Fig 1 a–c.
Acute pulmonary embolism. Transverse contrast-enhanced CT section (a) allows the diagnosis of a large intraluminal clot (arrow). Multiplanar reconstructions in the sagittal (b) and coronal (c) planes show the true sagittal and coronal extent of the clot (arrows)
Improved Morphological Evaluation of the Peripheral Pulmonary Vasculature
With the introduction of MDCT, CTA is now recognized as the reference standard for diagnosing acute PE [4]. A main advantage of MDCT over single-slice CT is the ability to scan the entire volume of the thorax with sub-millimetric collimation in a very short period of time, most often under the duration of a single breath-hold. This is particularly useful when evaluating dyspneic patients. These technological advances have improved both evaluations of the peripheral pulmonary arteries and the accuracy of CT in the workup of acute PE. Simultaneously, MDCT allows radiologists to scan patients at a low kilovoltage, with reductions in the dose of contrast material and the overall radiation dose. This is of particular importance in young female patients who may be exposed to substantial levels of radiation to breast tissue. In the context of acute PE, the latter concern is clinically relevant given the lower prevalence of acute PE, which has dropped from 33% on angiographic studies to <20% on CT/MDCT scans. To date, several studies have investigated the clinical benefits of low-kilovoltage techniques, i.e., 80–100 kV(p) vs. 120–140 kV(p), the parameters at which CTA is typically performed. However, for obvious ethical reasons, these studies were based on the comparative analysis of different populations scanned with single-source CT [5–8]. The limitations of these comparisons include the lack of systematic adjustment for individual patient morphology, cardiac hemodynamics, and potential underlying respiratory disease. With the introduction of DSCT, the two tubes can be set at different kilovoltages. In addition to the opportunity to evaluate lung perfusion, there are benefits for standard CTA as this scanning mode has been shown to improve the visualization of small pulmonary arteries at 80 kV(p) [9].
Perfusion Imaging with Dual-Energy CT
Lung perfusion with dual-energy CT does not reflect blood flow analysis per se, as it provides a measurement at only one time point; rather, it yields an iodine map of the lung microcirculation at a particular time point. Numerous parameters are known to influence the distribution of iodine within pulmonary capillaries; some are technique-related whereas others are due to the anatomical and/or physiological circumstances under which the data were acquired. Dual-energy CTA is performed using a scanning protocol similar to the one used in clinical practice. The acquisitions proceed from the top to the bottom of the chest, with an injection protocol similar to that of standard CTA obtained with a single energy on a 64-slice scanner. Two categories of images can be reconstructed: Diagnostic scans correspond to contiguous 1-mm-thick transverse CT scans generated from the raw spiral projection data of tube A and tube B (60% from the acquisition by tube A; 40% from the acquisition by tube B). Lung perfusion scans (i.e., images of the perfused blood volume of the lung parenchyma) are generated after determination of the iodine content of every voxel of the lung parenchyma on the separate 80- and 140-kVp images. The images can be rendered in gray-scale or color-coded. All images can be displayed as transverse scans, complemented as needed by corona land sagittal reformats. Even though a dual-energy acquisition does not correspond to true perfusion imaging, several applications of this pulmonary micro-CTA technique have been investigated [10].
Acute Pulmonary Embolism
Dual-energy CT can detect endoluminal clots on averaged images obtained with tubes A and B as reliably as can single-source CTA [11]. In a preliminary study, the detectability of perfusion defects beyond obstructive clots was validated. Perfusion defects in the adjacent lung parenchyma have the typical perfusion-territorial triangular shape well known from pulmonary angiographic, scintigraphic, and MRI perfusion studies. Dual-energy CTA can help predict perfusion defects without directly identifying peripheral endoluminal clots that may be located in subsegmental or more distal pulmonary arterial branches. It can also help differentiate lung infarction from less specific peripheral lung consolidation.
Chronic Pulmonary Embolism
Dual-energy CTA can depict perfusion defects distal to chronic clots (Fig. 2). Three vascular characteristics of chronic PE may manifest on dual-energy CT imaging. First, chronic PE causes a mosaic pattern of lung attenuation, characterized by areas of ground-glass attenuation, with enlarged vascular segments intermingled with areas of normal lung attenuation and smaller vascular segments. When present, these findings are suggestive of blood flow redistribution, but they are not consistently seen on conventional CT in patients with chronic PE. In these patients, dual-energy CT can detect ground-glass attenuation of vascular origin based on the high iodine content within the areas of ground-glass attenuation, thus enabling their distinction from ground-glass attenuation secondary to bronchial or alveolar diseases [12]. Second, chronic PE can cause calcifications within partially or completely occlusive chronic clots as well as within pulmonary artery walls, when chronic PE is complicated by longstanding or severe pulmonary hypertension. The calcifications are detectable using virtual non-contrast imaging, accessible by dual-energy CT imaging. Third, the images generated at 80 kV can improve visualization of the systemic collateral supply characteristic of chronic PE and originating from bronchial and non-bronchial systemic arteries.
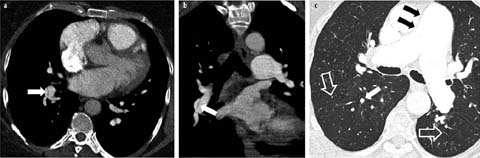
Fig 2 a–c.
Chronic thromboembolic disease. Transverse CT section (a) shows a web in the pulmonary artery of the right lower lobe (arrow), which is confirmed (arrow) by a coronal multiplanar reconstruction (b). Lung window (c) shows a dilated main pulmonary artery (black arrows), dilated peripheral pulmonary artery (white arrow), and parenchymal areas of hypoperfusion (open arrows)
Obstructive Airways Diseases
Abnormalities of pulmonary perfusion are a feature of numerous smoking-related respiratory diseases. Peinado et al. showed that endothelial dysfunction of the pulmonary arteries is present even in patients with mild chronic obstructive pulmonary disease (COPD) [13]. In these patients, as well as in smokers with normal lung function, some arteries show a thickened intima, suggesting that tobacco consumption plays an important role in the pathogenesis of pulmonary vascular pathologies in COPD. Several structural changes in the early stages of COPD have been described in experimental and animal models, including proliferation of smooth-muscle fibers within peribronchiolar arterioles and collagen and elastin deposition in the thickened intima of vessels [14, 15]. In preliminary studies, Hoffman et al. [16] showed an increased heterogeneity of the local mean transit times of contrast material within the pulmonary microvasculature of smokers with normal pulmonary function tests. In their dual-source, dual-energy CTA study of pulmonary lobar perfusion in COPD patients, Pansini et al. [17] found that nonsmokers had no alterations in lung structure and observed a uniform distribution of iodine content within the upper and lower lobes and between the right and left lungs. Perfusion scans showed significantly lower iodine content within the lung microcirculation of the upper lobes in emphysematous patients than in smokers without emphysema and a significantly lower perfusion in the upper than in the lower lung zones, consistent with the destruction of the lung parenchyma. These structural abnormalities have important implications given the epidemiologic and socioeconomic burden of COPD.
Restrictive Airways Diseases
The substantial importance of pulmonary hypertension on the clinical course and prognosis of patients with fibrotic lung disease has been extensively recognized. However, the mere measurement of pulmonary artery diameters might not be a reliable parameter in the assessment of disease severity due to the potentially confounding role of parenchymal traction on central and peripheral pulmonary vessels. Moreover, because of the age of the population in which these diseases usually occur, age-related changes have to be taken into account in morphometric-based clinical decision-making and disease classification. Overall, and despite promising initial scientific evidence, the roles of MDCT and DSCT in assessing patients with fibrotic lung diseases still need to be determined. The many ongoing pharmacological trials, notably in patients with usual interstitial pneumonits, may provide an ample study ground in this field.
Are There Indications for ECG-Gated CTA Examinations When Exploring Pulmonary Vascular Diseases?
Pulmonary Hypertension
Although a pulmonary trunk diameter 5⩾33.2 mm has 95% sensitivity for the diagnosis of pulmonary hypertension (PHT), the specificity of this measurement is only 58%, which is insufficient for the accurate diagnosis of PHT, notably in patients with mild disease [18]. Moreover, no correlation has been found between the degree of PHT and pulmonary trunk diameter. Electrocardiograph (ECG)-gated MDCT acquisitions of the entire thorax enable the evaluation of novel functional parameters in addition to the standard morphology. In patients with PHT, right pulmonary artery distensibility was recently shown to be an accurate predictor of PHT on ECG-gated 64-slice MDCT scans of the chest [19]. In that study, its diagnostic value was superior to that of the single measurement of pulmonary trunk diameter.
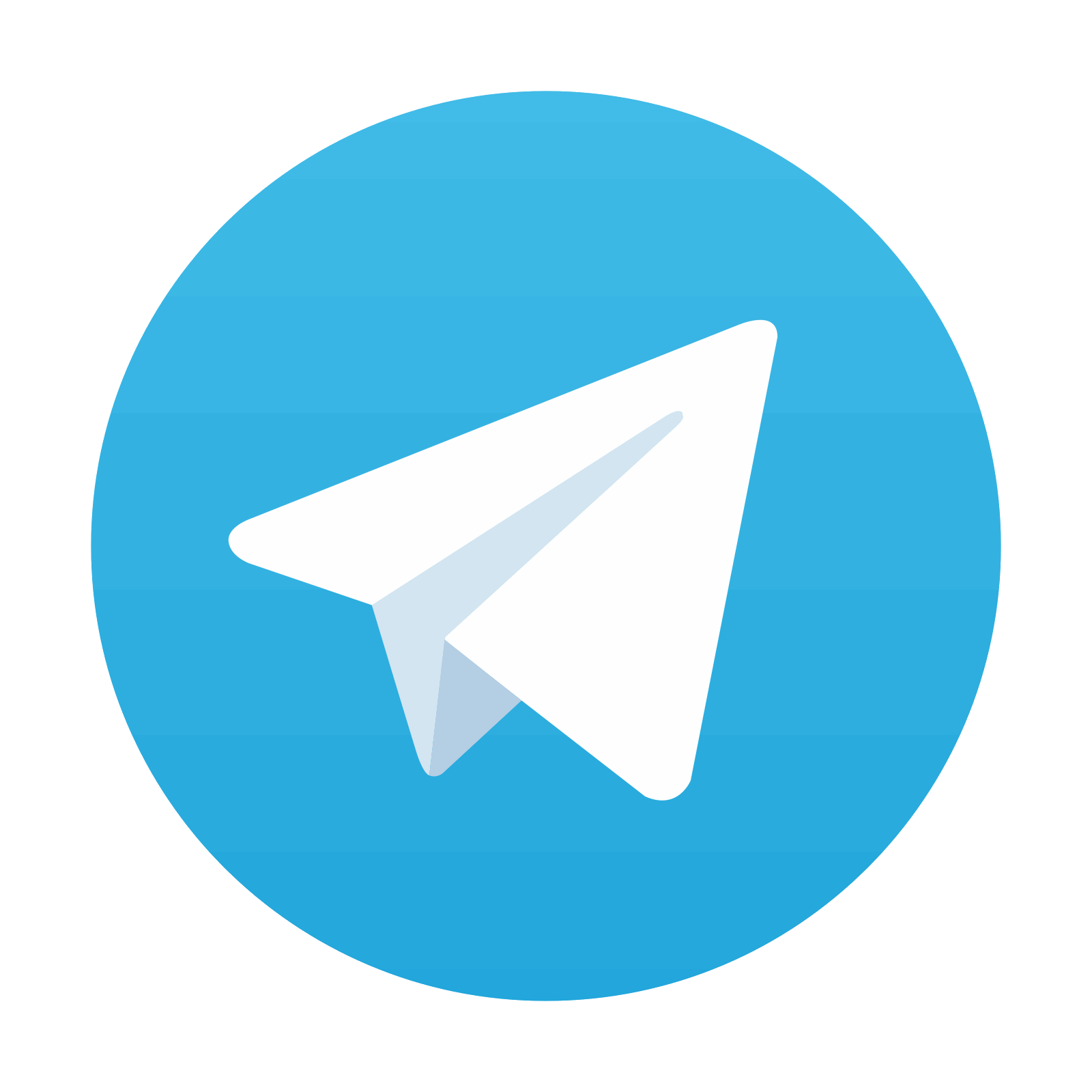
Stay updated, free articles. Join our Telegram channel

Full access? Get Clinical Tree
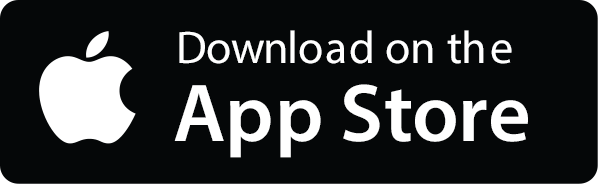
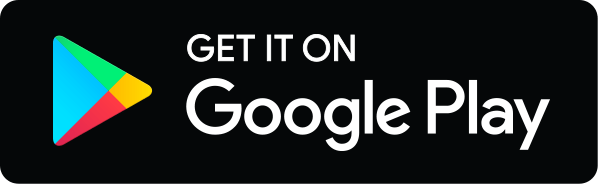