Diseases of the Pulmonary Vasculature
Marlene Rabinovitch
Overview
This chapter addresses the structural basis and functional sequelae of diseases of the pulmonary vasculature. The embryology of the pulmonary arterial tree is first presented and the genetic basis of developmental aberrations discussed. Perturbations in pulmonary vascular development in utero associated with lesions such as pulmonary atresia and ventricular septal defect (VSD), absence of the pulmonary valve, or diaphragmatic hernia lead to major difficulties in clinical management. Even mild structural changes associated with the altered pulmonary hemodynamics observed in congenital heart defects are of major concern in the correction of complex lesions with single-ventricle physiology.
A variety of conditions can lead to the development of pulmonary arterial hypertension (PAH) in the infant and adult. The pathophysiology of these conditions is described, with a focus on aberrant vascular cell–extracellular matrix interactions. The current approach to diagnosis and treatment is also addressed, primarily with a focus on alleviating the primary condition with which PAH is associated. Idiopathic pulmonary hypertension (IPAH) is then considered. Knowledge of the genetic abnormalities that underlie this disease is providing new insights into pathophysiology and future approaches to treatment. There has also been a substantial change in the armamentarium of therapeutic options, beyond intravenous prostacyclin.
Development of the Pulmonary Vasculature in Embryonic and Fetal Life
Macroscopic Features
In the fifth-week human embryo, primitive pulmonary vessels from the sixth aortic arch supply theupper “poles” of the right and left lung, whereas the lower poles are supplied by a pair of intersegmental arteries arising from the dorsal aorta and penetrating upward through the diaphragm. The developing lung parenchymal blood vessels are largely localized to the interlobular septae. Between the fifth and eighth weeks of gestation, numerous paired dorsal intersegmental arteries supply the emerging blood vessels that are developing alongside the branching bronchi in the lung parenchyma. As the true central pulmonary arteries form and anastomose with the intrapulmonary arteries, the primitive pulmonary arteries arising from the aorta, as well as the primitive intersegmental arteries, involute. Formation of the bronchial system occurs only at the ninth week of gestation. With each airway generation, there is an accompanying artery in addition to numerous supernumerary arteries. By the sixteenth week of gestation, the number of preacinar airways and accompanying arteries is complete. Thereafter, as the acini (terminal bronchioli, respiratory bronchioli, alveolar ducts, and alveoli) develop, so do the accompanying and
supernumerary arteries as reviewed by Rabinovitch (1) and Hislop and Reid (2).
supernumerary arteries as reviewed by Rabinovitch (1) and Hislop and Reid (2).
Abnormal maturation or maturational arrest in pulmonary arterial development is reflected in functional derangement, which can present in the newborn period. Persistent pulmonary hypertension of the newborn has been reported in association with pulmonary arterial maturational arrest at the fifth week of gestation (3). Infants with pulmonary atresia and VSD frequently have persistence of the intersegmental arteries (aortopulmonary collaterals) (Fig. 104.1) (4). These vessels can serve as the sole source of blood supply to a lobe or lobar segment; alternatively, they can form a dual circulation with intersegmental arteries anastomosing to arteries that can be traced back to a central pulmonary artery origin. There also can be multiple “indirect” aortopulmonary collaterals arising from aortic branches (e.g., from subclavian, intercostals, coronary arteries). These vessels are observed in the absence of direct collaterals, which suggests that they develop as a consequence of pulmonary atresia when it occurs later in development. Finally, in some cases of pulmonary atresia, there is supply by anastomotic vessels that arise from true bronchial arteries that develop after the ninth week of gestation.
A striking pulmonary vascular abnormality is observed with absence of the pulmonary valve (5). In patients who present with severe respiratory problems from birth, we have observed abnormal branching morphogenesis. Tufts of intersegmental pulmonary arteries are seen arising in “weeping willow” or “squid-like” fashion, encircling and compressing the intrapulmonary airways (Fig. 104.2).
Microscopic Features
In the fetus, the preacinar arteries and those at the terminal bronchiolus level are muscular, whereas the intraacinar arteries (i.e., those accompanying respiratory bronchioli) are partially muscular (surrounded by a spiral of muscle) or nonmuscular. Arteries at alveolar duct and wall levels are nonmuscular. The preacinar arteries are thick walled, and wall thickness changes little relative to external diameter throughout the fetal period.
Experimental studies (6) suggest that the immediate postnatal period is characterized by rapid recruitment of small alveolar duct and wall vessels, which appear to be functionally and structurally closed in the prenatal period. Progressive dilation of muscular arteries also occurs—that is, the smallest muscular arteries (<250 μm) dilate, and their walls thin to adult levels within a few days; by the age of 4 months, this process includes the largest pulmonary arteries at the hilum. As the external diameter of the intraacinar arteries at the various airway levels increases with age, muscle appears to extend peripherally, in that it is observed in arteries located more distally within the acinus. Nonmuscular arteries become partially muscular, and later they become fully muscularized. For example, in infancy, vessels at the alveolar duct level are still largely nonmuscular, but in childhood they become partially muscularized, and in the adult they are fully muscularized (7). Alveolar wall arteries remain largely nonmuscular even in the adult. The muscularization of these peripheral pulmonary arteries may be related to the differentiation of pericytes as well as to the recruitment of fibroblasts (8,9).
Experimental studies (6) suggest that the immediate postnatal period is characterized by rapid recruitment of small alveolar duct and wall vessels, which appear to be functionally and structurally closed in the prenatal period. Progressive dilation of muscular arteries also occurs—that is, the smallest muscular arteries (<250 μm) dilate, and their walls thin to adult levels within a few days; by the age of 4 months, this process includes the largest pulmonary arteries at the hilum. As the external diameter of the intraacinar arteries at the various airway levels increases with age, muscle appears to extend peripherally, in that it is observed in arteries located more distally within the acinus. Nonmuscular arteries become partially muscular, and later they become fully muscularized. For example, in infancy, vessels at the alveolar duct level are still largely nonmuscular, but in childhood they become partially muscularized, and in the adult they are fully muscularized (7). Alveolar wall arteries remain largely nonmuscular even in the adult. The muscularization of these peripheral pulmonary arteries may be related to the differentiation of pericytes as well as to the recruitment of fibroblasts (8,9).
Precapillary (alveolar duct and alveolar wall) arteries proliferate through the neonatal period and early infancy, accompanying the proliferation of alveoli; the ratio of alveoli to arteries can therefore be used as a measure of numerical arterial growth. The ratio of alveoli to arteries actually decreases from the newborn value of 20:1 to the value of 6:1 to 8:1, which is achieved first in early childhood and persists (Fig. 104.3). The growth and development of the pulmonary circulation are also likely influenced by the trophic effects of neuropeptides (10) released from nerve endings as well as from neuroendocrine bodies (11) associated with accompanying airways.
Experimental studies indicate that the normal adaptation to postnatal life is regulated by remodeling of the smooth muscle cell actin cytoskeleton (12), changes in the production and distribution of connective tissue, especially elastin and collagen (13,14), and consequent cellular and cytoskeletal rearrangement (15) that likely involves the Rho kinase pathway (16,17). It also has been shown experimentally that there are smooth muscle cells with differing proliferative potential (18,19). For example, metavinculin-positive smooth muscle cells are relatively resistant to proliferation, and the variability in proliferative potential reflects differences in activation of protein kinase C intracellular signaling pathways (20).
Cellular Mechanisms of Vascular Development
Studies by several groups [reviewed by Baldwin (21)] address specific cellular and molecular mechanisms that might regulate fetal growth and development of the pulmonary vasculature. These mechanisms include expression of cell adhesion molecules, such as vascular cell adhesion molecule 1 (22) and platelet endothelial cell adhesion molecule (23), in addition to the β1 (24) and β3 families of integrin receptors, especially αvβ3 (25). The β1 family of integrins binds fibronectin, and the β3 family also binds fibronectin as well as a host of matrix molecules, especially tenascin-C, osteopontin, and vitronectin, some of which have been shown to govern vascular cell migration (e.g., fibronectin) (26,27,28,29) or proliferation (e.g., tenascin-C) (30). Because these molecules mediate direct interactions between vascular cells and the extracellular matrix, perturbations can be expected to lead to malformation of the pulmonary and systemic arteries. Tenascin-C is regulated by the transcription factor Prx-1, and this factor is critical for endothelial cell growth and differentiation in the lung leading to angiogenesis and vasculogenesis (31). A variety of growth factors, including vascular endothelial growth factor (VEGF) (32) and acidic and basic fibroblast growth factors (FGF-1 and FGF-2) (33), are also responsible for the orderly growth and branching morphogenesis of blood vessels. These factors are regulated through their interaction with specific cell-surface tyrosine kinase receptors, such as flt-1 and flk-1, tek, and tie (34,35,36). The balance between proteases and antiproteinases also controls growth factor interaction with cell-surface molecules. Plasmin, thrombin (37,38), and elastases, including leukocyte and endogenous vascular elastase (39), release growth factors from storage sites in the extracellular matrix in an active form. In addition, a variety of endogenously expressed inhibitors of proteinases and elastases, such as plasminogen activator inhibitor (40) and elafin (41
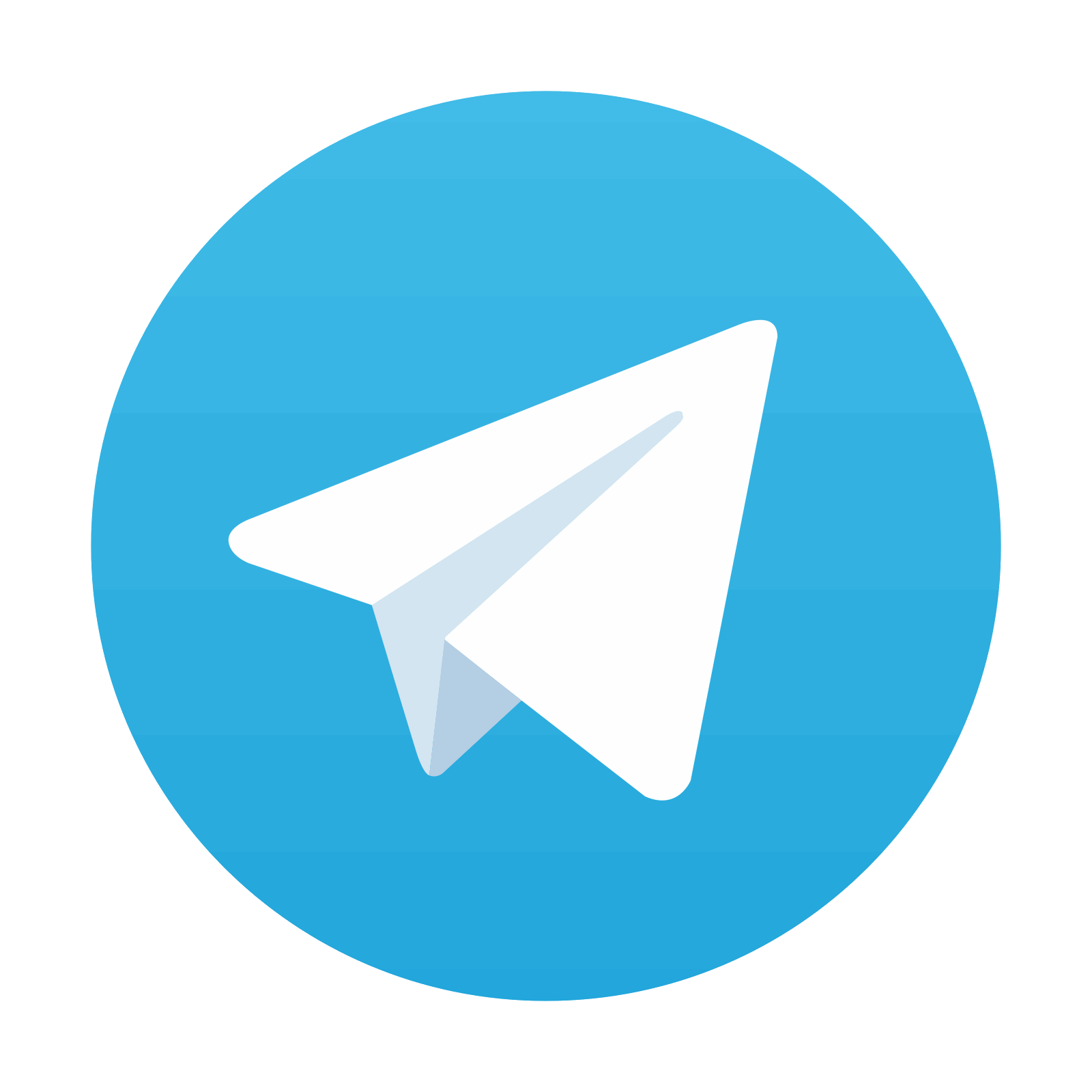
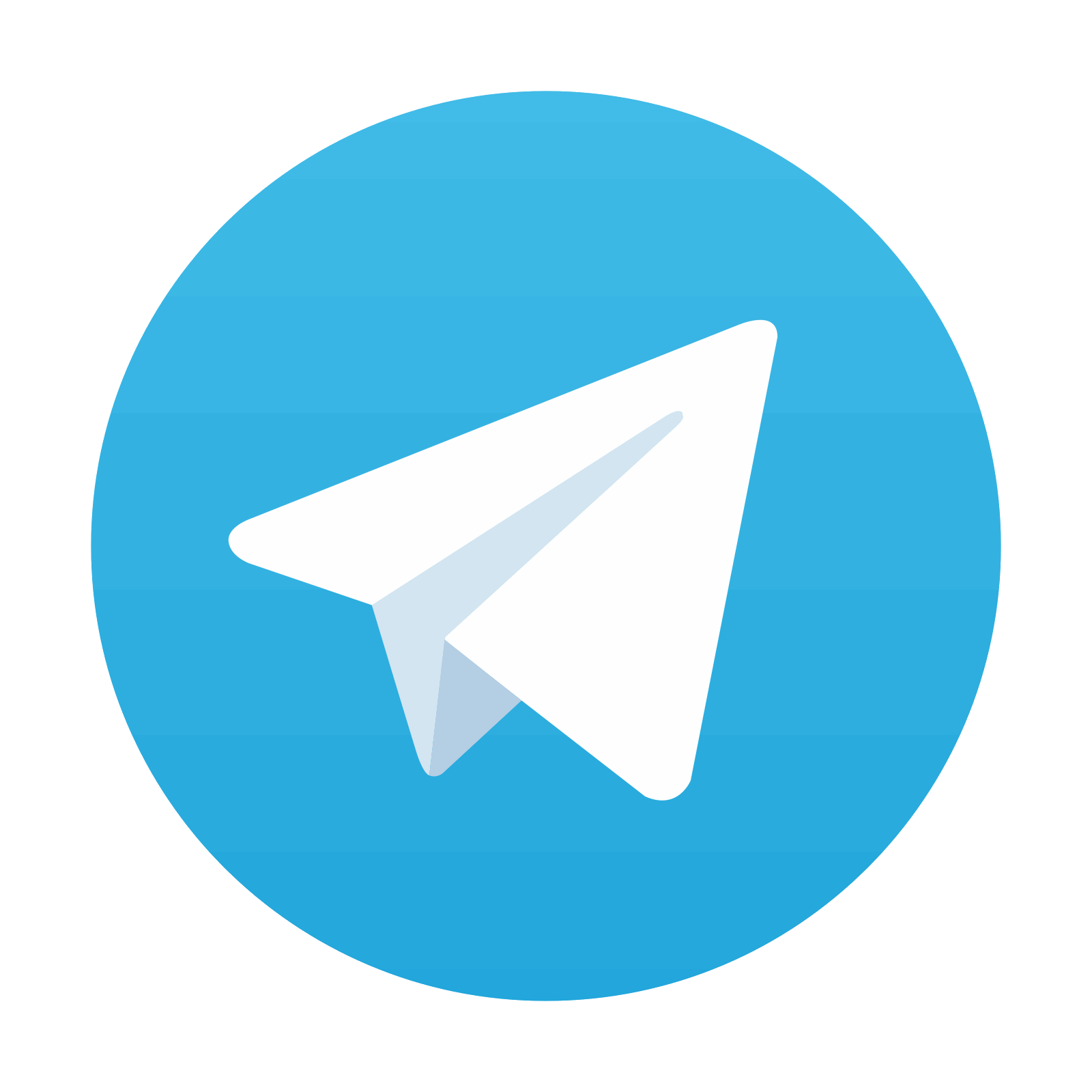
Stay updated, free articles. Join our Telegram channel

Full access? Get Clinical Tree
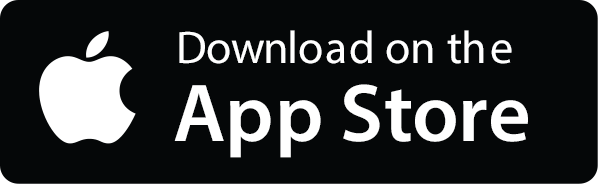
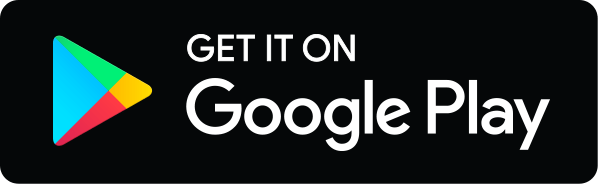