Background
Speckle-tracking imaging is a novel method for assessing left ventricular (LV) function and ischemic changes. The aim of this study was to assess the predictive value of two-dimensional longitudinal strain in the detection of longitudinal LV dysfunction and the identification of coronary artery disease (CAD) in patients hospitalized with angina.
Methods
Two-dimensional strain software was extended to allow the analysis of numerous longitudinal strain traces in the entire left ventricle and generate a histogram of peak systolic strain (PSS) values for the left ventricle and for each coronary territory. In each histogram, the value of the 10% worst strain values (PSS 10% ) was determined. Global strain, segmental PSS, and PSS 10% were analyzed in 97 patients hospitalized with angina and had normal LV function, who underwent coronary angiography, and 51 patients with low probability of CAD. Echocardiography was performed 2.9 ± 2 days after admission.
Results
Sixty-nine patients had significant CAD on coronary angiography. Significant differences were observed in all strain parameters between patients with and without CAD. PSS 10% showed the best accuracy in detecting CAD, with an area under the receiver operating characteristic curve of 0.85. The areas under the curve for global strain and segmental PSS were 0.80 and 0.76, respectively. The optimal cutoff for PSS 10% was −13.9%, with sensitivity and specificity of 86% and 75%, respectively. PSS 10% was better than segmental PSS in the detection of CAD in each coronary territory.
Conclusions
In patients hospitalized with angina who have significant CAD on coronary angiography, longitudinal systolic function is impaired. Histogram analysis improved the accuracy of longitudinal strain analysis in detecting global and regional impaired function.
Chest pain, a potential manifestation of coronary artery disease (CAD), is the second most common complaint of patients presenting to emergency departments. Several studies have demonstrated the ability of echocardiographic assessment of wall thickening to provide diagnostic and prognostic information in patients with acute chest pain. However, the accuracy of standard echocardiography decreases the more remote the episode of chest pain is and is lower in patients with no acute or previous myocardial infarction.
The introduction of ultrasonic speckle-tracking imaging has stimulated a multitude of clinical studies in an effort to quantify left ventricular (LV) mechanical function. Preliminary studies suggested that significant coronary stenosis may cause persistently impaired longitudinal function at rest, which can be detected by myocardial strain imaging. This effect can also be observed in patients with visually normal LV function. The populations studied were mainly patients with risk factors for CAD or patients with stable angina. The diagnosis of CAD in these studies was based on either segmental or global analysis, and various speckle-tracking imaging parameters were measured. However, standard segmental analysis may be limited, mainly because the impaired area is not necessarily aligned with the standard segmental division of the left ventricle. Global assessment, on the other hand, is less sensitive and not territory specific. We hypothesized that neither segmental nor global strain (GS) assessments fully use the information provided by the speckle-tracking imaging modality and are thus suboptimal in the assessment of LV function. We further hypothesized that detailed distribution analysis (histogram) of all longitudinal peak systolic strain (PSS) values provided by the two-dimensional (2D) strain method would result in a better and more precise assessment of longitudinal function, leading to a more accurate diagnosis of CAD.
In the present study, we assessed the diagnostic value of 2D longitudinal strain using global and segmental analysis as well as a novel method of generating histograms of LV strain distribution for the diagnosis of CAD in patients presenting with chest pain.
Methods
The study included 97 consecutive patients hospitalized with chest pain and suspected CAD during the study period and a control group of 51 patients with low probability of CAD. The patient group included patients who were hospitalized for chest pain and who underwent both echocardiography and coronary angiography and also met the following inclusion criteria: (1) echocardiography performed before angiography, (2) normal global (LV ejection fraction > 50%) and regional LV systolic function, (3) sinus rhythm without conduction abnormalities, and (4) good image quality and acquisition frame rates > 45 frames/sec. Patients with ST-segment elevation myocardial infarction at presentation or history of myocardial infarction, significant valvular disease, perimyocarditis, or cardiomyopathy were excluded. In 15 patients, strain analysis was technically not feasible, and these patients were not included in the study. The control group included 51 patients referred to stress echocardiography for the evaluation of atypical chest pain or before physical activity. These patients had a low probability of CAD, defined as no risk factors and normal results on stress echocardiography, and they did not undergo coronary angiography. The control group was used for comparison with patients with no CAD on coronary angiography.
Patients were assessed clinically at presentation. Acute coronary syndrome was defined as rest pain or crescendo angina with or without electrocardiographic changes or troponin level elevation. Stable angina was defined as effort pain or nonspecific chest pain with positive stress test results. All these patients underwent coronary angiography, and patients were defined as having CAD when there was ≥50% stenosis in one or more coronary arteries. All others were defined as having no CAD.
The study was approved by the institutional review board of Kaplan Medical Center.
Echocardiographic Examination
Patients were scanned in the left lateral decubitus position using a commercially available system (Vivid 7; GE Vingmed Ultrasound AS, Horten, Norway). Two-dimensional and Doppler images were obtained using a 3.5-MHz transducer from the standard views. LV function was assessed visually, and LV ejection fraction was calculated using the biplane Simpson’s method.
LV Deformation Analysis
Strain analysis was done offline by one investigator unaware of angiographic results using 2D strain software (EchoPAC SW version 6.0.0; GE Vingmed Ultrasound AS). Strain was first analyzed in the apical long-axis view, with closure of the aortic valve defined visually. The time interval between the R wave and aortic valve closure was measured and used as a reference for longitudinal strain assessment in all three apical views. After defining the mitral annulus and the LV apex with three points at the end-systolic frame in each apical view, the automated algorithm traced the endocardial border and built the region of interest covering the entire myocardial wall. The tracking algorithm followed LV wall motion throughout the cardiac cycle and calculated deformation parameters. The left ventricle was divided into six segments of equal length in each view, and tracking quality was automatically assessed per segment. The region of interest was occasionally manually adjusted to improve tracking.
Segmental Analysis
The longitudinal strain traces in each segment were averaged to a single segmental strain trace. Segmental longitudinal PSS was then computed as that of the segment with the smallest (least negative) longitudinal strain during systole. For the purpose of comparison with coronary angiography, we used the conventional segmental division for the three main coronary territories.
GS Analysis
Peak systolic longitudinal strain was calculated for the entire U-shaped length of the LV myocardium (basal, mid, and apical segments of two opposite walls) in each view, as previously described, and averaged out to a single value of LV global longitudinal strain.
Histographic Analysis
In histogram analysis, the region of interest is divided into 50 to 70 elements of 1 to 2 mm in length (knots) in each view. The 2D strain software generates longitudinal strain traces of all 150 to 200 knots in the three views. Proprietary code was written and used for further processing. In the framework of this processing, all corresponding PSS values were computed ( Figure 1 ). For each patient, four histograms were generated. One histogram described the PSS distribution in the entire left ventricle, while the other three represented the distribution in the three main coronary territories. For each histogram, we calculated the lowest (least negative) 10th-percentile PSS (PSS 10% ) value, which separated the 90% most negative and the 10% least negative PSS values, and used it as a value that characterized the “abnormality” of the strain distribution. Figure 1 shows an example of a histogram and PSS 10% calculations for patients with and without CAD.

Coronary Angiography
Coronary angiography was performed in multiple projections using the standard Judkins technique. Angiographic findings were assessed qualitatively by an experienced interventional cardiologist who was unaware of patients’ clinical and echocardiographic results.
Statistical Analysis
Statistical analysis was performed using SAS version 9.2 (SAS Institute Inc., Cary NC). Descriptive statistics are presented for demographic data and risk factor data. Categorical variables are expressed as counts and percentages and continuous data as mean ± SD. The demographic and risk factor data of the two groups of patients (CAD and no CAD) were compared using t tests for continuous variables and χ 2 tests for categorical data.
Diagnostic accuracy was assessed by receiver operating characteristic (ROC) curves, which were summarized by the area under the ROC curve (AUC). AUCs were compared using the method of DeLong, DeLong, and Clarke-Pearson. The sensitivity and specificity of optimal cutoff points on the ROC curves were calculated.
The distribution of PSS 10% values in patients with low probability of CAD and patients with no CAD on coronary angiography was analyzed using the Kolmogorov-Smirnov test for equality of distribution.
The correlation between strain values and acquisition frame rates was assessed using Pearson’s correlation test. The comparison between PSS 10% values acquired at frame rates <70 frames/sec and those acquired at frame rates ≥70 frames/sec in patients with no CAD was performed using the t test.
A stepwise multivariate logistic regression model was used to predict strain values.
The reproducibility of GS, segmental PSS (SPSS), and PSS 10% measurements was analyzed with repeated measurements, in a blinded fashion, by one experienced observer at two different time points and by a second experienced observer in 20 randomly selected patients. Intraobserver and interobserver agreement for each parameter were evaluated by Bland-Altman analysis. All statistical tests were two sided and tested at the 5% level of significance.
Results
Clinical Characteristics
The study included 97 patients meeting the inclusion criteria who underwent coronary angiography and a control group of 51 patients with low probability of CAD who did not undergo coronary angiography. Thirty-five patients of the patient group (36%) had acute coronary syndromes, and 62 patients (64%) had stable angina by the above-defined criteria. The majority of patients (85%) did not have electrocardiographic changes on admission. Thirteen patients in the group with acute coronary syndromes had elevated troponin within 12 hours of hospitalization. The mean troponin value was 0.98 ± 0.8 μg/L. Sixty-nine patients had significant coronary artery stenosis, and 28 patients had no CAD on coronary angiography. Thirty-one patients had singe-vessel CAD, 20 patients had two-vessel CAD, and 18 patients had multivessel CAD. Thirty-two of the 35 patients defined clinically as having acute coronary syndromes had CAD on angiography. Clinical characteristics of the patients are shown in Table 1 . There were no significant differences in baseline characteristics such as age, gender, risk factors, and prior revascularization between patients with and without CAD on coronary angiography. Heart rate, blood pressure, and β-blocker and angiotensin-converting enzyme inhibitor treatment during hospitalization were not significantly different between the two patient groups. Patients in the control group, with low probability of CAD, were younger and had no risk factors.
Variable | CAD ( n = 69) | Normal ( n = 28) | Low probability of CAD | P value, CAD vs no CAD | P value, no CAD vs low probability of CAD |
---|---|---|---|---|---|
Age (y) | 62.8 ± 10 | 62.5 ± 11 | 54.5 ± 14 | .40 | .005 |
Men (%) | 55 (80%) | 19 (68%) | 29 (57%) | .26 | .47 |
Risk factors | |||||
Diabetes | 18 (26%) | 4 (14%) | 0 | .32 | |
Hypertension | 39 (56%) | 18 (64%) | 0 | .63 | |
Dyslipidemia | 51 (74%) | 15 (54%) | 0 | .09 | |
Smoking | 23 (33%) | 5 (18%) | 0 | .20 | |
Family history of CAD | 15 (22%) | 6 (21%) | 0 | .80 | |
Previous revascularization | 16 (23%) | 5 (18%) | 0 | .76 | |
Pulse (beats/min) | 65 ± 3 | 66 ± 4 | 67 ± 4 | .46 | .51 |
Systolic blood pressure (mm Hg) | 126 ± 7 | 120 ± 8 | 110 ± 6 | .06 | .02 |
β-blockers | 45 (66%) | 18 (64%) | 0 | .89 | |
Angiotensin-converting enzyme inhibitors | 24 (35%) | 8 (2%) | 0 | .84 |
Baseline Echocardiographic Characteristics
Echocardiography was performed a mean of 2.9 ± 2 days after admission and 1.3 ± 0.5 days before coronary angiography. The baseline echocardiographic parameters are presented in Table 2 . There were no differences in LV size, mass, and systolic function between patients with and without CAD on coronary angiography. There were no significant differences in diastolic functional parameters between the groups. Patients in the control group had lower myocardial thickness and higher mitral E-wave velocities compared with those with no CAD on coronary angiography.
Variable | CAD ( n = 69) | Normal ( n = 28) | Low probability of CAD | P value | P value, no CAD vs low probability of CAD |
---|---|---|---|---|---|
LVDD (mm) | 47.3 ± 6.6 | 46.5 ± 3.9 | 46.5 ± 4.2 | .20 | .48 |
LVSD (mm) | 28.6 ± 5.0 | 27.6 ± 4.4 | 28.1 ± 4.1 | .20 | .34 |
Septum (mm) | 11.3 ± 2.2 | 11.0 ± 1.5 | 9.9 ± 1.0 | .18 | .04 |
LVEF (%) | 57.9 ± 2.5 | 58.0 ± 3.1 | 58.9 ± 2.08 | .30 | .09 |
Mitral E velocity (cm/sec) | 73.4 ± 18.9 | 66.7 ± 13 | 74.7 ± 18 | .06 | .03 |
Mitral E/A ratio | 1.1 ± 0.9 | 0.97 ± 0.26 | 1.09 ± 0.4 | .20 | .08 |
E-wave deceleration time (msec) | 238 ± 62 | 241 ± 78 | 217.2 ± 54.9 | .40 | .08 |
IVRT (msec) | 93.2 | 96.8 | 2.28 ± 0.2 | .20 | .10 |
TR velocity (m/sec) | 2.25 ± 0.35 | 2.3 ± 0.27 | 2.28 ± 0.2 | .40 | .10 |
Longitudinal Strain
GS, SPSS, and PSS 10% values in patients with and without CAD are presented in Table 3 . There were significant differences in all strain parameters between patients with and those without CAD. Patients with CAD had significantly lower longitudinal strain values compared with patients with no CAD. There were no differences in GS, SPSS, and PSS 10% values between patients with no CAD on coronary angiography and those with low probability of CAD. The distribution of PSS 10% values in patients with no CAD on coronary angiography and the control group of patients with low probability of CAD is shown in Figure 2 . The values of PSS 10% showed similar distributions ( P = .54) in both populations.
Variable | CAD ( n = 69) | Normal ( n = 28) | Low probability of CAD | P value, CAD vs no CAD | P value, no CAD vs low probability of CAD |
---|---|---|---|---|---|
GS | −17.3% ± 2.4 | −20.8% ± 2.3 | −21.4% ± 2 | <.001 | .11 |
Segmental analysis | −9.1% ± 3.2 | −12.9% ± 3.3 | −13.8% ± 2.1 | <.001 | .11 |
Histographic analysis | −9.9% ± 3.3 | −14.6% ± 2.3 | −15.2% ± 2.3 | <.001 | .12 |
A multivariate stepwise logistic regression model that included baseline echocardiographic variables (LV volume and thickness, mitral inflow E wave) and the presence of CAD on coronary angiography determined that CAD was the only independent predictor of PSS 10% values ( P = .03).
There was no correlation between PSS 10% values and acquisition frame rates ( r = 0.02, P = .80). In patients with no CAD or low probability of CAD, no significant differences were found between strain values acquisition frame rates <70 frames/sec and those acquisition frame rates ≥70 frames/sec ( P = .12).
ROC Curves for Cutoff Values and Accuracy
Figure 3 illustrates the results of the ROC analysis for GS, SPSS, and PSS 10% in the detection of CAD. The AUC for PSS 10% was significantly higher than the AUC for SPSS (0.86 and 0.76, respectively; P = .004). The AUC for GS was 0.8. There was no statistically significant difference between the AUC for PSS 10% and that for GS (0.86 and 0.8, respectively; P = .13). The optimal cutoffs and the accuracy of GS, SPSS, and PSS 10% to detect coronary stenosis are shown in Table 4 . The best accuracy for detecting CAD was seen with PSS 10% , with sensitivity of 86% and specificity of 75%. The sensitivity and specificity of GS was 81% and 67%, respectively. The values for SPSS were 77% and 68%, respectively.
Variable | AUC | Cutoff value | Sensitivity (%) | Specificity (%) |
---|---|---|---|---|
GS | 0.8 | −19.7% | 81 | 67 |
Acute coronary syndrome | 0.86 | 86 | 71 | |
Troponin positive | 0.84 | 92 | 71 | |
Stable angina | 0.75 | 76 | 68 | |
Segmental analysis | 0.76 | −12.6% | 77 | 68 |
Acute coronary syndrome | 0.81 | 82 | 64 | |
Troponin positive | 0.8 | 85 | 64 | |
Stable angina | 0.72 | 76 | 68 | |
Histographic analysis | 0.85 | −13.9% | 86 | 75 |
Acute coronary syndrome | 0.91 | 93 | 75 | |
Troponin positive | 0.91 | 100 | 75 | |
Stable angina | 0.82 | 80 | 75 |
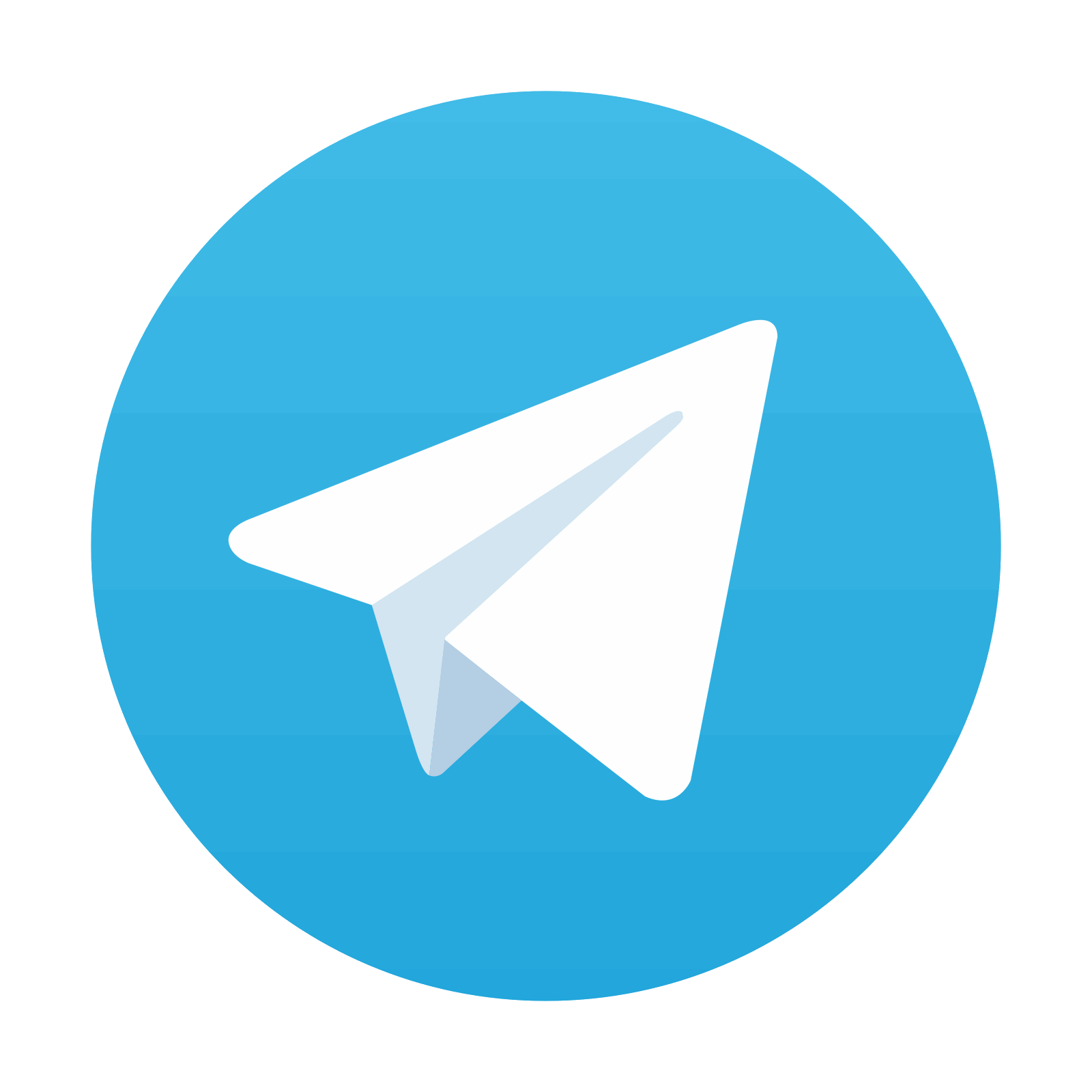
Stay updated, free articles. Join our Telegram channel

Full access? Get Clinical Tree
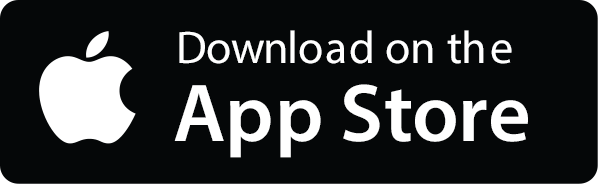
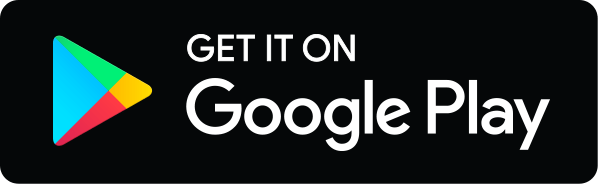
