Fig. 14.1
Autofluorescence bronchoscopy (AFB) image using AFI system. Representative case of carcinoma in situ. (a) White light bronchoscopy showed thickening of the bifurcation and partially covered with white coat. (b) Corresponding AFB image using AFI system (Olympus). The cancerous area was visualized as magenta lesion with clear boarder to normal mucosa
Narrow Band Imaging
Narrow band imaging (NBI) is an optical image technology classified as an image enhancement endoscopy using special blue and green light wavelengths allowing for enhanced visualization of microvascular structures in the mucosal and submucosal layers [18–20]. NBI utilizes wavelengths at 415 nm (blue light) and 540 nm (green light). Narrow bandwidths reduce the mucosal light scattering and enable enhanced visualization of endobronchial microvasculature structure. The 415 nm blue light is absorbed by the superficial capillary vessels, whereas the 540 nm wavelength is absorbed by the hemoglobin in the deeper, submucosal vessels. Fine blood vessels appear brown and the deeper vessels cyan.
Besides molecular changes allowing autonomous progression of cell cycle that imparts metastatic potential, cancer cells must also develop extended angiogenic capabilities allowing for rapid growth and invasion. Multistep angiogenesis process has been described in epithelial tumors [21, 22]. To fulfill high metabolic demands of rapidly dividing tumor, neoplastic cells have to develop enhanced angiogenic capabilities. Animal and human invasive neoplasia pathogenesis studies suggest that the so-called angiogenic switch is thought to occur in preinvasive lesions prior to invasive tumor formation [23, 24]. Since squamous cell cancer is thought to progress through developmental staged from squamous cell metaplasia to dysplasia and CIS, detection of each of these stages could have a significant impact on therapeutic interventions and prognosis (Fig. 14.2).


Fig. 14.2
Narrow band imaging (NBI) . Representative case of carcinoma in situ (same as Fig. 14.1). (a) White light bronchoscopy using high-definition bronchovideoscope. (b) Narrow band imaging (NBI) of the same area. (c) Close view of NBI identified dotted vessel and spiral-/screw-type vessels which were typically observed for carcinoma in situ
High Magnification Bronchovideoscope
High magnification bronchovideoscope (HMB) is a system that was developed to enhance detailed white light observation of bronchial dysplasia. Increased thickening of the bronchial epithelium and increased vessel growth are thought to be related to the appearance of areas of abnormal fluorescence, suggesting roles for neovascularization or increased mucosal microvascular growth in bronchial dysplasia. However, the only abnormality seen on WLB in dysplasia is swelling and redness at the bronchial bifurcations. HMB is a direct viewing WLB system that has an outer diameter of 6 mm and can easily be inserted into the tracheobronchial tree. HMB combines two systems—a video observation system for high magnification observation and a fiber observation system for orientation of the bronchoscope tip. For the video observation system, an objective optical system, in fixed focus mode rather than zoom mode, was used to give an outer diameter of about 6 mm to allow for the bronchoscope and the observation depth of 1–3 mm. Magnification is about fourfold higher than that of the regular bronchovideoscope. The bronchial mucosa is observed minutely on a 14-in. TV monitor at a high magnification of 110 times at the nearest point [25].
HMB has enabled observation of vascular networks within the bronchial mucosa in patients with respiratory disease such as asthma, chronic bronchitis, sarcoidosis, and lung cancer. Areas of increased vessel growth and complex networks of tortuous vessels in the bronchial mucosa that are detected using HMB at sites of abnormal fluorescence may allow clinicians to differentiate between bronchitis and dysplasia. In areas of abnormal fluorescence on AFB, HMB can detect dysplasia more accurately than AFB alone with a sensitivity of 70% and specificity of 90% [25]. HMB observation in patients with asthma showed that the vessel area density and vessel length density are significantly increased compared to control subjects [26].
Endobronchial Ultrasound
Two types of endobronchial ultrasound (EBUS) are currently available for clinical use. The radial probe EBUS first described in 1992 is used for the evaluation of bronchial wall structure, visualization of detailed images of the surrounding structures for assisting TBNA as well as detection of peripheral intrapulmonary nodules [27]. On the other hand, the convex-probe EBUS first described in 2004 has a built-in ultrasound probe on a flexible bronchoscope which enables bronchoscopists to perform real-time TBNA of mediastinal and hilar lesions [28].
Premalignant lesions or small intrabronchial radiologically invisible tumors are being detected more frequently as a result of new advanced mucosal imaging technologies. The decision to use endoscopic therapeutic intervention depends on the extent of tumor within the different layers of the bronchial wall. Conventional radiological imaging alone is not capable of distinguishing the tumor extent. The radial probe EBUS is a sensitive method for detection of alterations of the multilayer structure of the bronchial wall even in small tumors [29].
Optical Coherence Tomography
OCT is an optical imaging method that uses properties of light waves instead of sound waves [30]. OCT can generate high-resolution cross-sectional images of complex, living tissues in real time. Lam and colleagues investigated the ability of OCT to discern the pathology of lung lesions identified by AFB in a group of high-risk smokers and reported that normal or hyperplastic mucosa is characterized by one or two cell layers above a highly scattering basement membrane and upper submucosa [31]. As the epithelium changes from normal/hyperplasia to metaplasia, various grades of dysplasia, and CIS, the thickness of the epithelial layer increases. The basement membrane was still intact in CIS but became discontinuous or no longer visible with invasive cancer. Michel and colleagues examined five patients with endobronchial masses on the chest imaging with OCT [32]. OCT images showed differences between neoplasms and normal bronchial mucosa, and neoplastic lesions displayed irregular, ragged, dark lines between two light areas, which had the appearance of a fracture in the subepithelium.
Indications and Contraindications
By the use of its high sensitivity for detecting lung cancer as well as preinvasive lesions , the third ACCP guideline recommended AFB may be used as an adjunct modality when available in patients with severe dysplasia or CIS in sputum cytology who have chest imaging studies showing no localizing abnormality. In addition, patients with known severe dysplasia or CIS of central airways should be followed with WLB or AFB, when available [33]. AFB has also been shown to increase detection sensitivity of recurrent or new intraepithelial neoplasias and invasive carcinomas when added to WLB (from 25% for WLB alone to 75% when AFB is used in conjunction with WLB) in postoperative surveillance of patients who underwent curative resection for NSCLC [34]. AFB is also suggested for patients with early lung cancer who will undergo resection for delineation of tumor margins and assessment of synchronous lesions [33]. AFB combined with CT of the thorax in patients with radiographically suspicious and occult lung cancer has shown to be an effective lung cancer staging and tumor extension assessment modality with impact on therapeutic strategy choice [35, 36].
Currently the Lung SEARCH clinical trial of surveillance for the early detection of lung cancer in high-risk group is in progress [37]. The study targeted on 1568 high-risk individuals, and the patients who showed abnormal sputum receive annual CT and AFB screening to identify early lung cancer. However, before AFB and NBI can be incorporated into lung cancer screening, few issues need to be addressed. First, natural history of the squamous cell carcinoma (SCC) and bronchial dysplasia must be better characterized. SCC represents a third of all lung cancers diagnosed in the USA [1]. It is thought that pathologically, invasive cancer results from a stepwise process that begins with metaplasia, then dysplasia, followed by CIS, and finally invasive cancer. Previous studies showed development of invasive carcinoma in 40–83% of patients with severely dysplastic lesions [38, 39]. However, animal models and human studies show spontaneous regression of some of the lesions [40, 41]. Breuer et al. documented a 9–32% rate of malignant transformation for all dysplastic lesions in 52 patients followed over an 8-year period. Fifty-four percent spontaneous regression of all preinvasive lesions as well as non-stepwise transformation with development of invasive carcinoma at sites previously characterized as normal in appearance has also been described. These findings suggest that development of SCC may not always follow classic stepwise transformation pattern [41]. Also, population of patients at risk must be clearly identified and those with highest risk lesions (most likely to progress to invasive cancer) should be screened. Finally, appropriate therapeutic options and follow-up surveillance schedule must be developed based on evidence in order to decrease overall cancer mortality and recurrence [42]. Unfortunately, the recent data from the Pan-Canadian Lung Cancer Screening Study showed that the additional AFB only found one typical carcinoid tumor and one CIS lesion that were CT occult cancers. They concluded that additional AFB to LDCT in a high lung cancer risk cohort detected too few CT occult cancers (0.15%) to justify its incorporation into a lung cancer screening program [43]. Until all these issues have been addressed, the use of AFB and NBI will be predominantly in the research setting.
Description of the Equipment Needed
Autofluorescence Bronchoscopy
Safe-3000 system (Pentax, Asahi Optical, Tokyo, Japan) incorporated single action image switching and simultaneous display. Storz D-light (Storz, Tuttlingen, Germany) and Onco-LIFE systems (Xillix Technologies, Vancouver, Canada) combine autofluorescence and reflected light, all resulting in slightly different sensitivities as compared to WLB for pre- and malignant mucosal abnormalities detection.
Autofluorescence imaging (AFI) (Olympus Medical System Corp, Tokyo, Japan) is a new AFB system. The AFI system transmitted three wavelengths: excitation blue light (395–445 nm, to induce autofluorescence), 550 nm (red reflected light), and 610 nm (blue reflected light) [44]. Improved discriminatory nature of AFI system results from its ability to integrate three signals: autofluorescence signal with reflected green and red light signals [45]. Composite image displayed depicts normal epithelium as light green, areas of abundant blood flow seen not only in malignant epithelium but also in areas of chronic benign inflammation as dark green, and magenta color for malignant tissue due to mixed red/blue reflected signals and lack of the green autofluorescence signal [46] (Fig. 14.1). AFI demonstrated improved over the LIFE AFB system specificity (83% versus 36.6%) but slightly lower sensitivity (80% versus 96.7%) in detection of pre- and malignant bronchial lesions [46].
Confocal Laser Endomicroscopy and Endocytoscopy
The confocal laser endomicroscopy system is a new in vivo microscopic imaging device allowing the endoscopist to obtain real-time in vivo optical biopsies during ongoing endoscopy. The probe-based confocal laser endomicroscopy system (Cellvizio; Mauna Kea Technologies, Paris, France), which is capable of passage through the accessory channel of a standard endoscope, is available. Thiberville and colleagues observed 27 preinvasive lesions (metaplasia and dysplasia) and 2 invasive lesions and reported some specific basement membrane alterations within preinvasive lesions [47]. Methylene blue is a potent fluorophore, and its application to the target makes it possible to reproducibly image the epithelial layer of the main bronchi as well as cellular patterns of peripheral solid lung nodules [48]. Wellikoff et al. compared the images obtained by the probe-based confocal laser endomicroscopy and histological findings of biopsied malignant specimen in the same area [49]. They found an irregular connective tissue architecture with disorganization and fragmentation as well as mottling or “black holes” that represent nests of cells interrupting the fluorescence of the underlying connective tissue was correlated with a malignant diagnosis [49]. However, whether confocal laser endomicroscopy can discriminate among diseases requires additional studies.
The endocytoscopy system (ECS ; Olympus Medical System Corp) is another recently introduced, emerging endoscopic imaging technique enabling real-time in vivo diagnosis of cellular patterns at extremely high magnification [50]. The tip of the instrument contains an optical magnifying lens system and CCD. This endoscope can be inserted through the 4.2-mm biopsy channel and Olympus mother bronchoscope to become an “endocytoscope.” The ECS has a 570-fold magnification and provides an observation field of 300 × 300 μm, an observation depth of 0–30 μm, and spatial resolution of 4.2 mm for bronchial imaging. Shibuya and colleagues [13] reported that ECS was useful to discriminate between normal bronchial epithelial cells, dysplastic cells, and malignant cells during ongoing bronchoscopy. Another group used ECS in four patients for the immediate in vivo diagnosis of small cell lung cancer during ongoing bronchoscopy. ECS was able to reliably identify numerous small blue cells with hyperchromatic nuclei, which were confirmed in an in vivo diagnosis of small cell lung cancer by corresponding histopathologic diagnosis [50].
Raman Spectrophotometry
The use of Raman spectrophotometry system in addition to AFB and WLB may offer improved specificity (91%) in detection of preinvasive lesions, with only minor compromise in sensitivity (96%) as documented by a recent pilot study [52]. Laser Raman spectroscopy (LRS), currently used only in experimental setting, involves exposing the tissue to low-power laser light and collecting the scattered light for spectroscopic analyses [53]. This technology collects spectra nondestructively, and light scattered from tissues with different molecular composition can be easily differentiated. Using this technology can potentially reduce the number of false-positive biopsies for detection of preneoplastic lesions. The use of Raman spectra with AFB and WLB can offer a more objective airway mucosal assessment and detect more preneoplastic lesions. Also, Raman may be able to identify biomolecular changes in histologically preneoplastic and non-preneoplastic lesions that could be markers for development into late-stage malignancy. McGregor et al. examined 280 sites including 72 high-grade dysplasia/malignant lesions and 208 normal sites in 80 patients using real-time endoscopy Raman spectroscopy system. They could detect high-grade dysplasia/malignant lesions with a sensitivity of 90% and specificity of 65% [54]. More studies are needed to assess addition of this technology to armamentarium of tools for endobronchial neoplasia detection.
Application of the Technique
Autofluorescence Imaging and Optical Coherent Tomography
As previously described, autofluorescence imaging provides biochemical information about tissue by visualizing fluorescent tissue components such as collagen and elastin, and OCT provides high-resolution detailed information about tissue morphology. By combination of these two modalities, more precise observation of airway structure with emission of autofluorescence could be performed using ex vivo human lung [55]. This novel technology can apply for the peripheral pulmonary lesions, and the more precise observation on tumor tissue structure with vasculature information can be provided [56].
Supplemental Technology for Diagnostic Bronchoscopy
For the improvement of diagnostic rate of cytopathological material obtained by diagnostic bronchoscopy , several approaches have been attempted. By adding multitarget fluorescence in situ hybridization to conventional cytological smear, the sensitivity for detecting malignant cells was improved for bronchial brushing and washing specimens [57]. The immunohistochemistry for six protein expression including TP53, Ki67, MCM6, MCM7, KIAA1522, and KIAA0317 for bronchial brushing specimen improved the detection rate of lung cancer with sensitivity of 81.1% for non-small cell lung cancer and 83.3% for small cell lung cancer [58]. Recently a bronchial genomic classifier for the diagnostic evaluation of lung cancer has been reported [59]. In this study, epithelial cells were collected from the normal-appearing mainstem bronchus in current or former smokers undergoing bronchoscopy for suspected lung cancer. By evaluating 23 gene expressions, the diagnostic yield of bronchoscopy for the detection of lung cancer was improved with high negative predictive value of 91%. These advanced multidirectional analysis technologies will be the powerful support for detecting early lung cancer in combination with diagnostic bronchoscopy (Fig. 14.3).


Fig. 14.3
Several bronchoscopic imaging techniques of the airway. Representative case of microinvasive squamous cell carcinoma. (a) White light bronchoscopy, (b) AFB using AFI system, (c) white light bronchoscopy using high-definition bronchovideoscope, (d) narrow band imaging, and (e, f) endocytoscopy images using methylene blue staining of the mucosa
Evidence-Based Review
Multiple studies demonstrated that AFB improves detection of preinvasive central airway lesions and when combined with WLB also of squamous dysplasia, CIS, and early lung carcinoma. The meta-analysis of 21 studies comparing WLB used with AFB versus WLB alone in diagnosis of intraepithelial neoplasia and invasive lung cancer, involving 3266 patients, reported a pooled relative sensitivity of 2.04 (95% CI 1.72–2.42) on a per-lesion basis in favor of combined AFB and WLB approach [45]. Another meta-analysis showed that the pooled sensitivity of AFI and WLB was 0.89 (95% confidence interval [CI] 0.81–0.94) and 0.67 (95% CI 0.46–0.83), and the pooled specificity of AFI and WLB was 0.64 (95% CI 0.37–0.84) and 0.84 (95% CI 0.74–0.91), respectively [60]. However, the superiority of AFI in comparison with WLB is still controversial; as documented in previous individual studies, the sensitivity for detection of CIS and early invasive carcinomas was not superior to WLB alone (the RR of 1.15 at 95% CI 1.05–1.26) [45]. This suggests that while screening for invasive cancer WLB may be sufficient and more cost effective.
AFB can become a useful tool in endobronchial pre- and malignant lesion detection screening, especially in high-risk groups (patients with head and neck cancers, chronic obstructive pulmonary disease [COPD], and smokers) knowing that the incidence of synchronous lesions ranges from 0.7 to 15% and metachronous lesions might occur in as many as 5% high-risk patients annually [61, 62]. However, more studies are needed to determine how the AFB can best be incorporated into clinical practice in an economically efficient way and with reasonable reduction in lung cancer mortality.
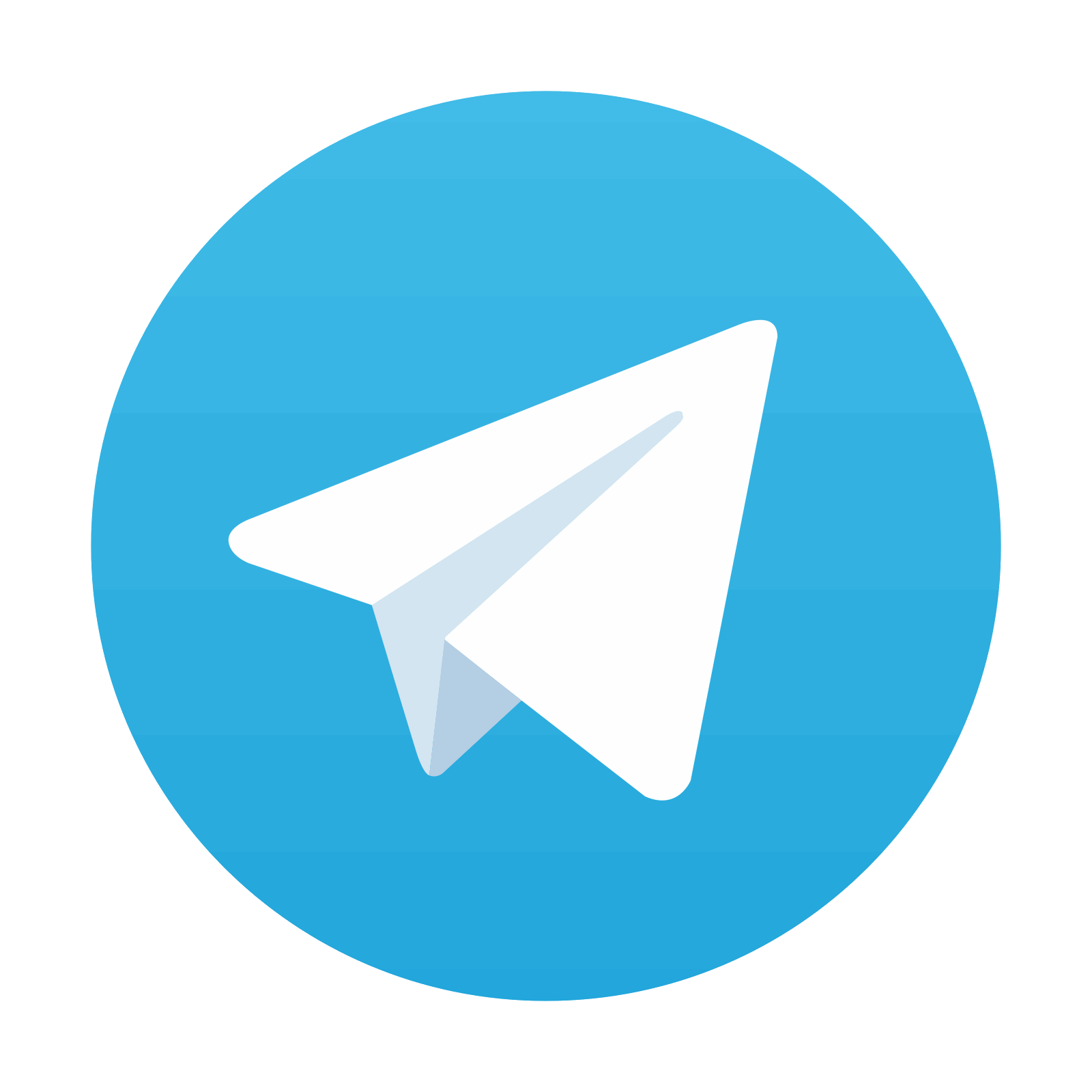
Stay updated, free articles. Join our Telegram channel

Full access? Get Clinical Tree
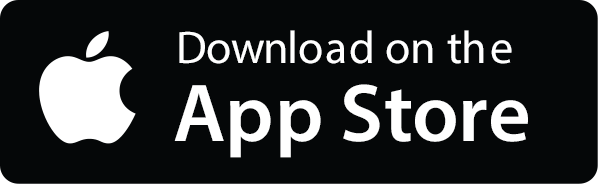
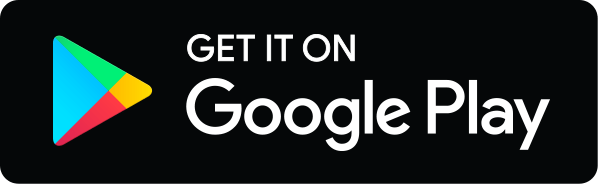