Chapter 7
Early cancer detection
Renelle Myers1,2 and Stephen Lam1,2
1Dept of Integrative Oncology, British Columbia Cancer Agency, Vancouver, BC, Canada. 2Dept of Medicine, University of British Columbia, Vancouver, BC, Canada.
Correspondence: Renelle Myers, 675 West 10 Avenue, Vancouver, British Columbia, Canada, V5Z 1L3. E-mail: renelle.myers1@bccancer.bc.ca
Lung cancer is the leading cause of cancer deaths worldwide as the majority of patients have advanced disease at diagnosis. Detection and early treatment of pre-invasive and minimally invasive lung cancer can significantly improve the current 5-year survival of <18%. Endoscopic optical imaging (such as autofluorescence imaging), NBI and OCT provide sensitive means to rapidly scan the central airways, in order to detect early lung cancer for biopsy confirmation and delineate the extent of the tumour spread to guide treatment. However, with the worldwide shift in lung cancer cell type to adenocarcinoma and the increasingly smaller lung lesions found by screening low dose CT, it is necessary to develop miniature imaging probes and better biopsy catheters to enable biopsy under real-time imaging.
Cite as: Myers R, Lam S. Early cancer detection. In: Herth FJF, Shah PL, Gompelmann D, eds. Interventional Pulmonology (ERS Monograph). Sheffield, European Respiratory Society, 2017; pp. 89–102. [https://doi.org/10.1183/2312508X.10002917].
With an estimated 1.8 million new cases and 1.6 million deaths in 2012, lung cancer is the most common cause of cancer death worldwide [1]. Clinical interventions over the last 40 years have had a minimal effect on lowering lung cancer death. The 5-year survival rate for lung cancer patients is currently <18%; this is because most are diagnosed when they become symptomatic with advanced incurable disease [2]. When stratified by stage, 70% of lung cancer patients who are diagnosed early (stage IA) survive ≥5 years, compared to <5% of those diagnosed late (stage IV). Improvements to early detection therefore hold significant promise in reducing mortality from this devastating disease. It is important to be able to detect in situ and minimally invasive lung cancers because of the survival advantage when detected early. Stage 0 (carcinoma in situ (CIS)), or micro-invasive, disease has a survival of >90% [3, 4]. Early malignancies can be adequately treated with minimally invasive endoscopic methods such as electrocautery, PDT [5] or cryotherapy [5–9]. In spite of the improved imaging capability of video bronchoscopy, early central type lung cancers remain difficult to detect with white light bronchoscopy; this is because these lesions are usually small and relatively flat, with subtle endobronchial changes [10]. Peripheral lesions in small airways or lung parenchyma are beyond the visible range of comparatively larger size standard video bronchoscopes. Advances in photonic imaging methods and fibreoptic probes offer advanced capabilities to use visible and near-infrared light to identify the early morphological, and biochemical changes occurring in the airways in pre-invasive and early invasive bronchial cancers. Photonic imaging is based on the physical phenomena that result when the bronchial surface is illuminated by light. Light can: 1) be reflected from the surface (specular reflection); 2) be absorbed; 3) induce autofluorescence; 4) travel in the bronchial tissue; 5) be back-scattered at the same wavelength as the incident light (elastic scattering); or 6) be scattered at a different wavelength (inelastic or Raman scattering) due to light energy modification by the vibrational state of molecules [11]. The simplest and most commonly used bronchoscopic imaging method is white light bronchoscopy (WLB). This method makes use of the specular reflection, back-scattering and absorption properties of broadband visible light from ∼400 nm to 700 nm, allowing it to define the structural features of the bronchial anatomy and surface features, in order to detect abnormal tissues. NBI, often used in conjunction with high magnification video bronchoscopy, [12–14], uses narrow band blue light centred at 415 nm coupled with green light centred at 540 nm (corresponding to the maximal haemoglobin absorption peaks) to highlight the vasculature of the airways. The blue light highlights the superficial capillaries while the green light penetrates deeper to highlight the larger blood vessels in the submucosa, providing a more detailed image of the microvasculature in pre-neoplastic and neoplastic lesions corresponding to the altered angiogenesis process.
This chapter reviews the principles of photonic imaging, the clinical application of these principles and the clinical trials supporting their ability to detecting early lung cancers.
Autofluorescence imaging and NBI
AFB uses fluorescence and absorption properties to provide information about the biochemical composition and metabolic state of bronchial tissues [11]. Bronchial tissue’s fluorescence properties are determined by the distribution of fluorophores, their distinct excitation and emission spectra, metabolic state and the tissue architecture. Autofluorescence also depends on the light attenuation (wavelength-dependent) concentration and distribution of non-fluorescent chromophores, such as haemoglobin [11]. Most endogenous fluorophores are either involved in the cellular metabolic processes or are associated with the tissue matrix. The most important fluorophores are structural proteins, such as collagen and elastin, and those involved in cellular metabolism, such as nicotinamide adenine dinucleotide (NADH) and flavins. Other fluorophores include the aromatic amino acids, various porphyrins and lipopigments.
When illuminated with violet or blue light (380–460 nm), normal bronchial tissues fluoresce strongly in the green (480–520 nm). When dysplasia or a neoplastic tissue is present in the bronchial epithelium, there is a progressive decrease in green autofluorescence and a proportionately less decrease in the red fluorescence intensity. These differences are caused by: the breakdown of stromal collagen cross-links; an increase in cellular metabolic activity leading to changes in NADH; flavin adenine dinucleotide coenzymes; and increased absorption of the excitation violet/blue light by haemoglobin due to angiogenesis. There are also changes in the light scattering process caused by an increase in nuclear size, cellular density and distribution of the cells associated with lung cancer development. An excitation wavelength of 405 nm produces the highest tumour to normal tissue light intensity and chromatic contrast [11, 15, 16].
The fluorescence differences seen at 480–700 nm in normal, dyplastic and neoplastic tissues have provided a basis for the design of several autofluorescence endoscopic imaging devices. These devices are in clinical use for the localisation of early lung cancer in the bronchial tree [17]. In 1991, PALCIC et al. [18] and LAM and co-workers [19, 20] reported using tissue autofluorescence for fluorescence diagnosis. Until then, an exogenous agent via injection had been required, which caused photosensitivity in the patient. When using autofluorescence alone, normal tissue appears green and abnormal areas appear reddish brown due to reduced green autofluorescence but maintenance of or an increase in red autofluorescence in dysplastic and neoplastic lesions. Commercially available AFB devices optimise the image quality through a combination of autofluorescence and reflectance imaging. Small amounts of reflected light (blue, green or near infrared) are used to form a reflectance image; this image enhances the chromatic contrast and normalises the green autofluorescence image to correct for non-uniformity caused by optical and geometrical factors, such as variable distances and angles between the endoscope tip to the bronchial surface [18, 19]. Depending on the type of reflected light used to combine with the fluorescence image, abnormal areas may appear brownish red, red, purple or magenta, while normal areas are green or light blue (table 1) [20–26]. Some devices allow white-light and fluorescence images to be displayed simultaneously [26, 27].
Table 1. AFB devices

Several meta-analyses and multicentre studies demonstrate the ability of AFB alone, or in addition to WLB, to improve the detection rate of precancerous lesions [28, 29]. SUN et al. [28] evaluated 21 studies involving 3266 patients. The pool relative sensitivity on a per-lesion basis of AFB plus WLB versus WLB alone to detect intraepithelial neoplasia and invasive cancer was 2.04 (95% CI 1.72–2.42) and 1.15 (95% CI 1.05–1.26), respectively. The pool relative specificity on a per-lesion basis of AFB plus WLB versus WLB alone was 0.65 (95% CI 0.59–0.73). AFB plus WLB is better than WLB alone in detecting pre-invasive lesions but there is not a lot of gain for invasive cancers. The lower specificity of AFB is due to false-positive changes related to inflammation and bronchoscopic trauma. As discussed later, training may also be an issue, with different colour schemes by different device manufacturers. Bronchoscopic trauma can be minimised by cough suppression and avoiding airway wall contact, as well as reducing suction during the bronchoscopic examination.
As NBI enhances visualisation of normal blood vessels and abnormal vasculature associated with the angiogenesis process and is less susceptible to artefacts from trauma and inflammation, a randomised trial was performed comparing AFB and NBI with WLB [30]. The sensitivity of WLB was 0.18 and the specificity was 0.88. In comparison with WLB, AFB had a slightly but not significantly higher sensitivity than NBI (3.7 versus 3.0). NBI has a comparatively higher specificity than AFB (1.0 versus 0.5). Combining AFB and NBI did not increase diagnostic yield significantly. ZHANG et al. [29] reported a meta-analysis that compared WLB, AFB and NBI. The study included 53 studies (39 WLB, 39 AFB, 17AFB and WLB, and six NBI), involving 6543 patients with 18 458 biopsies. It showed a sensitivity and specificity of: 54% (95% CI 46–61%) and 79% (95% CI 73–84%), respectively, for WLB; 87% (95% CI 82–90%) and 65% (95% CI 58–72%), respectively, for AFB; and 96% (95% CI 78–99%) and 84% (95% CI 70–92%), respectively, for NBI. A meta-analysis by IFTIKHAR and MUSANI [31], involving 632 patients who underwent NBI in eight studies, showed a sensitivity of 0.80 (95% CI 0.77–0.83) and a specificity of 0.84 (95% CI 0.81–0.86). Data from studies where NBI and AFB were used together showed a pooled sensitivity and specificity of 0.86 (95% CI 0.82–0.89) and 0.75 (95% CI 0.71–0.79), respectively. NBI had a high sensitivity and specificity, with both at >80% when assessing the range of pathology from hyperplasia to invasive lesions; however, when narrowing the pathology criteria from moderately dysplasia to invasive cancer, the specificity dropped to 43% [29]. Therefore, NBI appears to perform well for cancerous lesions but no conclusion could be reached for NBI’s performance when detecting precancerous lesions. Additional studies are needed.
Angiogenesis takes place relatively early in lung cancer pathogenesis [32]. NBI is able target the angiogenic features of neoplasia, improving the detection of pre-invasive lesions and differentiating these lesions from invasive carcinoma. The progressive pattern of neovascularisation correlates with the progression of invasiveness. Dotted vessels, increased vessel growth and complex networks of tortuous vessels of various sizes are observed with angiogenic squamous dysplasia. Dotted vessels and small spiral or cork-screw type tumour vessels are observed with CIS. In micro-invasive or invasive lung cancer, prominent spiral or cork-screw type tumour vessels of various sizes and grades are visible [12, 33].
Photonic imaging is also used by head and neck surgeons to examine vocal cord abnormalities and help guide biopsies. The addition of both AFB and NBI has a higher sensitivity and specificity than WLB alone in predicting malignant and premalignant lesions based on the vascular pattern surrounding the lesion. For bronchoscopists it can guide referral for concerning vocal cord lesions, as biopsies can often lead to dysphonia [34–36].
Recognition of abnormal vascular patterns for biopsy using NBI requires training. Development of an image-based program to teach NBI and other image-enhanced endoscopic technologies to identify areas for biopsy and for competency assessment is an important step in the realisation of the clinical benefits of new technologies (figure 1) [37]. The lack of standardisation by AFB manufacturers using different technology platforms (such as autofluorescence with green autofluorescence alone, green and red autofluorescence or a combination of autofluorescence and reflectance imaging (table 1)) makes developing training and competency testing tools that are similar to NBI very challenging. This may be a significant factor contributing to the low specificity of AFB. When using an objective measure by quantifying the red to green fluorescence ratio (R/G) of the target lesion during the bronchoscopic procedure, at a sensitivity of 85% to detect moderate/severe dysplasia and CIS, the specificity of AFB was found to be 80%. The specificity further improved to 88% by combining the R/G ratios with the visual score [38]. A higher false-positive rate was also found in a multicentre trial by EDELL et al. [21], in which bronchoscopists were unaware of the R/G ratios when making the visual classification of the bronchial mucosal changes. Quantitative imaging decreases intra- and inter-observer variation.

Figure 1. Example of dotted (end-on) blood vessels and spiral (convoluted) blood vessels visualised by NBI. a) The areas were marked by experts for a quiz used in training. The trainee must choose the type of pathology from the list and state their degree of confidence (options 1–4) for each area. There is also an area for comments. In this example, the areas in b are as follows. Expert area 1: abnormal mucosal end-on vessels; expert area 2: abnormal mucosal convoluted vessels; expert areas 3 and 4: abnormal mucosal end-on vessels; expert area 5: normal submucosal vessels; expert area 6: abnormal end-on vessels. Reproduced with the kind permission of Dr David Fielding (Royal Brisbane and Women’s Hospital, Brisbane, Australia) and Dr Cédric Dumas (Ecole des Mines de Nantes, Nantes, France).
The issue of training and competency assessment also applies to WLB. New technologies like AFB and NBI force bronchoscopists to look much more carefully at the mucosa for the subtle changes indicative of early bronchial cancer (e.g. loss of mucosal sheen, mucosal irregularity, loss of longitudinal or circular folds and thickening of subcarina) that pioneers in bronchoscopy had meticulously described in the past (table 2) [39].
Table 2. The endoscopic findings of early lung cancer
Carcinoma in situ | Submucosal invasion | Peribronchial extension |
---|---|---|
Loss of luster | Loss of longitudinal striations | Vascular engorgement |
Fine mucosal irregularity | Loss of circular folds | External compression |
Mucosal thickening | Indistinct bronchial cartilage | |
Pale mucosa | ||
Redness | ||
Nodular or polypoid lesion |
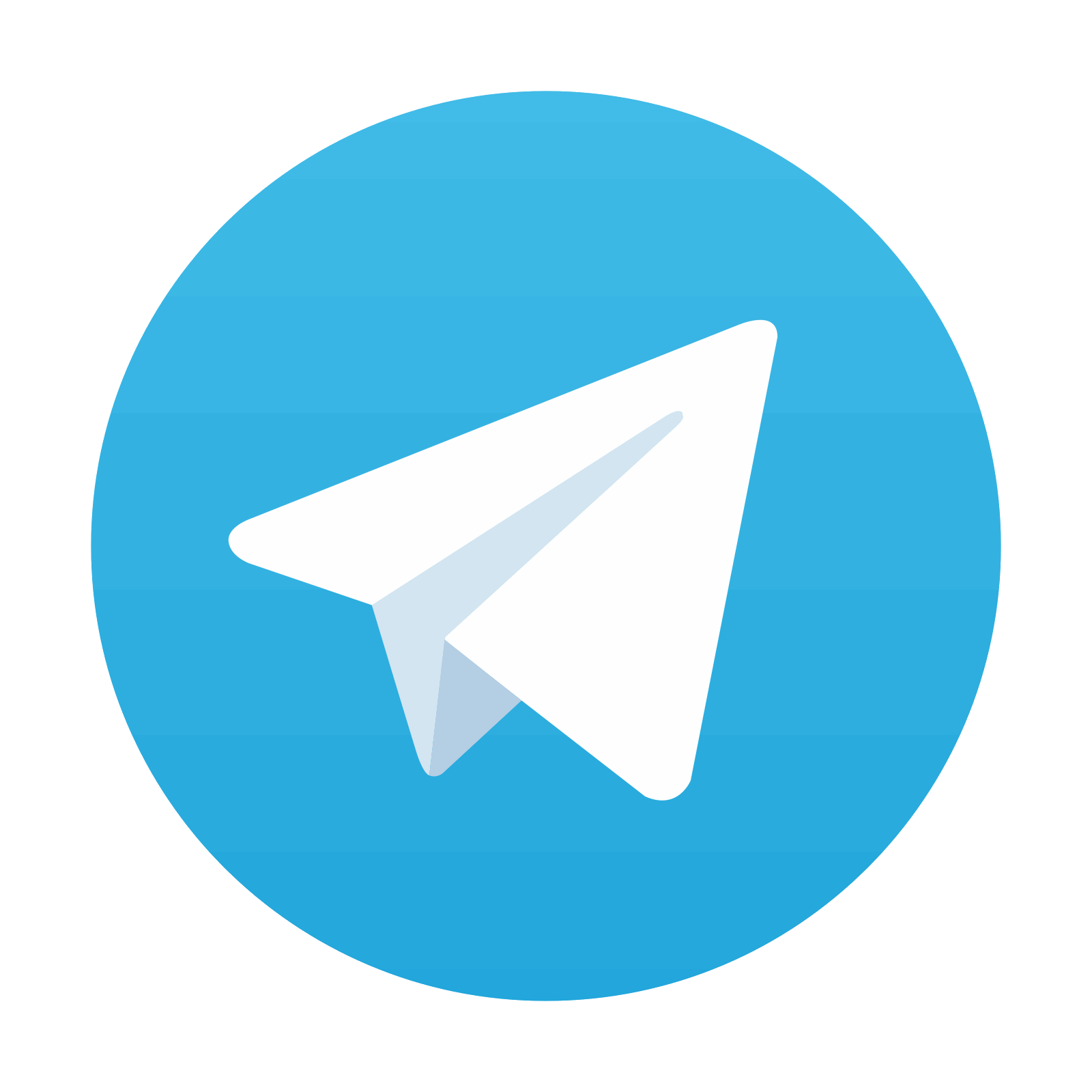
Stay updated, free articles. Join our Telegram channel

Full access? Get Clinical Tree
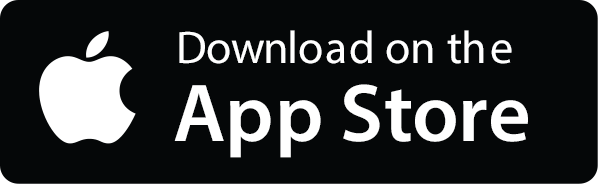
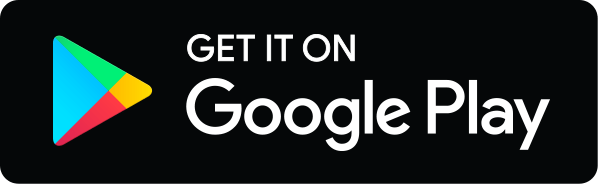