There are three studies in this issue of The Journal that contribute to our understanding of the noninvasive determination of pulmonary hemodynamics. This editorial discusses aspects of each of these studies and places them in context of the contemporary practice of echocardiography. The editorial concludes with a discussion of improving current practice and research, partly based on the model of aortic stenosis (AS).
Background
In 1981 Skjaerpe and Hatle described the general concept of obtaining hemodynamic data from continuous wave Doppler flow signals of tricuspid regurgitation (TR). In 1985 Yock and Popp and 1986 Skjaerpe and Hatle, among other groups, independently conducted the first catheter–based confirmations of calculating the peak systolic pressure drop between right atrium (RA) and right ventricle (RV) by applying the simplified Bernoulli equation to Doppler detected peak TR velocity. In all of the studies, an estimation of RA pressure was added to obtain peak RV systolic pressure. The accuracy of the determination was found valid across the range of TR severity, including small regurgitant orifice areas. Subsequently, the determination of peak RV systolic pressure (usually considered as equivalent to peak pulmonary artery (PA) pressure if there is no obstruction to RV outflow) has become a standard component of the routine echocardiography examination. Practically speaking, Doppler is the only routine access to PA pressure for most practitioners, most of the time.
Nonetheless, the wide dissemination of routinely measuring RV peak systolic pressure has met with considerable pushback. While there are many reasons for this challenge, erratic if not disappointing performance by clinical laboratories in making the diagnosis of pulmonary hypertension (PHTN) was primary among them. However, while some laboratories have showcased inaccurate correlations in pulmonary specialty journals, others are able to provide more accurate correlations of right heart hemodynamics. Furthermore, echocardiographic measurements of right heart hemodynamics are themselves prognostic for future events.
Yet in echocardiography, there is a close analog to Doppler right heart hemodynamics that has been widely accepted, applied, and that no longer endures pushback. That analog is the echocardiographic quantitation of AS. The Doppler hemodynamics of quantification of the severity of AS were also pioneered by Hatle but, unlike pulmonary hemodynamics, in current practice they do not rely on a single determination of a peak pressure. Despite the experience with AS, two of the three studies in the current issue of The Journal indicate that we are still rooted in the practice of defining pulmonary pressure by a single parameter, peak systolic pulmonary pressure, and needlessly preoccupied in its defense. In AS, the success of echocardiography can be attributed to having adopted the practice of measuring not only peak pressure but mean pressure, the flow that drives the pressure gradient, and their derivative, valve area. These additional parameters have relegated diagnostic catheterization to a little performed determination, and echocardiography has become the reference standard that informs clinical decisions.
In fact, the critics of echocardiography Doppler determination of peak systolic pulmonary pressure point to the fact that it disagrees with the anointed gold standard, right heart catheterization (RHC). While we are unaware of reproducibility studies of RHC, a study by Come et al compared aortic valve area invasively calculated on two successive days. The first catheterization was diagnostic and the second was performed in conjunction with balloon valvuloplasty. The correlation between the two determinations was weak (r = 0.42). Therefore, we must allow for the plausible possibility that invasive peak pulmonary pressure determination, rather than a true “gold standard,” may also be a variable entity with undefined and untested confidence limits. This suspicion may be shared by practitioners and advocates of RHC because they have designated the guideline driven diagnosis of PHTN as being based primarily on mean pulmonary pressure and not on peak PA systolic pressure (PASP).
Studies Reported
Lafitte et al. sought to study the diagnosis of PHTN in a university cardiology department in Bordeaux, France, performing 15,000 transthoracic echocardiographic exams and 4,500 RHCs annually. The study population numbered 310 patients, each of whom had both echocardiography and RHC; a sub-study was performed on 115 subjects in whom the RHC was performed within two days of one another. The retrospective design of the study limited bias in favor of echocardiography in that it was strictly a data base review of reports generated by multiple readers from studies made by fellows, nurse sonographers, and senior clinicians. No contrast was used to enhance TR signals, and TR and inferior vena cava size and collapse were the only right heart Doppler parameters evaluated. Presumably these were the only right heart measurements routinely performed in the laboratory clinical protocol. RHC was performed by “senior physicians” using fluid filled catheters on nonsedated patients. Both peak and mean PASPs were compared with echocardiography for the purpose of this study. It may be noteworthy that there was no control over the independence of the data. That is, some echocardiographers may have known the results of the catheterization when reading, and some performing RHC might have known the results of echocardiograms. Invasive and noninvasive measurements correlated at r = 0.80 with mean difference of 3.6 +/− 15.3 mmHg. Accuracy of correct classification as to whether a patient had PHTN or not was 86% but, importantly, accuracy improved to 89% when the studies were done within two days of one another. Valvulopathy had a nonsignifiant trend toward misclassification with no other disease group significantly associated. The peak systolic pressure that most accurately identified patients who would be classified as PHTN (based on a mean pulmonary pressure of 25 mmHg by RHC) was a Doppler peak PASP of 38 mmHg. However, despite the claims by the authors of high accuracy, sensitivity and specificity, inspection of the plot of the raw data (RHC vs Echo) reveals considerable scatter. For example, if a pulmonary pressure of 40 mmHg is found on echo, the RHC may range from 20 to 60 mmHg. The Bland Altman plot is no more encouraging and variance between the measurements is obvious. In our opinion, these data seem to demand a more comprehensive multiparameter approach to the diagnosis of PHTN and to right heart hemodynamics in general by echocardiography.
Steckelberg et al. , starting with the premise that “mean pulmonary pressure can be difficult to derive with current noninvasive methods,” designed a study to derive a regression equation from which to estimate mean PA pressure from peak systolic pressure measured at RHC. In this retrospective study they evaluated two groups of patients. The first group included 198 randomly selected patients who underwent RHC between 2002–2004, and the second a validation cohort of 109 collected in the first 5 months of 2011 who underwent both RHC and echocardiography up to one month apart. The catheterization protocol was carefully described with the mean PA pressure derived from area under the systolic pressure curve divided by the pulse interval. The patients were sedated. The derivation study compared invasive PA pressure to mean PA pressure, and derived a regression equation to predict invasive mean from invasive peak pressure. Despite an R2 of >0.9 there was considerable scatter between the mean and peak pressures at the highest levels of pulmonary pressures. A regression equation was derived:
In the validation study, to test if echocardiography Doppler PASP could be substituted to calculate mean pressure, the echocardiograms were done nonsimultaneously with up to one month separating the RHC from the echocardiograms. The result was a stronger correlation (R 2 ≥0.78) between invasive mean PA and noninvasive mean PA than achieved in the Lafitte study. However, inspection of the scatter of data in the plot of individual data points in the validation study suggests that other factors are at play and that an unacceptable number of patients will be misclassified relative to RHC.
Based on these two studies, the use of peak pulmonary pressure as a single parameter by which to describe pulmonary hemodynamics is analogous to using a single dimension to describe left ventricular or left atrial size. It is roughly correct but quite inferior to volumetric information. The time has come to replicate the success of AS evaluation and apply multiple variables to define pulmonary hemodynamics. A suggested multiparameter approach appears below.
The third study in this issue by Bech-Hanssen et al. measured differences in pulmonary arterial pressure and flow characteristics among individuals with normal and increased pulmonary vascular resistance (PVR). This study is based on the observation that the normal pulmonary circulation has low resistance to flow into a highly compliant low pressure system without reflection of the pulmonary pressure wave, while in PHTN with elevated vascular resistance there is a reflected pressure wave that alters the temporal relationship between the peak TR jet and peak PA flow. In PHTN, as the RV pressure increases the relative flow into the pulmonary vasculature decreases. Although not discussed in the paper, the notch in the pulmonary flow signal, an inconstant but specific sign of PHTN, is likely a visual manifestation of the reflected wave when PVR is elevated. Measurements that characterize these changes are the truncated time of pulmonary arterial forward flow (shorter PA acceleration time [PA AcT]), increase in PA pressure after peak flow has been achieved (augmented pressure), and delay from peak flow to peak pressure that develops when PVR is elevated.
The concepts proposed by Bech-Hanssen et al. are valuable in approaching pulmonary hemodynamics independently from the peak TR gradient. The PA AcT may be used as a stand-alone parameter, and the delay to peak pressure and augmented pressure are additional measurements that can be useful to color in the full picture of PVR. The authors evaluated 118 individuals who had echocardiograms and RHCs within 48 hours. The authors also included relevant echocardiographic information on RV area change and tricuspid annular plane systolic excursion. Just as in the other two manuscripts, the authors strove to find a cutoff to detect mean PA pressure >25 mmHg. The authors report different cutoffs for ruling in or ruling out PHTN among the three variables (PA AcT, augmented pressure, and delay to peak pressure).
Bech-Hanssen et al. deserve recognition for their novel approach and attention to full details of the echocardiogram, including estimation of the pulmonary capillary wedge pressure from mitral inflow parameters and PVR from velocity and flow parameters. However, practical measurement of time intervals can be challenging in many laboratories. Even in good hands the measurements do not correlate uniformly with PVR. Simple regression revealed the highest correlation coefficients for augmented pressure (0.73) and delay to peak pressure (0.57) for PVR, but these measurements require multiple time interval measurements and comparison or superimposition of the PA pulsed wave Doppler wave form to or on the TR continuous wave Doppler waveform for pressure measurement. The complexity of these techniques may make uniform adoption challenging, but if confirmed in additional studies could provide an additional tool in interpreting pulmonary hemodynamics.
Studies Reported
Lafitte et al. sought to study the diagnosis of PHTN in a university cardiology department in Bordeaux, France, performing 15,000 transthoracic echocardiographic exams and 4,500 RHCs annually. The study population numbered 310 patients, each of whom had both echocardiography and RHC; a sub-study was performed on 115 subjects in whom the RHC was performed within two days of one another. The retrospective design of the study limited bias in favor of echocardiography in that it was strictly a data base review of reports generated by multiple readers from studies made by fellows, nurse sonographers, and senior clinicians. No contrast was used to enhance TR signals, and TR and inferior vena cava size and collapse were the only right heart Doppler parameters evaluated. Presumably these were the only right heart measurements routinely performed in the laboratory clinical protocol. RHC was performed by “senior physicians” using fluid filled catheters on nonsedated patients. Both peak and mean PASPs were compared with echocardiography for the purpose of this study. It may be noteworthy that there was no control over the independence of the data. That is, some echocardiographers may have known the results of the catheterization when reading, and some performing RHC might have known the results of echocardiograms. Invasive and noninvasive measurements correlated at r = 0.80 with mean difference of 3.6 +/− 15.3 mmHg. Accuracy of correct classification as to whether a patient had PHTN or not was 86% but, importantly, accuracy improved to 89% when the studies were done within two days of one another. Valvulopathy had a nonsignifiant trend toward misclassification with no other disease group significantly associated. The peak systolic pressure that most accurately identified patients who would be classified as PHTN (based on a mean pulmonary pressure of 25 mmHg by RHC) was a Doppler peak PASP of 38 mmHg. However, despite the claims by the authors of high accuracy, sensitivity and specificity, inspection of the plot of the raw data (RHC vs Echo) reveals considerable scatter. For example, if a pulmonary pressure of 40 mmHg is found on echo, the RHC may range from 20 to 60 mmHg. The Bland Altman plot is no more encouraging and variance between the measurements is obvious. In our opinion, these data seem to demand a more comprehensive multiparameter approach to the diagnosis of PHTN and to right heart hemodynamics in general by echocardiography.
Steckelberg et al. , starting with the premise that “mean pulmonary pressure can be difficult to derive with current noninvasive methods,” designed a study to derive a regression equation from which to estimate mean PA pressure from peak systolic pressure measured at RHC. In this retrospective study they evaluated two groups of patients. The first group included 198 randomly selected patients who underwent RHC between 2002–2004, and the second a validation cohort of 109 collected in the first 5 months of 2011 who underwent both RHC and echocardiography up to one month apart. The catheterization protocol was carefully described with the mean PA pressure derived from area under the systolic pressure curve divided by the pulse interval. The patients were sedated. The derivation study compared invasive PA pressure to mean PA pressure, and derived a regression equation to predict invasive mean from invasive peak pressure. Despite an R2 of >0.9 there was considerable scatter between the mean and peak pressures at the highest levels of pulmonary pressures. A regression equation was derived:
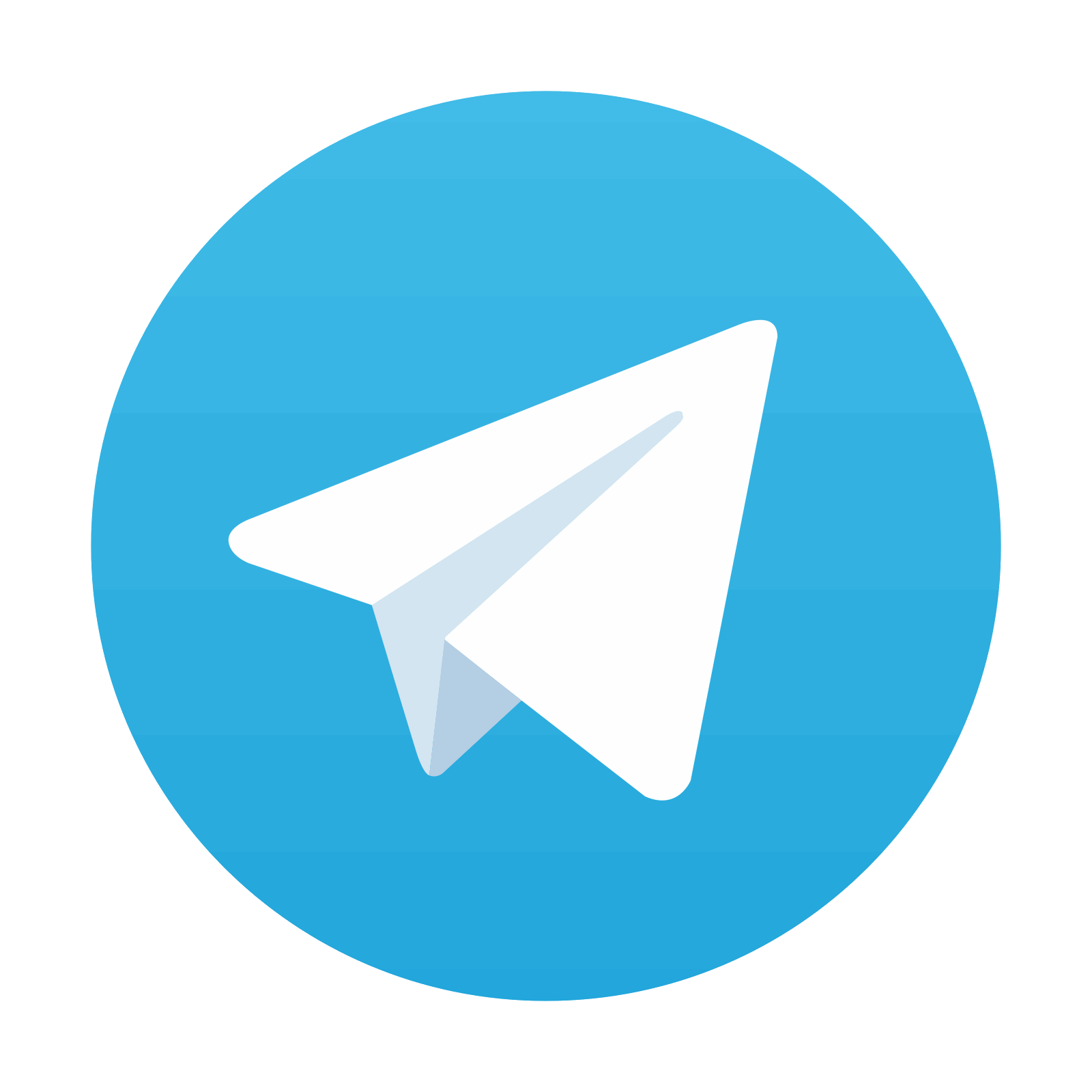
Stay updated, free articles. Join our Telegram channel

Full access? Get Clinical Tree
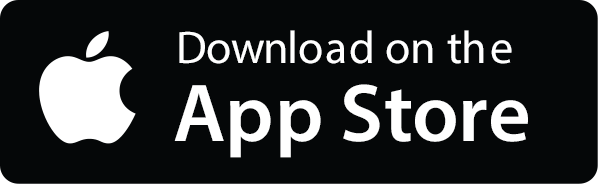
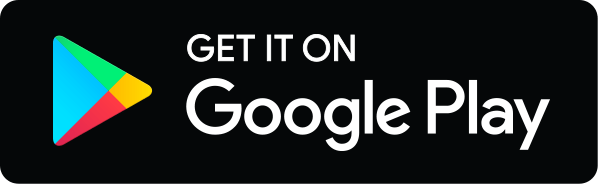