Chapter 3
Bronchoscopy in intensive care
Suveer Singh
Respiratory and Intensive Care Medicine, Royal Brompton and Chelsea and Westminster Hospitals, Imperial College London, London, UK.
Correspondence: Suveer Singh, Respiratory and Intensive Care Medicine, Chelsea and Westminster Hospital, 369 Fulham Road, London, SW10 9NH, UK. E-mail: suveer.singh@imperial.ac.uk
Bronchoscopy in the intensive care unit developed in the early 1970s. Its flexibility and versatility provide a crucial diagnostic and therapeutic tool. Indications are commonly to facilitate placement of a definitive airway, airway sampling for infection and clearance of secretions. More complex situations such as airway haemorrhage, percutaneous tracheostomy insertion, foreign body removal, burns inhalation injury, tracheal tears and persistent air leaks in mechanically ventilated patients are amenable to therapeutic bronchoscopy. Single-use portable bronchoscopes are important in the emergency setting, where a fast response, rapid visualisation and prevention of decontamination delays are essential. Bronchoscopy of the acutely sick awake patient is a valuable skill, requiring expertise and experience. An understanding of working endobronchial anatomy, physiological effects, and the risks and complications of bronchoscopy in this setting is necessary. Protocols for preparation, documentation and World Health Organization-style “time outs” should be encouraged. Training programmes still require significant development to ensure maintenance of satisfactory contextual and experiential skills.
Cite as: Singh S. Bronchoscopy in intensive care. In: Herth FJF, Shah PL, Gompelmann D, eds. Interventional Pulmonology (ERS Monograph). Sheffield, European Respiratory Society, 2017; pp. 29–48 [https://doi.org/10.1183/2312508X.10002517].
This chapter focuses on the use of bronchoscopy, specifically flexible bronchoscopy, in the intensive care unit (ICU) and other emergency settings. More general aspects of bronchoscopy are discussed elsewhere in this Monograph [1, 2].
History of bronchoscopy in intensive care
The first use of the fibreoptic bronchoscope in the ICU was reported by Marvin A. Sackner and colleagues in 1971 [3]. He described obtaining a flexible bronchoscope from Olympus, having attended a lecture by Dr Shigeto Ikeda in 1970. While the thoracic surgeons who performed rigid bronchoscopy were not initially impressed by the potential of the flexible scope, Sackner placed it through the endotracheal tube (ETT) of intubated patients, allowing a view of the effects of the inflated cuff on the tracheal mucosa. This engaged the thoracic surgeons in considering it as a tool for assessing airway integrity in their long-term ventilated postoperative patients. Sackner went on to demonstrate transnasal insertion of the flexible fibreoptic bronchoscope through a nasopharyngeal airway, a route that had gained acceptance for reliable tracheobronchial suction in the nonintubated patient [4]. Other applications of flexible bronchoscopy use in pulmonary medicine were described, including facilitation of intubation in the difficult airway of a patient with obstructive sleep apnoea (the first reported such interventional case in the USA [5]), selective aspiration of mucus plugs, observation of local tumours, lung collapse and stenosis, localisation of haemoptysis, and selective sampling of the lower airways [6].
Equipment
The flexible bronchoscope provides an image of the distal view either directly through an eyepiece, or as a processed video image projected onto a small screen connected to a video bronchoscope or to an external monitor connected to a processor unit. Disposable, single-use bronchoscope systems are invaluable in the ICU and other emergency settings. They are easily accessible, quick to use and relatively inexpensive. While the optics and image quality are inferior to the traditional videostack systems, they are being increasingly utilised for urgent therapeutic bronchoscopy and for luminal visualisation during percutaneous tracheostomy. Disposable systems contain a distal mounted camera illuminated by a light-emitting diode rather than fibreoptic cables. The image is transmitted via a cable in the device to the monitor screen. This arrangement combines quality of image, although inferior to conventional systems, with low manufacturing costs. Disposable systems eliminate the need for disinfection between procedures, and potentially reduce cross-contamination and infectious outbreaks, so long as they are discarded rather than reused.
Rigid bronchoscopy is rarely used in the critical care unit and will not be discussed further in this chapter.
Indications
The common indications for bronchoscopy in the critical care setting are diagnostic and therapeutic. Bronchoscopic surveillance is also important, either as part of optimising the endobronchial environment (e.g. prior to planned re-extubation in complex cases) or following certain therapeutic procedures (e.g. to reassess patency after relief of lobar collapse or post-endobronchial valve placement). A list of common indications is given in table 1.
Table 1. Indications for bronchoscopy in the critical care setting

Infection
Suspected respiratory infection, and the need for microbiological sampling, is the commonest reason for diagnostic bronchoscopy in this setting. Sampling modalities include nondirected bronchial lavage (NBL), bronchial washings, BAL, TBNA and protected catheter brushing.
Ventilator-associated pneumonia
Ventilator-associated pneumonia (VAP) develops in ∼5–20% of critically ill patients receiving mechanical ventilation, although published rates from observational studies are subject to variation, with attributable mortality suggested to be 2–27% [7, 8]. Symptoms are a combination of fever, purulent secretions, new, progressive or persistent radiographic appearances and worse inflammatory markers. The previously adopted convention of a minimum of 48 h mechanical ventilation to distinguish pre-ventilatory infection from that considered to be contributed or caused by the mechanical ventilation is losing favour, and moves to change terminology to infective (or noninfective) ventilator-associated complications are gaining credence. Ventilator care bundles are aimed at reducing these risks of VAP development. Inappropriate initial empirical antibiotic therapy is an independent predictor of increased mortality.
The diagnosis of VAP depends on the criteria used [9]. Accepted current systems include the Centers for Disease Control and Prevention criteria and the HELICS (Hospital in Europe Link for Infection Control and Surveillance) system, which allows various sampling methods (NBL or bronchoscopic washings) and semiquantitative microbiology [10, 11]. The diagnostic superiority of BAL over less invasive sampling techniques such as bronchial washings, NBL or blind catheter brushings is dependent on the protagonists and study design. Thus, a bronchial wash of 20 mL from a segment corresponding to a new or persisting area of radiological opacification, or from where purulent secretions emanate, is the standard practice for evaluation of suspected new pulmonary sepsis. However, BAL and semiquantitative microscopy may reduce the overdiagnosis of infection based upon the presence of any organisms in a NBL or bronchial wash, which may be colonisers or contaminants.
BAL involves serial insertion of 40–50 mL aliquots of up to 120–180 mL of warm saline into the relevant bronchial segment (depending on tolerance and oxygenation), through the wedged bronchoscope. The first 20 mL is discarded to reduce contamination from any components of bronchial secretions and as much of the remainder as possible is retrieved by suction. While there is no evidence of mortality difference in VAP [12], when comparing qualitative (presence or absence of pathogens in the culture) and quantitative (with a threshold CFU count of bacterial growth to differentiate between infection and colonisation of the lower airways) cultures obtained by bronchoscopic versus nonbronchoscopic techniques, the possibility of reducing unnecessary antibiotic prescriptions favours bronchoscopic sampling. This may further be relevant through the development of concomitant rapid biomarker sampling to rule out pulmonary sepsis [13–15].
Pulmonary infection in the immunocompromised patient
Pulmonary infection in the immunocompromised patient may be viral, bacterial, fungal, tuberculous, due to other organisms or copathogenic. The diagnostic value of bronchoscopic sampling in this setting is gaining in importance, not least as a clinical decision-making tool in rationalising multiple anti-infective medications.
The diagnosis of viral pneumonia is challenging. Sampling is important, particularly in the context of immunocompromise due to haematological malignancy. Once again, the challenge is pathogenicity or the “bystander” effect. A move to real-time PCR is emerging [16]. Pneumocystis jirovecii pneumonia (formerly Pneumocystis carinii pneumonia) is effectively diagnosed by BAL. Sensitivities of up to 98% are reported in treatment-naive patients. In those on prophylaxis, this is reduced when using indirect immunofluorescence staining [17]. In the diagnosis of tuberculosis, bronchoscopic sampling in the mechanically ventilated patient and its diagnostic accuracy are influenced by the clinical likelihood of disease and radiographic “targets”. Bronchial washes are usually sufficient for mycobacterial tuberculosis (MTB) diagnostics. PCR-based rapid diagnostic MTB and rifampicin resistance platforms such as the MTB/RIF (Xpert) assay provide high diagnostic accuracy in low- and high-prevalence cohorts, even when smear negative [18, 19]. The use of other sampling modalities such as TBNA may increase diagnostic yield. Invasive respiratory fungal infection is difficult to diagnose, with a high mortality (>80%) if untreated. Haematological malignancy, acquired and iatrogenic immunosuppressed patients most frequently require rapid diagnostics, often in the critical care setting. Diagnostic criteria are determined by the revised European Organization for Research and Treatment of Cancer/Mycoses Study Group guidelines [20]. The sensitivity of cultures is low. Current diagnostic methods are optical density assays to identify fungal cell wall elements (β-D-glucan for any fungi and galactomannan for invasive pulmonary aspergillosis) or PCR. The diagnostic accuracy of BAL galactomannan and/or PCR-based assays depends on the likelihood of disease. In likely or probable disease, using optical density cut-offs of 1.0 or 1.5, BAL galactomannan has shown sensitivities between 70% and 92%, with specificities up to 98% [21]. BAL β-D-glucan and serum galactomannan are as sensitive but less specific, while PCR has similar diagnostic accuracies. Combining BAL galactomannan and PCR provides the current best diagnostic accuracy [21, 22], although it should be noted that empirical treatment with antifungal agents reduces the accuracy of these tests.
Airway management
The bronchoscope is an invaluable tool in various situations, including the difficult airway, percutaneous tracheostomy, lobar collapse, foreign body removal, placement of double-lumen tubes (DLTs) or endobronchial balloon blockers, airway haemorrhage control and isolation of air leaks.
Difficult airway
Provision of bronchoscopes as part of difficult airway protocols has been established for many years. Awake fibreoptic intubation is the anaesthetic gold standard for the anticipated difficult airway. Failed intubation in the operating room is reported at <1%, whereas up to 25% of airway complications reported in hospitals in the UK were in the ICU or emergency department [23]. Here, a combination of unpreparedness or lack of a backup plan and lack of appropriate airway skills, including bronchoscopy, were identified [23]. The pre-placement of an ETT over the bronchoscope allows the ETT to be advanced into the trachea under direct vision. This may be done with or without sedation/neuromuscular blockade, the latter requiring communication, anticipation and cooperation of the patient. The access point (oral or nasal) will determine the size of the outer diameter of the bronchoscope (and the ETT).
By definition, access to the trachea and visualisation of the entry point (i.e. the vocal cords) is difficult, and often the use of multimodality tools can improve the chances of success. For instance, the flexible tip of the bronchoscope may not be easily manoeuvred through the vocal cords due to distortion or excess soft tissue/debris. Furthermore, advancing the ETT may be difficult. In these situations the use of a laryngoscope (direct or indirect viewing) or a supraglottic airway device (e.g. i-gel airway (Intersurgical, Wokingham, UK), laryngeal mask airway, Berman/Guedel airway or “Magic” airway) to allow better and quicker controlled direction of the bronchoscope to its target can be helpful (figure 1). The numbers and types of video laryngoscope continue to increase. All have particular characteristics providing improved visualisation of the vocal cords over conventional laryngoscopy. However, insertion of the ETT through the cords may in itself not be straightforward despite the view of the larynx. As such, bronchoscopy and these devices should be immediately available as part of a difficult airway protocol [24]. However, their order of use must be governed by the level of expertise and familiarity of the user, adopting published guidance and protocols (e.g. those of the Difficult Airway Society; www.das.uk.com). Indeed, there is no direct comparison of video laryngoscopy and bronchoscopy-assisted intubation in the critical care setting. A hybrid technique, using the channel of the video laryngoscope to direct the ETT-sheathed bronchoscope towards the larynx, has been used. Here, the bronchoscope is used as a manoeuvrable end-viewing bougie.

Figure 1. Supraglottic airway adjuncts through which the bronchoscope can be directed towards the larynx. A) Mouth guard. B) “Magic” airway. C) Guedel airway. D) i-gel airway. E) Armoured endotracheal tube (uncuffed) with paraxial channel.
There is now an increasing recognition of awake fibreoptic intubation being a training requirement by several international anaesthesia training programmes. Further information can be sought from agencies such as the Difficult Airway Society or the Airway Management Academy (www.airwaymanagementacademy.com).
Percutaneous tracheostomy
Direct visualisation of the endotracheal airway during tracheostomy has several inherent advantages over “blind” insertion. These include accurate percutaneous needle insertion, observation of cartilaginous ring integrity during dilatation and tracheostomy insertion, prevention of posterior membranous tracheal wall damage, confirmation of accurate final placement, and post-procedure bronchial segmental therapeutic lavage. While randomised studies have not shown an outcome difference with ultrasound guidance versus bronchoscopic guidance, the TRACHUS randomised noninferiority controlled trial has demonstrated the benefit of bronchoscopic guidance in reducing complications and its use is increasingly recommended [25, 26].
A few practical points should be highlighted. 1) The procedure may require a third operator, separate from the tracheostomist and assistant holding the ETT. 2) The bronchoscope tip should be within the distal end of the ETT at the time of needle insertion, so as to prevent damage to the bronchoscope. The use of disposable bronchoscopes may find a useful role in this setting. 3) An awareness of the partially occluded airway during withdrawal of the ETT and intraprocedurally should necessitate ongoing assessment of the airway pressure/volume and oxygen/carbon dioxide (CO2) profiles.
Lobar collapse
The cause of lobar collapse in the critical care setting is often multifactorial (table 2). A combination of predisposing disease-related risks, procedural impact on the lung, nonphysiological impacts of sedation, position, inadequate airway clearance mechanisms and lack of spontaneous ventilatory effort all contribute to lobar collapse. High inspiratory oxygen fraction (FIO2) can in itself lead to reabsorption atelectasis through nitrogen washout.
Table 2. Causes of lung collapse in the critically ill patient
Obstructive | |
Large airway | Mucopus, migrated endotracheal tube, inflammatory (tuberculosis, sarcoid), foreign body |
Small airway | Mucopus, inflammatory, foreign body |
Nonobstructive | |
Compression | Air trapping (COPD), lymphadenopathy (tumour, sarcoid, tuberculosis), malignancy |
Passive | Supine position, neuromuscular weakness, pleural effusion, pneumothorax, abdominal distension |
Adhesive | Post-cardiopulmonary bypass, smoke inhalation injury, acute respiratory distress syndrome (surfactant loss) |
Bronchoscopy is commonly used in lobar and complete lung collapse during mechanical ventilation, where physiotherapy or recruitment manoeuvres have been unsuccessful. It may identify migration of the ETT as a cause of the collapse. Directed suction is usually combined with forced saline flushing. Repeated episodes may be necessary, with intermittent periods of re-recruitment following temporary removal of the bronchoscope from the ETT. Concurrent mucolytics (e.g. nebulised N-acetylcysteine) can be used to treat airway plugging when nonpurulent mucus is tenacious (e.g. in COPD). It is considered not to be useful when there are purulent secretions or if FIO2 >40%, at which its activity is reduced.
The use of endobronchial DNase has been reported to improve the success of bronchoscopic lobar re-expansion in resistant paediatric cases [27]. DNase has been used safely and shown to improve chest radiography appearance compared with hypertonic or normal saline, but with no difference in other outcomes [28].
Foreign body removal
The removal of foreign bodies bronchoscopically in the critical care setting is uncommon. The nature of the foreign body, its location, potential impact, bronchoscopic tools and expertise available are factors in this setting [29]. A range of retrieval devices including grasping forceps, wires and baskets may be needed.
Cryoadhesion and extraction, using a through-the-bronchoscope probe, whose tip freezes on the foreign body by delivering high-pressure CO2 or nitrous oxide, is safe and effective in the bronchoscopy clinic [30, 31]. Its use in mechanically ventilated patients has not yet been formally evaluated.
General aspects of foreign body removal and the specifics of cryoadhesion/extraction are discussed in more detail elsewhere in this Monograph [32, 33].
Balloon blocker and DLT placement
Single-lung ventilation, through endobronchial isolation, can be achieved with accurate placement of bronchial blockers or DLTs by bronchoscopic visualisation. This may be for indications such as isolation of bronchopleural fistulas or alveolopleural fistulas, airway haemorrhage control, lung resection, transplantation, or differential lung ventilation.
Balloon blockers may be endobronchial (e.g. lumen <2 mm for subsegmental isolation) or paraxial, passing alongside the bronchoscope. This may be through the ETT, if space allows, or by passage outside the ETT, with bronchoscopy through the lumen of the ETT. Accurate positioning of the balloon blocker may require twisting it round at the proximal end and use of the tip of the bronchoscope to feed it down, either manually or by lassoing the loop wire of the balloon blocker onto the end of the bronchoscope. Certain balloon blockers have tip-deflecting ends allowing a certain degree of control over the direction of placement. Balloon blockers that fit through the working channel of the bronchoscope are used for various procedures such as assessment of collateral ventilation in COPD, (sub)segmental isolation of air leaks due to an alveolopleural fistula or temporary bronchial tamponade as part of airway haemorrhage control (no suction is possible while this balloon blocker occupies the working channel of the bronchoscope).
DLTs are conventionally inserted with bronchoscopic confirmation of positioning post-insertion. The endobronchial cuff should be identified in the appropriate bronchus, just below the carina and the patency of the upper lobe bronchus checked, noting the distal position of the tracheal lumen. The size of the bronchoscope needs to fit the inner lumen diameter of the smaller bronchial tube of the DLT. Bronchoscopic reassessment of position should occur if the patient is moved into a lateral position or if a change in ventilatory parameters suggests possible tube migration and obstruction. Common bronchoscope, ETT and DLT sizes are listed in table 3.
Table 3. Bronchoscope, endotracheal tube (ETT) and double-lumen tube (DLT) sizes

Airway haemorrhage
In the critical care setting, airway haemorrhage must be acted upon quickly from a diagnostic and therapeutic perspective. Major haemorrhage is fortunately rare, but may quickly become catastrophic. The clinical situation (e.g. medical or surgical patient, iatrogenicity, risk factors for coagulopathy and likely source of bleeding) needs to be considered when planning the strategy for haemorrhage control and patient management. Likely causes of haemorrhage may be local mucosal, tumour related, from cavitatory lung disease, anomalous vessels (either from fragile vessels in distorted endobronchial submucosa or from the higher pressure bronchial circulation), diffuse alveolar haemorrhage or traumatic. The risk of hypoxaemia and asphyxiation must be managed alongside haemodynamic control. Systematic approaches for control have been published, but there is no formal training in these strategies beyond various training workshops [34]. The use of endobronchial techniques or devices for the control of haemoptysis is well established, as part of a multidisciplinary approach.
Definitions of severity based upon volume of haemoptysis retrieved are not helpful beyond documentation. More useful is a change in the rate of bleeding and the time from intervention to control. Once bleeding is settling, it is imperative to survey the other airway segments and clear spillover blood that will quickly form a tenacious clot. Bronchoscopy is performed to identify the source of bleeding, isolate the remaining lung and create a tamponade until there is control of the bleeding.
Haemorrhage management involves suction above the point of bleeding, administration of cold saline, diluted adrenaline (1:100 000 to 1:200 000; although up to 1:10 000 has been used, there is concern about cardiovascular risks at higher doses) and tamponade of the source segment with the bronchoscope. If necessary, tamponade with a through-the-bronchoscope (loss of suction) or paraxial balloon blocker are options.
Other options such as endobronchial tranexamic acid or surgical gauze packing have been proposed as further measures for controlling the bleeding [35]. Methods such as turning the patient onto the side of the bleeding, DLTs and the use of noncontact strategies such as endobronchial APC have also achieved success in isolated reports, but outside the critical care setting [36]. The use of Watanabe spigots or endobronchial inspiratory-only one-way valves to control recurrent bleeding by isolating the source has been reported in selected situations. However, acute haemorrhage control would have to be established first by alternative means and the technique cannot be recommended in the acute setting [37, 38].
Use of antifibrinolytic agents, specifically tranexamic acid administered intravenously for haemoptysis in tuberculosis, may shorten the duration of bleeding, but remission is unclear from limited studies. Tranexamic acid may also be delivered endobronchially. In the specific situation of diffuse alveolar haemorrhage, due to vasculitides, post-haemopoietic stem cell transplant, drugs or toxins, untreated mortality is >50%. Serial instillation of endobronchial recombinant factor VII at doses of 30–50 mg·kg-1 in addition to systemic glucocorticoids and aminocaproic acid (an antifibrinolytic agent) resolved this in a small case series [39]. The airway haemorrhage literature appears to confirm that some form of tamponade is the most effective way of controlling immediate local endobronchial bleeding.
The patient’s cardiorespiratory status, source and rate of control of bleeding will determine the need for sequential actions. A multidisciplinary approach is essential with rapid recourse to alternatives such as rigid bronchoscopy, interventional radiology or surgery if initial bronchoscopic strategies fail. The role of bronchial artery embolisation via interventional radiology is well documented, with low complications when good pre-embolisation anatomy is definable [40]. Surgical resection must also be available if necessary and feasible, with good reported success rates of bleeding control related to mycetoma.
The interventional pulmonology management approaches to haemoptysis are discussed further elsewhere in this Monograph [41].
Air leak isolation and resolution
Persisting pneumothorax may result from thoracic surgery, chest trauma or as a complication of underlying pleuroparenchymal lung disease. The air leak is either due to a bronchopleural fistula, usually following surgery, or an alveolopleural fistula. In some cases the air leak does not seal and alternative treatments are needed such as surgery or inserting a foam/gel to seal the leak. Endobronchial (inspiratory-only) valves can be used for patients with air leaks for whom the usual treatments have not worked or for those unable to undergo surgery. Insertion of endobronchial valves for persistent air leaks aims to reduce or eliminate airflow through the leaks so that the rest of the lung can function normally. They may also allow the tissues around an air leak to heal so that the leak stops. In the critical care setting, they have reported success rates up to ∼50% [42].
The first stage is to identify and isolate the bronchial segment(s) involved. This involves placing a through-the-bronchoscope balloon blocker into and occluding the suspected segment(s) (having first adjusted ventilatory and oxygenation settings for the procedure and cleared the airways of mucus and debris). The balloon blocker is then fully inflated while monitoring the airflow reduction/cessation at the external pleural flow device (e.g. underwater seal bottle). Having removed the balloon blocker, a one-way valve mounted on a flexible catheter is passed through the bronchoscope and inserted into the target airway(s). Several valves may be placed and are generally removed some weeks/months after resolution. Serial bronchoscopic surveillance is necessary to check the position of the valve and clear away mucus, which may occlude the valve and limit its effectiveness.
General therapeutic interventions for airway fistulas are discussed in more detail elsewhere in this Monograph [43].
Airway assessment
Assessment of the airway, both upper and lower, can be diagnostic, therapeutic or for surveillance in certain situations.
Smoke inhalation injury
This occurs in at least 5% of patients with major burns (>20% total body surface area) and contributes to their mortality. It may increase mortality by 20% alone and up to 60% if also complicated by pneumonia [44]. Moreover, it increases the risk of pulmonary sepsis [44]. It is the most frequent cause of death at the scene of a fire. Injury to the airway and tracheobronchial tree may also result from inhalation of chemicals (e.g. chlorine gas), drugs and biological weapons. Systemic disturbances are common, but depend on the nature and toxicity of the inhaled substance.
Airway swelling/obstruction is progressive and may be exacerbated during fluid resuscitation, emphasising the need for constant reassessment [45]. Hoarse voice, carbonaceous sputum on deep cough, singed facial and nasal hair, and erythema and oedema of the mucosa in the mouth should raise suspicions of potential airway compromise. Swelling may not reach its maximum until 24 h after injury and may occur rapidly during fluid resuscitation. Early bronchoscopy is recommended to record the level and degree of injury.
Careful evaluation of the upper airways, especially in patients who present no evidence of more distal injury, is extremely important during bronchoscopy. Severe oedema in the supraglottic area or a large quantity of upper airway secretion may indicate that these patients are more likely to present acute airway obstruction and this is an indicator for the early intubation of patients suspected of having upper airway injury.
The diagnosis of inhalation injury is clinical, based upon a history of smoke inhalation and physical findings of facial burns, singed nasal hairs, oedema, erythema and soot from the upper or proximal lower airways. Bronchoscopic visualisation identifies soot, erythema, oedema or ulcerations in the upper and lower airways. Of note, severe vasoconstriction from hypovolaemia may mask significant injury initially in the absence of soot. Apart from this exception, bronchoscopy has a high accuracy in confirming the diagnosis of established inhalation injury.
A macroscopic grading system allows standardisation of observed findings (table 4) [46]. However, there is only weak evidence to suggest a correlation between this measure of endobronchial inhalation injury and subsequent outcomes [47]. This is likely due to the variable distal effects of the less soluble inhaled injurious gaseous agents, which bypass the upper airways [48].
Table 4. Burns inhalation injury: bronchoscopic grading system

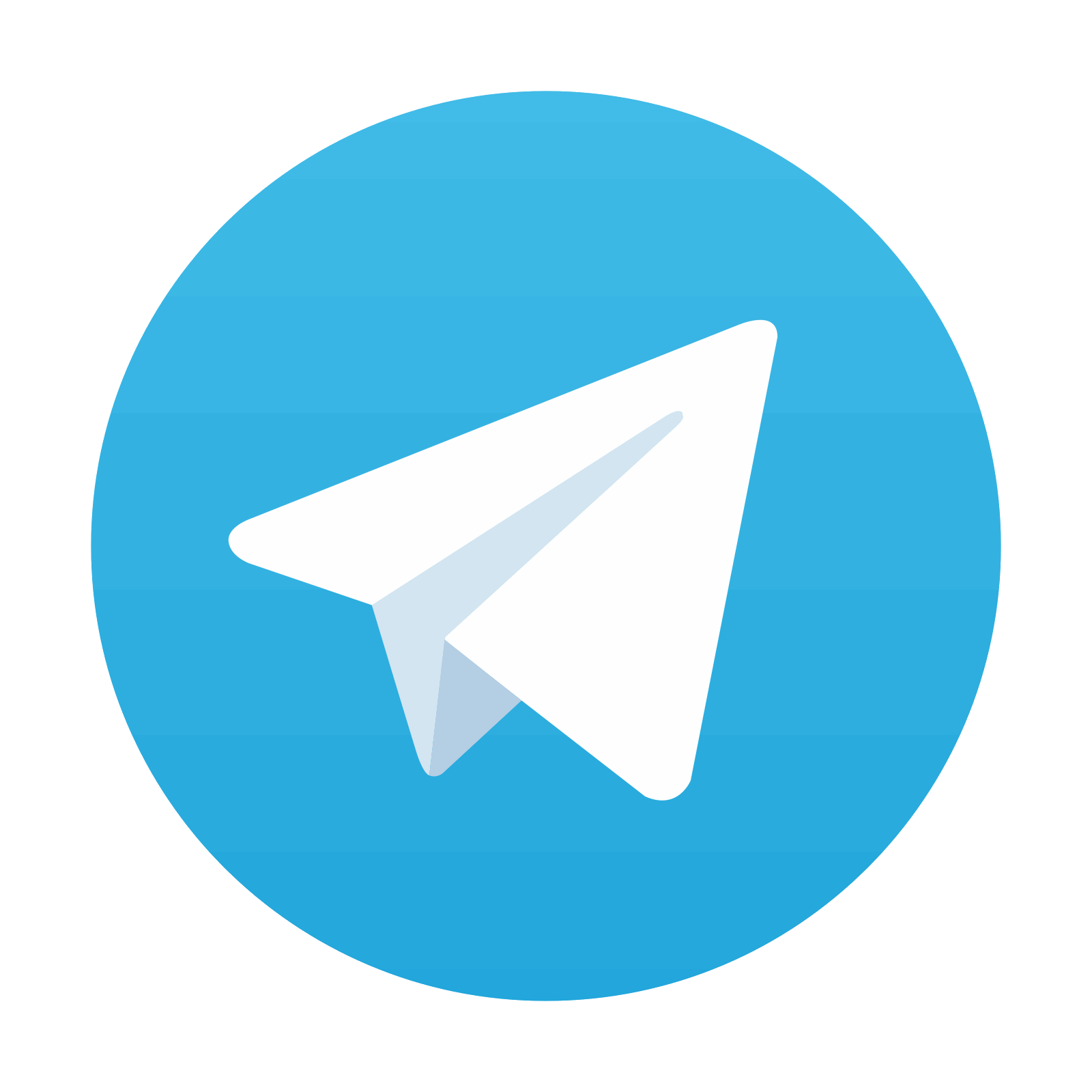
Stay updated, free articles. Join our Telegram channel

Full access? Get Clinical Tree
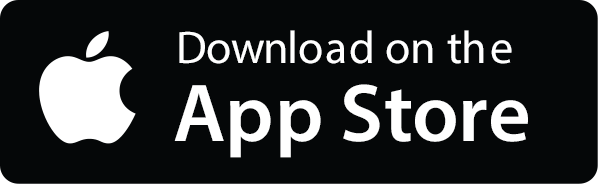
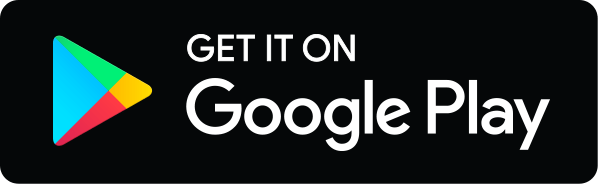