Background
The aim of this study was to investigate the effects of transcatheter aortic valve implantation (TAVI) on left ventricular (LV) hypertrophy and diastolic function in patients with severe aortic valve stenosis (AVS). There are few and conflicting data on LV mass remodeling and LV diastolic function after TAVI.
Methods
Echocardiography and clinical assessment were performed at baseline and at 6 months in high-risk patients affected by severe AVS who underwent TAVI.
Results
One hundred thirty-five patients successfully underwent TAVI. Peak transvalvular aortic pressure gradient and mean transvalvular aortic pressure gradient were reduced from 87 ± 25 to 18 ± 7 mm Hg and from 54 ± 14 to 9 ± 4 mm Hg, respectively ( P < .001), accompanied by significant clinical improvement. The mean LV ejection fraction improved from 50 ± 13% to 54 ± 11% during follow-up ( P = .009). At 6-month follow-up, interventricular septal wall thickness regressed from 14 ± 2 to 12 ± 1 mm and posterior wall thickness from 13 ± 3 to 11 ± 2 mm ( P < .001). LV mass and LV mass index decreased from 332 ± 106 to 228 ± 58 g ( P < .001) and from 191 ± 58 to 132 ± 30 g/m 2 ( P < .001), respectively. Ninety-seven patients (72%) showed improvements in LV diastolic filling pattern. The mean e′ value increased from 4.1 ± 1.7 to 5.6 ± 2.2 cm/sec, and the mean E/e′ ratio decreased from 24 ± 7 to 17 ± 6 ( P < .001) after TAVI.
Conclusions
Significant LV mass reverse remodeling associated with improvement in LV systolic and diastolic function was found in patients with severe AVS 6 months after TAVI. These changes may have relevant clinical prognostic value.
Degenerative aortic valve stenosis (AVS) is the most common form of valvular heart disease in Western countries, and it is increasing in prevalence with an aging population. Conventional open surgical aortic valve replacement (AVR), using cardiopulmonary bypass, is the reference treatment in patients with symptomatic AVS. However, nearly one third of these patients are not accepted for surgical treatment, because of advanced age, comorbidities, and high surgical risk.
Consequently, a newer, less invasive technique, transcatheter aortic valve implantation (TAVI), is being evaluated as an alternative treatment for AVS in patients with excessive risk for surgical AVR.
TAVI for the treatment of AVS currently represents a dynamic field of research and development. The evidence suggests that this technique is feasible and provides hemodynamic and clinical improvement up to 2 years, but questions remain concerning long-term durability.
Aortic valve disease is associated with increased pressure and/or volume load of the left ventricle, causing left ventricular (LV) hypertrophy (LVH) associated with systolic and diastolic function disturbances, recognized risk factors for cardiac morbidity and mortality.
Conventional prosthetic aortic valves differ to some extent in their effects on LV function and LVH after AVR. There are still few and conflicting data on LV mass (LVM) remodeling and LV diastolic function after TAVI.
The remodeling of the left ventricle in patients with AVS is a complex process involving structural and functional changes. The early impact of TAVI on LVH and on LV diastolic function has not been extensively evaluated. Therefore, the objective of the present study was to investigate in a larger population of patients with AVS the effects of TAVI on LVM and changes in LV diastolic function in the months after prosthetic valve implantation.
Methods
The study population included symptomatic patients with critical AVS (aortic valve area ≤ 0.6 cm 2 /m 2 of body surface area), with or without aortic regurgitation. Patient-related inclusion criteria included age ≥ 75 years, logistic European System for Cardiac Operative Risk Evaluation score ≥ 15%, or age ≥ 65 years and one or more of the following complicating factors: cirrhosis of the liver (Child class A or B), pulmonary insufficiency (defined as forced expiratory volume in 1 sec < 1 L), pulmonary hypertension (pulmonary systolic pressure > 60 mm Hg), previous cardiac surgery (coronary artery bypass graft surgery or valvular surgery), porcelain aorta, recurrent pulmonary emboli, right ventricular insufficiency, contraindication to open-chest surgery (e.g., previous chest burns or radiation), or cachexia (body mass index ≤ 18 kg/m 2 ).
Exclusion criteria were any of the following: known contraindications to antiplatelet agents or anticoagulant therapy, nitinol or contrast media that could not be premedicated, any sepsis, myocardial infarction in the preceding 30 days, percutaneous coronary intervention <15 days before implantation or scheduled during or within 30 days after TAVI, uncontrolled atrial fibrillation (heart rate > 100 beats/min), history of AVR, any condition considered a contraindication to extracorporeal assistance, stroke within the previous month, symptomatic carotid or vertebral artery disease (>70% stenosis), abdominal aortic aneurysm, bleeding diathesis or coagulopathy, creatinine clearance <20 mL/m, and life expectancy < 1 year.
These patients were considered high-risk or nonsurgical candidates by a multispecialty team. Surgical risk was assessed by clinical judgment and logistic European System for Cardiac Operative Risk Evaluation score. Detailed medical history, physical examination, electrocardiography, color Doppler echocardiography, and coronary and peripheral angiography of the great arteries were performed. The current third-generation (18Fr) CoreValve ReValving System (Medtronic, Inc., Minneapolis, MN), consisting of a trileaflet porcine pericardial tissue valve mounted in a self-expanding nitinol frame, was used.
All patients were examined using two-dimensional transthoracic echocardiography before TAVI and at 6-month follow-up (Vivid 7; GE Medical Systems, Milwaukee, WI). LV internal dimensions and LV wall thicknesses were measured at end-diastole and end-systole. According to these parameters, we calculated LVM as follows: LVM (g) = 1.04 × [(LV end-diastolic diameter + LV diastolic posterior wall thickness + LV diastolic posterior wall thickness) − (LV end-diastolic diameter) ] × 13.6. The LVM index (LVMI) was obtained using the following formula: LVM/body surface area. LVMI was also derived by normalization of LVM for height 2.7 . LVH was defined as LVMI > 115 g/m 2 for men and LVMI > 95 g/m 2 for women. Relative wall thickness was calculated as 2 × LV diastolic posterior wall thickness/LV end-diastolic diameter and considered abnormal when >0.42. Relative wall thickness and LVMI were used to assess LV geometry.
Velocity-time integral, peak aortic velocity, peak instantaneous gradient, and mean transvalvular aortic gradient were measured by tracing the aortic waveform using the equipment’s software.
The aortic annulus was measured in a zoomed-in parasternal long-axis view, as recommended, and the LV outflow tract (LVOT) diameter was measured within 5 to 10 mm into the LVOT. Using the continuity equation, aortic valve area (LVOT area × [LVOT velocity-time integral/valve velocity-time integral]) was estimated and indexed to body surface area. In patients with sinus rhythm, the three best available consecutive signals were recorded and averaged. In patients who were not in sinus rhythm, a minimum of five consecutive measurements were averaged. After TAVI, the effective orifice area of the prosthesis was similarly calculated using the continuity equation approach and indexed to body surface area. Severe prosthesis-patient mismatch (PPM) was defined as an indexed effective orifice area < 0.65 cm 2 /m 2 .
Aortic and mitral valve regurgitation grades were assessed semiquantitatively according to current guidelines. LV ejection fraction (LVEF) was estimated according to LV volumes evaluated using the biplane Simpson’s method.
Maximal left atrial volume was measured using the biplane Simpson’s method and indexed to body surface area.
LV diastolic filling pattern was obtained from pulsed Doppler mitral inflow recordings in the apical four-chamber view, positioning the sample volume 1 cm below the mitral annular plane between the mitral leaflet tips. LV diastolic function was obtained from mitral Doppler tracings by peak flow velocity of early filling (E wave), peak flow velocity of atrial contraction (A wave), the E/A ratio, and early filling deceleration time (DT).
In addition, myocardial systolic and diastolic peak velocities were recorded using pulsed-wave Doppler tissue imaging (DTI). Systolic (s′) and early (e′) and late (a′) diastolic wave velocities were measured by placing the sample volume underneath the mitral annulus on the lateral and septal myocardial walls of the left ventricle from the apical four-chamber view.
Measurements of transmitral flow and annular tissue velocity were recorded from the averaged multiple consecutive beats.
Finally, the dimensionless E/e′ ratio was also calculated; we used the average e′ velocity obtained from the septal and lateral sides of the mitral annulus for the prediction of LV filling pressures.
By integrating these variables, LV diastolic dysfunction was classified as follows: normal function (grade 0); mild diastolic dysfunction (grade I, abnormal relaxation) if mitral E/A < 0.8, DT > 200 msec, pulmonary venous systolic velocity greater than diastolic velocity, annular e′ < 8 cm/sec, and average E/e′ ratio < 8; moderate diastolic dysfunction (grade II, pseudonormal) if mitral E/A = 0.8 to 1.5, E-wave DT = 160 to 200 msec, average E/e′ ratio = 9 to 12, e′ < 8 cm/sec; and severe diastolic dysfunction (grade III, restrictive) if E/A ≥ 2, E-wave DT < 160 msec, pulmonary venous systolic velocity less than diastolic velocity, and average E/e′ ratio > 13 (or septal E/e′ > 15 and lateral E/e′ > 12), as described in the American Society of Echocardiography and European Association of Echocardiography guidelines on the evaluation of LV diastolic function.
In patients who were not in sinus rhythm, e′ was used as an additional parameter to help determine the degree of LV diastolic dysfunction: impaired relaxation if e′ < 10 cm/sec, pseudonormal filling if e′ < 8 cm/sec, and restrictive filling if e′ < 5 cm/sec.
For patients with hypertension, medical therapy was instituted in the hospital to achieve a systemic blood pressure < 140/90 mm Hg, and therapy was reassessed and modified at each follow-up visit.
Statistical Analysis
Categorical variables were compared using χ 2 tests and are presented as frequencies. Continuous variables are presented as mean ± SD. Two-tailed unpaired Student’s t tests were used for comparisons between groups, and paired Student’s t tests were used for intragroup comparisons. P values < .05 were considered statistically significant. A multivariate regression model was used to identify independent predictors of LVM regression. The research on change in LVM had to take into account the great number of analyzed parameters in relation to the number of patients. We performed a first selection of the predictors, using Spearman’s rank correlation coefficient or Wilcoxon’s test. The approach of Benjamini and Hochberg was also used to adjust the P values for the multiple comparisons. Only parameters with adjusted P values < .05 were used in the linear multivariate regression model. The Gaussian distribution of the dependent variable was assessed using the Kolmogorov-Smirnov test ( P > .05). To avoid overfitting and overoptimism, we performed the selection of the most parsimonious model according to the approach of Austin and Tu. The goodness of fit was quite good (adjusted R 2 = 0.70).
Results
The demographic and clinical characteristics of the population at baseline are summarized in Table 1 . Our population comprised 135 elderly patients (mean age, 83 ± 7 years; 70 men, 65 women) with important comorbidities and a mean logistic European System for Cardiac Operative Risk Evaluation score of 21 ± 13 who were excluded for surgical AVR. All patients were implanted successfully using 26-mm and 29-mm inflow CoreValve bioprostheses. During hospitalization, 25% of the patients had complete heart block or relevant bradycardia associated with new-onset left bundle branch block. Therefore, they received permanent pacemakers. Remarkably, there were no periprocedural deaths.
Variable | Value |
---|---|
Age (y) | 83 ± 7 |
Women | 65 (48%) |
Systolic blood pressure (mm Hg) | 130 ± 17 |
Diastolic blood pressure (mm Hg) | 72 ± 11 |
Heart rate (beats/min) | 85 ± 12 |
Body surface area (m 2 ) | 1.7 ± 0.17 |
NYHA functional class | |
I | 0 (0%) |
II | 60 (45%) |
III | 70 (51%) |
IV | 5 (4%) |
Logistic EuroSCORE (%) | 21 ± 13 |
Weight (kg) | 68 ± 12 |
Height (cm) | 163 ± 7 |
Hypertension | 92 (68%) |
Diabetes mellitus | 64 (47%) |
Dyslipidemia | 42 (31%) |
Cardiac ischemic disease | 34 (25%) |
Previous CABG surgery | 25 (18%) |
Atrial fibrillation | 8 (6%) |
LV diastolic dysfunction grade | |
I | 1 (0.7%) |
II | 71 (52%) |
III | 60 (44%) |
IV | 3 (2%) |
Creatinine clearance (mL/min) | 45 ± 21 |
Hemoglobin (g/dL) | 11 ± 1 |
Significant clinical improvement was observed in the majority of patients during the 6 months of follow-up; mean New York Heart Association functional class improved from 3 ± 0.6 to 1 ± 0.6 ( P < .001; Figure 1 ). The echocardiographic parameters evaluated at baseline and during follow-up are summarized in Table 2 .
Parameter | Baseline ( n = 135) | Follow-up ( n = 135) | P |
---|---|---|---|
Vmax (m/sec) | 4.7 ± 0.6 | 2.1 ± 0.4 | <.001 |
AVA index (cm/m 2 ) | 0.33 ± 0.15 | 1.04 ± 0.40 | <.001 |
Peak TAPG (mm Hg) | 87 ± 25 | 18 ± 7 | <.001 |
Mean TAPG (mm Hg) | 54 ± 17 | 9 ± 4 | <.001 |
IVSDWT (mm) | 14 ± 2 | 12 ± 1 | <.001 |
LVDPWT (mm) | 13 ± 3 | 11 ± 2 | <.001 |
LVM | 332 ± 106 | 228 ± 58 | <.001 |
LVMI | 191 ± 58 | 132 ± 30 | <.001 |
LVM/height 2.7 | 88 ± 28 | 61 ± 14 | <.001 |
RWT | 0.51 ± 0.14 | 0.42 ± 0.09 | <.001 |
LVEF (%) | 50 ± 13 | 54 ± 11 | .009 |
E wave (cm/sec) | 0.95 ± 0.38 | 0.99 ± 0.11 | .23 |
DT (msec) | 210 ± 48 | 230 ± 71 | .08 |
e′ (cm/sec) | 4.1 ± 1.7 | 5.6 ± 2.2 | <.0001 |
E/e′ ratio | 24 ± 7 | 17 ± 6 | <.0001 |
S′ (cm/sec) | 5.9 ± 1.6 | 6.9 ± 1.6 | <.001 |
Mitral regurgitation grade | 2 ± 0.6 | 1 ± 0.6 | <.001 |
sPAP (mm Hg) | 41 ± 13 | 36 ± 11 | .001 |
LAVI (mL/m 2 ) | 40 ± 10 | 37 ± 9 | <.001 |
The procedure resulted in significant improvement in hemodynamics. There was a large significant postprocedural reduction in the peak transvalvular aortic gradient (from 87 ± 25 to 18 ± 7 mm Hg, P < .001) and in the mean transvalvular aortic gradient (from 54 ± 14 to 9 ± 4 mm Hg; P < .001) ( Figure 2 ). This resulted in a significant increase in aortic valve area index from 0.33 ± 0.15 to 1.04 ± 0.40 cm 2 /m 2 ( Figure 2 ). Severe PPM was not documented in this series of patients. The mean LVEF improved from 50 ± 13% to 54 ± 11% during follow-up ( P = .009). Improvement in LVEF was most pronounced in patients with LVEFs < 50%. In 19 patients (14%), the mean LVEF increased from 25 ± 5% to 41 ± 14% ( P < .001). In addition, DTI mean peak systolic wave velocity increased significantly, from 5.9 ± 1.6 to 6.9 ± 1.6 cm/sec ( P < .001).
LV end-diastolic volume remained unchanged (from 106 ± 42 to 100 ± 35 mL, P = NS), while LV end-systolic volumes decreased significantly from 56 ± 37 to 46 ± 26 mL at 6 months ( P < .001).
The hemodynamic improvements seen in this study were associated with a 13% reduction in septal and a 16% reduction in posterior wall thickness. At 6 months, interventricular septal diastolic wall thickness regressed from 14 ± 2 to 12 ± 1 mm and LV diastolic posterior wall thickness from 13 ± 3 to 11 ± 2 mm ( P < .001). LVM and LVMI decreased from 332 ± 106 to 228 ± 58 g and from 191 ± 58 to 132 ± 30 g/m 2 , respectively ( P < .001; Figure 3 ).
During follow-up, 128 patients (95% of the population) showed LVM regression > 10%, and 50 patients (37% of the population) showed LVM regression > 30% ( Figure 3 ). Concentric hypertrophy was the predominant LV geometric pattern and significantly improved at 6 months. Relative wall thickness decrease from 0.51 to 0.42 ( P < .001; Table 3 ).
Pattern | Baseline | Follow-up | P |
---|---|---|---|
Relative wall thickness > 0.42% | 92 (68%) | 85 (63%) | <.001 |
Normal LV geometry | 0 (0%) | 7 (5%) | <.001 |
Concentric LV remodeling | 16 (12%) | 18 (14%) | NS |
Eccentric LVH | 31 (23%) | 35 (26%) | NS |
Concentric LVH | 88 (65%) | 75 (55%) | <.001 |
On multivariate modeling analysis of covariance, adjusting for numerous factors, only baseline ventricular mass influenced the rate of subsequent LVM regression; the greatest rate of regression occurred in patients with the highest baseline values of ventricular mass ( P < .001). The basal value of LVM was the best predictor of its reduction during follow-up ( Table 4 ). History of hypertension, diabetes, and age were not found to be predictors of LVM regression.
Parameter | Estimate | SE | t | P |
---|---|---|---|---|
Female gender | −16.447 | 77.700 | 0.61 | .543 |
BSA | −73.262 | 11.399 | −1.44 | .152 |
Logistic EuroSCORE | 0.895 | 0.474 | 1.89 | .061 |
LVEDD | −3.975 | 1.828 | −2.17 | .052 |
LVSWT | −0.487 | 0.720 | −0.676 | .501 |
LVM | 8.349 | 0.913 | 9.14 | <.001 |
Peak TAPG | 1.357 | 0.506 | 2.68 | .08 |
Mean TAPG | −1.405 | 0.756 | −1.86 | .065 |
E/e′ ratio | 27.593 | 20.228 | −1.40 | .166 |
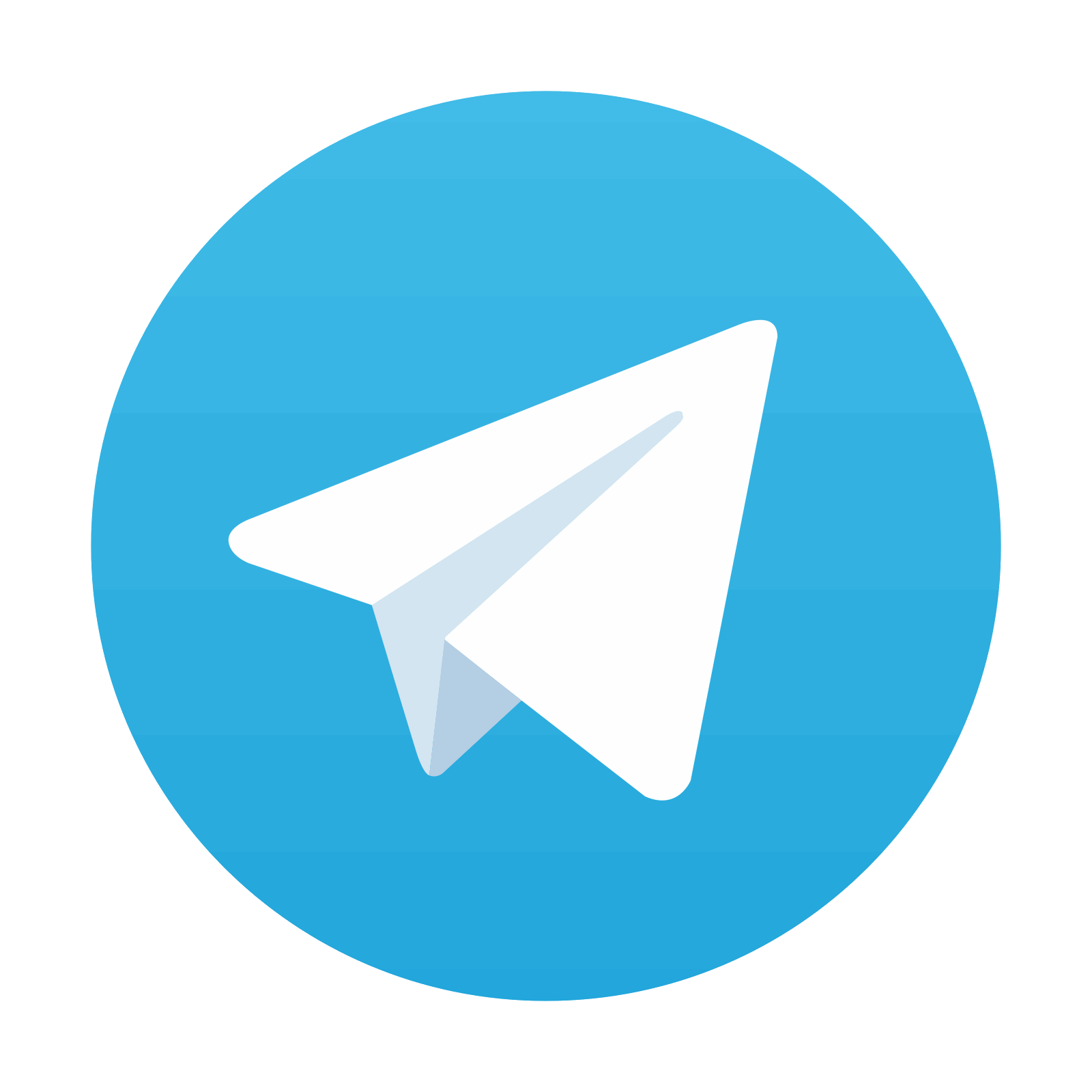
Stay updated, free articles. Join our Telegram channel

Full access? Get Clinical Tree
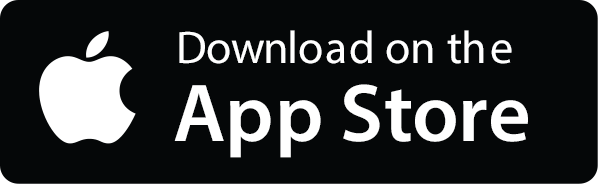
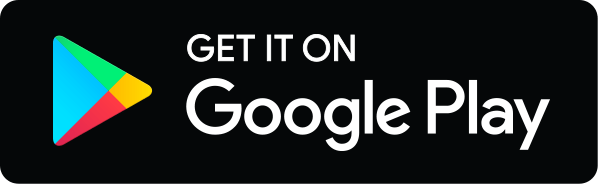
