Symptoms
Early preterms (%)
Moderate to late preterms (%)
Full-term born children (%)
Wheeze
34
28
19
Cough
34
33
24
Treatment for respiratory symptoms
21
18
9
Current asthma
9
10
6
Absent from school due to respiratory symptoms
22
21
11
Other studies, however, found that late preterm birth was not associated with a diagnosis of asthma in early childhood [30, 31].
The EPICure study is a good example of considering respiratory symptoms during preadolescence and adolescence in children born extremely preterm. This study defined extremely preterm as <25 completed weeks of gestation. It showed increased respiratory morbidity at 11 years of age, especially among those children who had been diagnosed with BPD [32]. When compared to classmates, children born extremely preterm were more likely to have a current diagnosis of asthma (25 % versus 13 %; P < .01), recent respiratory symptoms, and medication. Among members of the extremely preterm group, significantly more with prior BPD reported wheeze in the past 12 months (see Table 2).
Table 2
Prevalence of respiratory symptoms at the age of 11 years in extremely preterm children [32]
Symptoms | Extremely preterm, with BPD (%) | Extremely preterm, without BPD (%) | Full-term born children (%) |
---|---|---|---|
Wheeze | 25 | 12 | 14 |
Nocturnal cough | 22 | 15 | 11 |
Current asthma | 28 | 19 | 13 |
Asthma medication | 27 | 19 | 11 |
For some former preterm-born adolescents, particularly those who suffered BPD, obstructive lung disease persists into adulthood. Wong et al. reported significantly increased respiratory symptoms in a young adult population born at a time prior to the routine use of surfactant and who had all survived moderate to severe BPD [33]. During adolescence and adulthood, the balance of evidence suggested that preterm infants either with or without subsequent BPD have excess respiratory symptoms, including cough, wheeze, and asthma [34]. Functional pulmonary abnormalities consist of airway obstruction, airway hyperreactivity, and hyperinflation as well as exercise restriction [14, 35]. In these patients, a program of lung function monitoring and pulmonary prophylaxis by means of elimination of specific risk factors, such as smoking, in adulthood is advisable.
Does Gestational Age Matter?
Complications of preterm birth (<37 completed weeks of gestation) are most often seen in very preterm infants (28–31 weeks’ gestation) and extremely preterm infants (<28 weeks’ gestation), although it is increasingly recognized that even moderate to late preterm infants are at increased risk of adverse health and developmental outcomes [36]. This increased risk of respiratory morbidity in late preterm infants is probably related to immature lung structure, since lung development of the terminal respiratory sacs and alveoli continues between gestational weeks 34 and 36.
Infants born most extremely preterm, during the late canalicular or saccular stage of lung development, carry the greatest burden of early respiratory disease, putting them at greatest risk of later pulmonary morbidity. Indeed, despite medical advances in neonatal care that has led to improvements in the survival rate of extremely preterm infants, the prevalence of the neonatal chronic lung disease, BPD, has not diminished [18]. It remains the most common complication of extremely preterm birth. Later in life, the risk of asthma or wheezing disorders increases as the degree of prematurity increases [9]. The risk was considerably higher among children born very preterm, (OR 3.00, 95 % CI 2.61–3.44), when compared to moderately preterm children (OR 1.49, 95 % CI 1.34–1.66) [9].
Which Putative Mechanisms Are to Be Expected?
What is the pathophysiology of respiratory symptoms, such as wheezing disorders, in former preterms? There is the assumption of asthmatics wheeze due to airflow obstruction as a result of the cumulative effects of smooth muscle constriction around airways, airway wall edema, intraluminal mucus accumulation, inflammatory cell infiltration of the submucosa, and basement membrane thickening [37]. Inflammation can cause airway hyperresponsiveness and airflow obstruction.
Is this also the case for preterms? They are often labeled asthmatic, although the underlying mechanisms are likely to be very different. Several mechanisms, such as lung growth, inflammation, and structural changes might play a role in putative mechanisms for wheezing in patients born prematurely.
Lung Growth
The respiratory system undergoes significant growth and development during the third trimester of fetal life and throughout the first year of infancy. Postnatally, the pattern of physiological airway development is best characterized by the global lung initiative (http://www.lungfunction.org/). In healthy children, lung volume and function (FVC and FEV1) continue to increase throughout childhood and reach a plateau at 20 to 25 years [38]. Subsequently, lung volume and function decline steadily with age. In individuals who experienced early lung injury or maldevelopment during infancy, a reduction in peak lung growth may appear [8]. The outcome of poor lung development depends on the type and severity of the insult as well as the developmental stage of the lung at the time it occurred [39]. Longitudinal studies show temporal tracking of small airway diseases among preterm-born individuals [40, 41].
The “new” BPD is often described with pathologic changes of large, simplified alveolar structures, a dysmorphic capillary configuration, and variable interstitial cellularity and/or fibroproliferation [15]. Airway and vascular lesions, when present, tend to be present in infants who develop more severe diseases over time [42]. The concept that “new” BPD results in an arrest in alveolization should be modified to that of an impairment in alveolarization since evidence shows that short ventilatory times and/or the use of nCPAP allow continued alveolar formation [42]. An area of emerging interest in the field of lung imaging is 3He diffusion MRI. With this technique, alveolar damage was studied in survivors of extreme preterm birth by comparing alveolar dimensions between full-term born and preterm-born school children [43]. Alveolar size at school age was similar in survivors of extreme prematurity and full-term born children. Because extreme preterm birth is associated with deranged alveolar structure in infancy, the most likely explanation for this finding is catch-up alveolarization.
Therefore, prematurity per se may have an impact on lung growth, but neonatal events and treatment, for example, supplemental oxygen or mechanical ventilation, may cause inflammatory responses followed by a repair process. The repair process may be “healing” but may also become chronic in response to continued inflammation, resulting in structural changes in the airways that are referred to as remodeling. These structural changes may result in irreversible narrowing of the airways. Over time, in most children, with or without BPD, pulmonary function improves. Whether this improvement represents repair of damaged lung tissue or growth of new lung tissue, or both, has yet to be determined.
Children and adolescents with severe BPD, however, show evidence of chronic obstructive pulmonary disease. Kotecha et al. systematically reviewed the literature to determine whether the percentage predicted forced expiratory volume during 1 s (%FEV1) is lower in preterm-born subjects, with or without BPD, in comparison to full-term born controls. They found that %FEV1 is decreased in preterm-born survivors, even in individuals who did not develop BPD. For the preterm-born group without BPD, the mean difference %FEV1 in comparison to full-term born controls is −7.2 %, and for the BPD groups, it was −16.2 % to −18.9 %, respectively (according to the definition of BPD) [44].
Inflammation
Former preterm-born children show little evidence of eosinophilic inflammation [45]. Exhaled nitric oxide concentrations are significantly lower in BPD survivors than in asthmatic cases, suggesting that different pathogenetic mechanisms characterize these two chronic obstructive lung diseases [46].
When inflammation plays a role, treatment with inhaled corticosteroids might be effective. A recent systematic review studied whether inhaled bronchodilators and inhaled corticosteroids improve long-term outcomes in neonates with BPD. No meta-analysis was attempted due to the large degree of heterogeneity and quality assessment of the studies included. The conclusion was that although these inhaled therapies seem to have some benefit, very limited data are available suggesting that these treatments at neonatal age improve long-term outcomes of infants with BPD [47].
Kotecha et al. systematically reviewed the evidence for bronchodilator treatment in former preterm-born children and adults. They concluded that the majority of the studies reported short-term effects of a single-dose administration with an improvement in %FEV1 after bronchodilator treatment. There is, however, a paucity of data on the effect of longer term administration of bronchodilators on the lung function of former preterm-born children [48]. The number of studies with inhaled corticosteroids is small in older preterm-born children and these studies show no effect [49]. There is no current evidence to advocate widespread use of bronchodilators or inhaled corticosteroids, even though a component of variable airflow obstruction may be present. Additional evidence for optimal treatment is required [50].
Acute inflammation due to respiratory tract infections, such as respiratory syncytial virus (RSV) infection, could be an important mechanism of recurrent wheeze during the first year of life in preterm-born infants [51]. A connection between viral infections and exaggerated cell death and inflammatory pathways in the developing lung was recently revealed [52].
Structural Changes
In contrast to early lung development, a process exemplified by the branching of the developing airways, the later development of the immature lung remains poorly understood. A key event in late lung development is secondary septation, in which secondary septa arise from primary septa, creating a greater number of alveoli of a smaller size. This phase in lung development dramatically expands the surface area over which gas exchange can take place [52]. Secondary septation, together with architectural changes to the vascular structure of the lung that minimize the distance between inspired air and blood, is the objective of late lung development. When late lung development is disturbed, lung architecture is malformed. Depending on the severity of the architectural malformation, there may be serious consequences in terms of respiratory function, as well as long-term consequences in later life [52]. It is not clear whether early preterm lung injury is associated with structural damage to surrounding lung tissue because it is difficult to obtain histological tissue for study purposes. Nevertheless, a crucial mechanism that secures airway patency and thus adequate maintenance of functional residual capacity (FRC) is airway tethering [53–55]. Tethering is the element that couples lung volume to airway patency. Thus, as lung volumes increase, airway diameter and hence expiratory flows increase. Maturation of the alveolar network improves parenchymal elastance and, as a consequence, airway tethering. Immaturity adds up to the elements constituting the vulnerability of preterm infants [53].
Radiologic studies in older and more severe cases with BPD showed structural changes such as emphysema on high-resolution CT scans [33, 56, 57]. There seems to be an association between the extent of radiological abnormality on high-resolution CT scans and the severity of lung function impairment [33].
Which Risk Factors Are Described for Respiratory Problems in Former Preterm-Born Children?
On the one hand, there are several factors that may cause a preterm birth and that, additionally, are likely to affect fetal lung development and adult outcome. On the other hand, there are several postnatal factors that affect the risk of continuing respiratory problems in a former preterm-born child.
Preterm delivery is the most common cause of abnormal lung development and can itself lead to lifelong sequelae. As described above, compared to full-term born children, children born very preterm (before 32 weeks’ gestation) approximately run a three times higher risk of developing asthma and/or wheezing disorders in unadjusted and adjusted analyses [9].
Factors that may result in poor lung development prenatally include inadequate nutrition or specific nutrient deficiencies, maternal alcohol consumption, exposure to tobacco smoke, chorioamnionitis, and intrauterine infection. Postnatal factors include being ventilated with high oxygen concentrations, BPD, respiratory infections, and exposure to environmental pollution [8, 52]. Hypoxia also represents a potent stimulus that has a negative impact on late lung development. The impact of corticosteroids on lung development is complicated, since the positive anti-inflammatory and lung maturation impact of corticosteroids is counterbalanced by growth retardation and other effects on the lung [52].
With regard to the respiratory tract infections, “the chicken or the egg” debate is ongoing. Human RSV is the most common cause of severe lower respiratory tract illnesses in both preterm and full-term newborns and young children, and it is associated with subsequent recurrent wheeze. Observational studies cannot determine whether RSV infection is the cause of recurrent wheeze or the first indication of preexistent pulmonary vulnerability in preterm infants. Several studies showed increased susceptibility of preterm-born children (with and without BPD) to RSV [58]. In the Lollipop study, the rates of hospitalization due to proven RSV infection are higher in both preterm groups than in full terms. No difference in disease severity was observed. Among moderate–late preterms, the rate of RSV hospitalization was higher for lower gestational ages and if the infants had been exposed to passive smoking [59].
Nonrandomized trials in preterm infants (approximately 30 ± 2 weeks’ gestational age) suggested that the prevention of lower respiratory tract illness caused by RSV reduces subsequent recurrent wheeze in infants without a family history of atopy, while no effect was found in infants with a family history of atopy [60, 61]. In another study, in otherwise healthy 33–35 weeks’ gestational age preterm infants, palivizumab treatment resulted in a significant reduction in wheezing days during the first year of life, even after treatment had stopped. These findings might implicate RSV infection as an important mechanism of recurrent wheeze during the first year of life in such infants [51]. Other risk factors for continuing respiratory problems in moderate to late preterm children were found to be eczema during the first year of life, passive smoking during the first year, higher social class, and a positive family history of asthma [29].
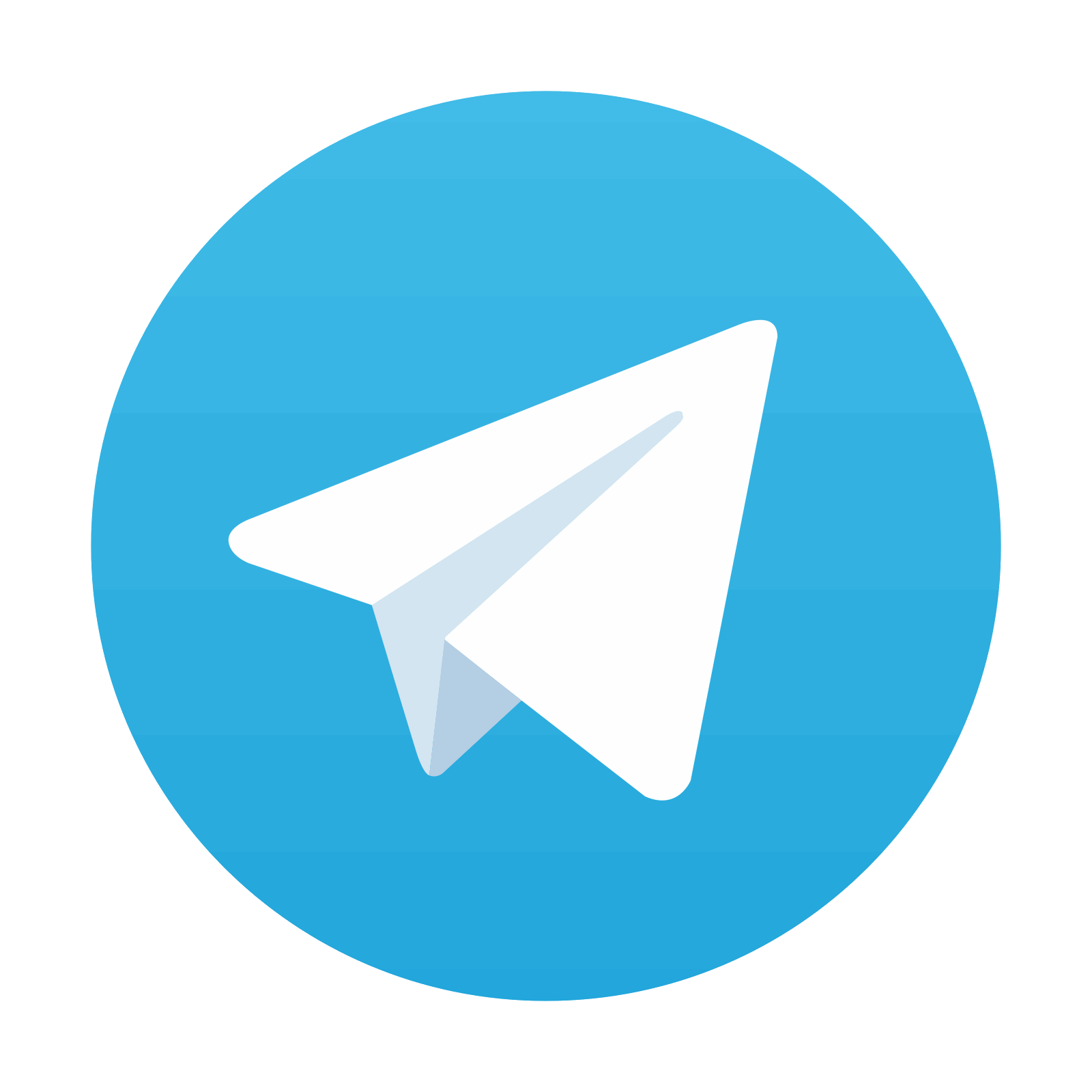
Stay updated, free articles. Join our Telegram channel

Full access? Get Clinical Tree
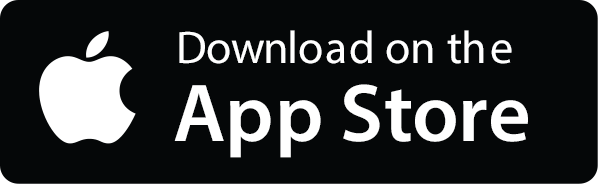
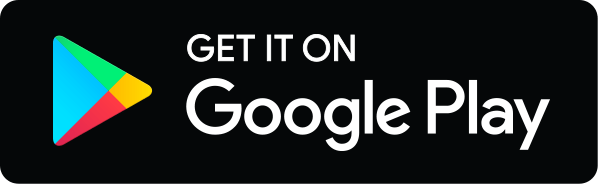