR in the study. All patients included had chronic AR, and those who underwent surgery were hemodynamically stable and did not have AR due to aortic dissection or acute endocarditis. Image acquisition, analysis, and grading of regurgitation severity were performed according to current 2017 American Society of Echocardiography guidelines. Patients with four or more specific criteria (flail leaflet, vena contracta > 6 mm, central jet with >65% of left ventricular outflow tract, pressure half-time < 200 msec, prominent holodiastolic flow reversal in the DAO, and enlarged left ventricle defined as left ventricular end-diastolic volume indexed to body surface area [LVEDVindex] > 2 SD) were considered to have severe AR. Quantitative methods were used whenever possible in patients with two to three specific criteria. Patients with RVol ECHO ≥ 60 mL or regurgitant orifice area ≥ 0.3 cm 2 were considered to have severe AR as well as patients with three specific criteria and RVol ECHO 45-59 mL or regurgitant orifice area 0.20-0.29 cm 2 . The RVol ECHO and regurgitant orifice area were obtained using the color Doppler flow convergence method. Echocardiography and CMR were performed within 4 hours. Exclusion criteria were moderate or more than moderate regurgitation in any other valve, presence of a cardiac shunt, any other form of significant cardiac disease, and irregular heart rhythm.
The study was conducted according to the Declaration of Helsinki. The Regional Ethics Review Board in Gothenburg gave ethical approval for the study protocol, and written informed consent was obtained from all participants.
Echocardiography
The echocardiographic investigation was performed using a commercially available imaging system (Vivid E9, GE Healthcare, Milwaukee, WI). Image analysis was performed using EchoPAC (GE Healthcare). End diastole and end systole were defined visually as the frames with the largest and smallest left ventricular cavities. Left ventricular volumes were acquired using the apical four- and two-chamber view according to the biplane method of disks to determine the end-diastolic and end-systolic volume. The PWD DAO was performed without a low-velocity filter, and the sample volume (2.5 mm) was positioned distal to the origin of the left subclavian artery. The EDFV was measured at the time point corresponding to the electrocardiogram’s (ECG’s) R-wave ( Figure 1 ). The recordings where classified as either having only backward flow or both backward and forward flow during diastole. The dVTI and sVTI were measured from the outer contour of the velocity envelope ( Figure 1 ), and the VTI ratio was calculated ((dVTI/sVTI) × 100). Dimensions of the left ventricular outflow tract, aortic root, and proximal part of the AAO was obtained from a parasternal long-axis view. The AAO was also investigated with the patient on the right side and the transducer on the right side of the sternum. The EDFV measurements were performed on three consecutive RR intervals. All echocardiographic analyses were performed in a blinded manner to CMR.

Cardiovascular Magnetic Resonance
CMR imaging was performed using a 1.5 Tesla scanner (Achieva, Philips Healthcare, Best, The Netherlands) with a five-channel phased-array cardiac coil. After standardized patient-specific planning, a series of cine-images was performed, first in the short-axis view covering the whole heart without gap from the atrioventricular ring to the apex, followed by cine-images in the common long-axis projections. All cine-images were acquired using balanced steady-state free precession sequences (repetition time 3.4 msec, echo time 1.7 msec, flip angle 60°) with retrospective ECG gating (30 phases per cardiac cycle) and parallel imaging (acceleration factor 2) during gentle expiratory breath hold. Typical in-plane spatial resolution was 1.7 × 1.7 mm, with a slice thickness of 8 mm. The quantification of the pulmonary and aortic flow was performed using through-plane phase-contrast (PC) velocity sequences (repetition time 4.8 msec, echo time 2.9 msec, flip angle 12°, 40 phases per cardiac cycle, field of view 320 × 260 mm, voxel size 2.5 × 2.5 mm, slice thickness 6 mm) with retrospective ECG gating during gentle expiratory breath hold. PC images were acquired perpendicularly aligned to the direction of the blood flow in the pulmonary trunk just above the pulmonary valve and at the level of the sinotubular junction (STJ) and in the proximal AAO, which even includes the DAO ( Supplemental Figure 1 , available at www.onlinejase.com ).
Analysis of the CMR Data
Image analysis was performed using ViewForum (Philips Healthcare). Left ventricular volumes were obtained by manual tracing of the endocardial contour in end diastole in the successive short-axis slices of the continuous short-axis stack and subsequently propagated through all phases using a semiautomated tracing algorithm, followed by manual adjustment, if necessary. Papillary muscles and trabeculae were included in the left ventricular cavity. Aortic flow was determined by integrating the velocity of each pixel of the respective region of interest during one heart cycle that consists of 40 phases. Graphically the flow rate (mL/sec) was presented as a function of time ( Figure 2 B). The areas under this PC flow rate curve (PC-FRC) in systole and diastole are the total stroke volume and the RVol, respectively. The regurgitant fraction (RF) is calculated from RVol/total stroke volume ratio in percent. Stroke volume was obtained at the STJ level and RVol, and RF was obtained at the STJ level and DAO. The net PC-FRC from the proximal DAO was reanalyzed using a research software (Segment v1.9 R2046), which enabled the decomposition of the net flow into forward and backward flow ( Figure 2 B). The DFF and DBF volumes were calculated from the diastolic part of the forward and backward PC-FRC. Furthermore, distinctive two-dimensional phase-contrast (2D-PC) velocity profiles describing the velocity distribution over the vessel area were plotted over one heart cycle using Segment. Based on the appearance of the PC-FRC in the proximal DAO during diastole, patients were classified into two groups: (1) complex flow with both backward and forward flow in the middle and late part of diastole (Group DFF) and (2) predominantly backward flow during diastole (Group No DFF).

Statistical Analysis
Continuous variables are expressed as the mean ± SD or median (range), unless otherwise stated. The degree of the linear relationship was assessed by the correlation coefficient ( R ). Simple and multiple regression analyses were used to study predictors of DFF in the DAO. Optimal cutoff values for the detection of severe AR were obtained by receiver operator characteristic curve (ROC) analysis. The diagnostic usefulness was described using sensitivity, specificity, and positive and negative likelihood ratios. Statistical analyses were performed using IBM SPSS Statistics 23 (IBM, Somers, NY).
Results
Patient age ± SD was 50 ± 15 years, and 85% were male. Twenty-one patients (53%) had a bicuspid valve. The regurgitation mechanism was cusp prolapse ( n = 30), annular dilatation ( n = 4), cusp perforation or rupture ( n = 4), and cusp restriction ( n = 2). Thirty-one patients (78%) had an eccentric jet. The AAO was dilated (>40 mm) in 19 patients (47%). Three patients (8%) had signs of low output defined as stroke volume index < 35 mL/m 2 by CMR in the pulmonary trunk.
Echocardiographic Grading of Severity
Complete echocardiographic data sets needed to apply the diagnostic algorithm could be obtained in 33 patients (83%). Quantitative Doppler was not possible to perform in the remaining seven patients (17%) with four or fewer specific criteria and diagnostic ambiguity. Twenty-five patients had severe and eight had moderate AR. The EDFV was below the recommended threshold of >20 cm/sec in 13 patients (52%) with severe AR. No patient with moderate AR had an EDFV > 20 cm/sec.
ROC analysis regarding the detection of severe AR as defined by the echocardiography algorithm showed large areas under the curve for both EDFV and dVTI ( Table 1 ). Lowering the cutoff value for EDFV from >20 cm/sec to >13 cm/sec improved the diagnostic performance. Using a cutoff value of 13 cm, the diagnostic performance of dVTI was superior to EDFV. A dVTI > 13 cm increased the likelihood of severe AR 7.4-fold, whereas dVTI < 13 cm decreased the likelihood 11.1-fold. The VTI ratio had low sensitivity and specificity regarding detection of severe AR.
Variable | AUC (95% CI) | Cutoff | Sensitivity (95% CI) | Specificity (95% CI) | PLR (95% CI) | NLR (95% CI) |
---|---|---|---|---|---|---|
EDFV, cm/sec | 0.91 (0.81-1.00) | >20 | 50 (31-69) | 100 (68-100) | ∗ | 0.5 (0.34-0.75) |
>13 | 76 (57-89) | 88 (53-98) | 6.1 (0.96-39) | 0.27 (0.13-0.58) | ||
dVTI, cm | 0.90 (0.77-1.0) | >13 | 92 (75-98) | 88 (53-98) | 7.4 (1.2-46) | 0.09 (0.02-0.35) |
VTI ratio, % | 0.70 (0.51-0.89) | >63 | 68 (48-82) | 50 (22-78) | 1.4 (0.6-2.9) | 0.64 (0.26-1.6) |
PWD Flow in the Proximal DAO versus CMR
The spatial distribution and direction of velocities in the proximal DAO throughout the cardiac cycle (40 phases) are illustrated in a patient with moderate AR (RVol 35 mL and RF 29%) in Figure 2 A. In late systole, an area with backward flow evolves and increases in size during early diastole with a counterclockwise rotation pattern. In mid and late diastole, the backward velocity is lower and there are regions with forward flow. Thus, from late systole and throughout diastole, there are both forward and backward flow. The PC-FRCs ( Figure 2 B) show the net flow rate, the forward flow, and the backward flow. The 2D-PC phases corresponding to the end-systolic net forward flow (*) and to a region with backward flow in early diastole (**) are shown. To illustrate the effect of different spatial distribution of the velocities, small regions of interest have been located in a lateral and medial position ( Figure 2 C). The corresponding PC-FRCs for the lateral ( Figure 2 D) and medial position ( Figure 2 F) are shown. Observe how the timing of the end of systolic forward flow is affected by the lateral (earlier) or medial (later) position. In the medial position in late diastole there is more forward than backward flow. Figure 2 E shows the PWD DAO recording in a lateral position, and the velocity envelope is comparable to the PC-FRC from the lateral region ( Figure 2 D) with no detectable forward velocities. Figure 3 depicts from the proximal DAO the 2D-PC velocity profiles ( Figure 3 A, 3B), PC-FRC ( Figure 3 C), and PWD DAO ( Figure 3 D) in a patient with severe AR (RVol CMR = 128 mL). The 2D-PC velocity profile as well as the PC-FRCs show only DBF, and the PWD DAO is in agreement with the CMR findings with only diastolic backward velocities. Figure 4 depicts a patient with moderate AR (RVol CMR = 29 mL) with significant DFF. In early and late diastole it is apparent from the 2D-PC velocity profiles ( Figure 4 A, 4B) that there are separate vessel regions with DBF and DFF, while in mid diastole there is predominantly DFF. The PWD DAO ( Figure 4 D) demonstrates clearly the forward flow component during diastole, but there are also backward flow velocities.


Importance of Complex Diastolic Flow Pattern in the DAO
Based on the visual analysis of the decomposed PC-FRCs, we found that 17 patients (43%) had predominantly DBF (Group No DFF), while 23 patients (57%) also had DFF in the proximal DAO (Group DFF). Patients without DFF tended to have less dilated aortic root and AAO, and they had significantly larger RVol compared with patients with DFF by PC-FRC data ( Table 2 ). Based on the visual analysis of the PWD DAO , we found diastolic forward velocities in 19 of the patients (83%) with DFF from PC-FRC data. The sensitivity, specificity, and positive and negative likelihood ratio (95% CI) of PWD DAO to detect nonuniform flow with DFF visualized by PC-FRC was 83% (63%-93%), 88% (66%-97%), 7.0 (1.9-26), and 0.19 (0.08-0.49), respectively. Seven patients with moderate (88%) and 12 patients with severe (48%) AR had signs of DFF by PWD DAO . The sensitivity, specificity, and positive and negative likelihood ratio (95% CI) of using DFF by PWD DAO to detect moderate AR were 88% (53%-98%), 52% (34%-70%), 1.8 (1.1-2.9), and 0.24 (0.03-1.6), respectively. Furthermore, the presence of DFF influenced the assessment of diastolic flow reversal. The relations between DAO-RVol CMR and either dVTI or EDFV were strong in patients without DFF, whereas the relations were weak in patients with DFF ( Figure 5 ). The RVol CMR and systolic backward flow in DAO were independent predictors of DFF ( Table 3 ), but the SFF was not. The AAO and DAO diameter did not influence the flow profile.
Variables | No DFF ( n = 17) | DFF ( n = 23) | P value |
---|---|---|---|
Age, years | 55 ± 15 | 47 ± 16 | .15 |
Gender, % male | 91 | 82 | .63 |
BSA, m 2 | 2.1 ± 0.25 | 2.0 ± 0.21 | .41 |
Heart rate, bpm | 66 ± 9 | 59 ± 9 | .02 |
Systolic blood pressure, mm Hg | 136 ± 22 | 136 ± 22 | .98 |
Diastolic blood pressure, mm Hg | 60 ± 12 | 74 ± 11 | .03 |
Echocardiography | |||
Bicuspid valve, % | 35 | 65 | .11 |
Left ventricular outflow tract, mm | 28 ± 3 | 27 ± 3 | .59 |
Sinus valsalva, mm | 40 ± 5 | 39 ± 5 | .51 |
STJ, mm | 33 ± 5 | 36 ± 5 | .07 |
AAO, mm | 39 ± 7 | 43 ± 7 | .09 |
Aortic arch, mm | 31 ± 4 | 30 ± 6 | .41 |
Proximal DAO, mm | 23 ± 4 | 21 ± 5 | .16 |
LVEDVindex Echo , mL | 117 ± 39 | 81 ± 16 | .002 |
Vena contracta, mm | 8.1 ± 1.1 | 6.4 ± 1.1 | .001 |
Pressure half-time, msec | 367 ± 129 | 641 ± 194 | .001 |
RVol ECHO , mL | 84 ± 37 | 52 ± 23 | <.0001 |
ROA, cm 2 | 0.4 ± 0.2 | 0.2 ± 0.1 | .002 |
EDFV, cm/sec | 24 ± 9 | 14 ± 5 | <.0001 |
CMR | |||
RVol CMR , mL | 79 ± 46 | 46 ± 26 | .009 |
RF CMR , % | 44 ± 14 | 32 ± 16 | .01 |
DAO-RVol CMR , mL | 48 ± 23 | 14 ± 10 | <.0001 |
DAO-RVol CMR in percent of RV CMR , % | 50 ± 11 | 30 ± 14 | <.0001 |
Systolic forward flow, mL | 109 ± 31 | 81 ± 18 | .002 |
Systolic backward flow, mL | 1 (0-7) | 1 (0-18) | .97 |
DBF, mL | 44 (10-90) | 20 (3-36) | <.0001 |
DFF, mL | 1 (0-8) | 8 (2-29) | <.0001 |
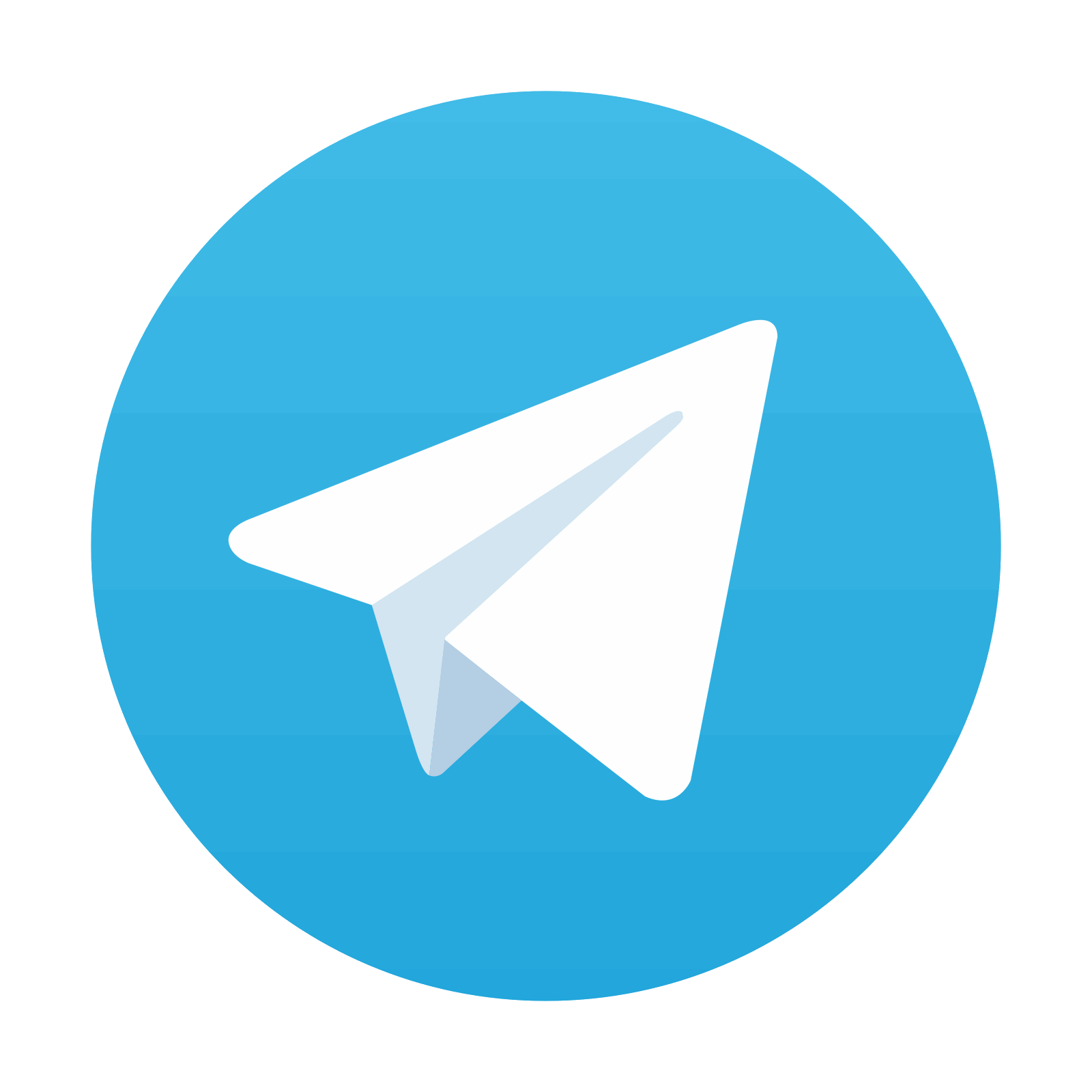
Stay updated, free articles. Join our Telegram channel

Full access? Get Clinical Tree
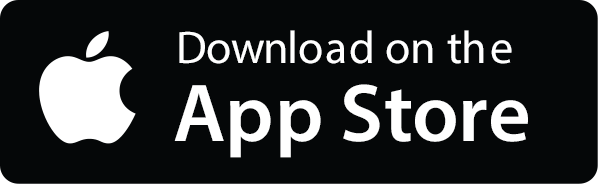
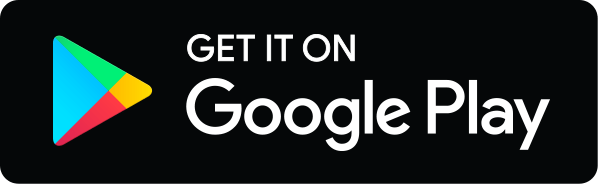
