Diabetes mellitus is among the leading causes of mortality and major morbidity in the United States. It continues to plague society with an additional 800 000 new cases diagnosed each year.1 Consequently, the cost of treating the associated complications such as cardiovascular death, end-stage renal failure, and major amputations has created a growing economic burden on U.S. health care systems. Fifty percent of diabetics will be affected by a manifestation of diabetic foot (neuropathy, ischemia, or infection) and 15% of diabetics will experience a foot ulcer in their lifetime.2,3 Diabetic foot problems are among the leading causes of hospitalization for diabetics; the cost of caring for diabetic foot ulcers is estimated as high as $13 billion annually, a figure that is approximately 27% of the total cost of diabetes care.4 Its seriousness cannot be overstated; 20% of those affected with a foot ulcer will progress to an amputation, a lower extremity amputation rate of 4.1 per 1000 diabetics per year.1,5 This confers an amputation relative risk 40 times greater for diabetics and a reamputation rate of more than 60% at 5 years.3,6
The pathophysiology of the diabetic foot is a multifactorial problem including neuropathy, ischemia, and infection. Treatment is based on a multidisciplined approach and requires control of infection, evaluation of ischemia, arterial reconstruction, and ultimate wound closure.
The diabetic foot is a result of the synergistic derangements affecting the peripheral nerves, peripheral vasculature, and host defenses. The contribution each makes to a particular clinical situation may vary, but the underlying changes in physiology and function of these three systems are chiefly responsible for the cascade of events that lead to an insensate foot, ulceration, bone and joint destruction, infection, ischemia, and possibly limb loss (Figure 42-1).7 An understanding of these primary processes is essential to treating a patient with diabetic foot.
FIGURE 42-1.
Underlying mechanisms of diabetic foot ulceration. Sensorimotor neuropathy leads to diminished sensation and small muscle atrophy in foot, resulting in flexed metatarsals, metatarsal head prominence, and clawing of toes. Altered architecture of foot, coupled with ischemia and microvascular dysfunction, ultimately leads to ulceration.
Reproduced with permission from Akbari CM, LoGerfo FW. Diabetes and peripheral vascular disease. J Vasc Surg. 1999;30:373–384.

An estimated 15% to 50% of diabetics have some degree of peripheral neuropathy.4 The importance of neuropathy as a contributory cause to foot ulceration was confirmed in a large multicenter study from Europe and North America that reported a 7% annual risk of ulceration in neuropathic patients. Previous evidence had suggested that the risk in non-neuropathic patients is less than 1%.8 Diabetic neuropathy is a result of segmental demyelination and axonal and neuronal degeneration. The nerve cell transmits afferent signals (pain, temperature, proprioception) along the axon to the neuron body and transmits efferent signals (motion, reflexes) in the opposite direction “down” the axon. Signal conduction is a complex process of electrical potential changes conducted along the axon. This conductance depends on the highly metabolic axon’s ability to generate and maintain electrical action potentials (electrical gradients) across its membrane and also on the support of the myelin sheath that surrounds the axon as both an insulator and conductor. This myelin sheath is produced by the metabolically active Schwann cells that line the axon. Hyperglycemia and/or hyperinsulinemia cause defects in neuron and Schwann cell metabolism that result in cellular dysfunction and subsequent impaired signal conduction. There are several well-studied theories of how diabetes causes this neuronal dysfunction. Evidence suggests that a combination of metabolic derangements with microvascular dysfunction (discussed below) lead to creation of oxidative stress, alteration of cellular energy and substrate handling, production of abnormally glycosylated proteins, and creation of an environment of “nerve hypoxia.”9,10
Oxidative stress occurs when the production of free radical moieties exceeds the antioxidant capacity of a cell or system. Free radicals attack and damage the proteins, lipids, and nucleic acids. These oxidized or nitrosylated products have decreased biological activity, leading to loss of energy metabolism, cell signaling, transport, and other major functions. Free radicals in the form of reactive oxygen species (such as superoxide, hydrogen peroxide, and nitric oxide) are byproducts of the glycolytic pathway. Under normal conditions, their presence can be controlled by antioxidant systems. However, under conditions of hyperglycemia these radicals are overproduced and damage cellular proteins, DNA, and disrupt energy pathways. The damage can be far reaching within each cell, and in the case of neurons and Schwann cells the critical process of signal conduction is hindered.11,12 Increasing the oxidative stress level within cells also causes a proinflammatory state and induces the process of apoptosis and cell death.
Altered energy and substrate pathways also lead to cellular damage. One well-described pathway, the polyol pathway, has been implicated in diabetic neuropathy. The enzyme aldose reductase converts toxic aldehydes to inactive alcohols. Glucose is a poor substrate for aldose reductase, but in hyperglycemia this enzyme coverts glucose to sorbitol, initiating the polyol pathway of glucose conversion to fructose. Increased aldose reductase activity in response to hyperglycemia, in turn, has been shown to result in several deleterious effects. It causes a decrease in nitric oxide (NO) synthase activity with reduced production of nitric oxide—a potent vasodilator and effector of vascular smooth muscle cells (VSMCs). It consumes and depletes the intracellular supply of the cofactor NADPH, this interferes with several cellular pathways and increases the oxidative stress level within a cell. It also impairs myo-inositol uptake by the Schwann cell. Myo-inositol is the precursor in the inositol containing phospholipids which are essential to myelin production and nerve conduction.13
Hyperglycemic conditions lead to the nonenzymatic glycolation of proteins. These products are termed advanced glycation endproducts—AGEs. Formation of AGEs may damage cells by inducing modifications of extracellular or intracellular proteins. Modification of an extracellular protein such as collagen causes alterations in its structure, strength, and electrical charge. This results in basement membrane thickening which represents a poorly functioning barrier between tissues and the intracellular environment. This barrier is critical in the maintenance of signaling, transportation of cellular products, and local homeostasis. Modification of intracellular proteins causes nonspecific binding to a family if IgG related transmembrane receptors (RAGEs) that produces a cascade of cellular signaling events. One of these, the activation of the mitogen-activated protein kinase (MAP kinase) causes dysfunction of pathways involved with gene expression, mitosis, and apoptosis.10 Disruption of the pathway that controls sodium–potassium adenosine triphosphatase expression and activity leads to an inability to maintain adequate membrane electrical potentials. This results in the loss of electrical conduction in neural tissue and segmental demyelination of the axon.14
Another cause for diabetic neuropathy is localized “nerve hypoxia.” This is a result of pathologic blood shunting and increased vascular resistance (discussed below under autonomic dysfunction) leading to decreased endoneurial blood flow.15,16 Poor endoneurial blood flow is made worse by the metabolic derangements of hyperglycemia and hyperinsulinemia on NO production by the endothelial cells of the nerve blood supply—the vasa vasorum.17 Decreased NO production leads to VSMC dysfunction that can result in an occlusive-like process of these nutrient vessels. The end result of all these injurious processes manifests itself microscopically as basement membrane thickening, breakdown of the myelin sheath into separate conglomerates, and axon degeneration. The nerves of the distal part of the leg are more affected than the proximal nerves and the cell bodies themselves mirror a pattern of injury involving the distal segments of the spinal cord first.18 The clinical manifestation is delayed nerve conduction velocity that seems to affect initially unmyelinated or thinly myelinated nociceptive sensory nerve fibers but gradually involves all nerve types. Clinically, these do result in a neuropathy involving all three neural systems—sensory, motor, and autonomic—that contribute to ulceration.
The loss of sensation leads to a loss of the protective nature of pain perception. The onset is usually insidious and often unrecognized by the patient. Some patients may complain of uncomfortable, painful, and paresthetic symptoms in a symmetrical, “stocking” distribution, but just as commonly there will be no symptoms. Another manifestation of sensory neuropathy also involves autonomic nerves and is called the axon reflex. Injury stimulating a nociceptive fiber not only conducts to the spinal cord but also in a local arc to adjacent fibers and other axon branches near the site of stimulation. One function of this axon reflex is to stimulate secretion of several active peptides, such as substance P and calcitonin gene-related peptide, which directly and indirectly (through mast cell release of histamine) cause vasodilation and increased permeability—allowing for the delivery of cells and other factors involved in inflammation and healing. Because of sensory nerve dysfunction and delayed signal conduction in diabetes, this neurogenic vasodilatory response is impaired thereby blunting the hyperemic response when it is most needed under conditions of injury and inflammation.19
The clinical presentation of motor nerve dysfunction is wasting of the small muscles in the feet and absent ankle reflexes. This chronic motor denervation results in malfunction of the intrinsic muscles of the foot that distorts foot architecture. Chronic metatarsal flexion, extensor subluxation of the toes, proximal migration of the metatarsal fat pad and an imbalance in the action of the toe flexors and extensors leads to a claw foot deformity. More importantly, with dislocation of the metatarsophalangeal joints the heads of the metatarsals become more prominent and become the striking surface during ambulation. Other bony prominences become abnormal pressure points as well and combined with a loss of pain sensation the overlying skin is subject to repeated injury and ulceration.
Sympathetic autonomic neuropathy leads to a loss of sympathetic tone and increased arteriovenous shunting in the foot. This can mistakenly give the impression of a warm, well-perfused foot, but effective nutrient and oxygen delivery is actually impaired. Autonomic denervation of the skin leads to reduced sweating, dry skin, and development of cracks and fissures which predispose to skin breakdown. Blood flow to the bone becomes unregulated and the increased flow causes osteopenia and “bone washout” which may also contribute to osteoarthropathy and decreased joint mobility. Continued ambulation on an insensate joint, combined with muscle imbalance and atrophy from motor neuropathy and limited joint mobility, leads to joint instability, loss of joint architecture, and ultimately bone and joint destruction.20 This process, leading to collapse of the ankle mechanism and collapse of the plantar arch, results in the deformity known as Charcot’s foot.
The incidence of lower extremity arterial disease is roughly four times greater among patients with diabetes compared to nondiabetics. Moreover, diabetics with peripheral arterial disease (PAD) carry a two- to threefold excess risk of intermittent claudication.21 Of the numerous risk factors for PAD, diabetes is one of the two strongest factors (smoking is the other.) For diabetics the risk of developing PAD is related to age, duration of diabetes, and presence of neuropathy.22 Diabetics are also more likely to present with a more severe form of ischemia and more likely to progress quicker to critical limb ischemia.23 The impact of PAD is quite significant, approximately 27% of PAD patients demonstrate progression of symptoms over a 5-year period with limb loss occurring in approximately 4%. During this same 5-year period there is a 20% stroke or myocardial infarction risk and a 30% mortality rate.24 For those with critical limb ischemia the outcomes are worse: 30% will have amputations and 20% will die within 6 months.25 The natural history of PAD in diabetics has not specifically been studied longitudinally, but it is known from prospective clinical trials of risk interventions that the cardiovascular event rates in patients with PAD and diabetes are higher than those of their nondiabetic counterparts.22
Arterial disease in diabetes may be summarized as an alteration in vascular function at both the microvascular and macrovascular levels.7 The microvascular component is best described as a nonocclusive microcirculatory impairment. This should not be confused with the term “small vessel disease” which refers to the common misconception of an untreatable occlusive lesion in the microcirculation. Dispelling the notion of “small vessel disease” has been fundamental to diabetic limb salvage, because arterial reconstruction is almost always possible and successful in these patients.3 Whereas there is no occlusive disease in the microcirculation, multiple structural and physiologic abnormalities result in a functional microvascular impairment.26 Endothelial cell dysfunction as a result of hyperglycemia and hyperinsulinemia has been well studied and shown to play a major role in this functional defect.27 NO is the main vasodilator released by the endothelium and causes vasodilation by diffusing into the adjacent VSMCs and stimulating cyclic guanosine 3′,5′-monophosphate mediated relaxation. NO is synthesized in the endothelial cell through the action of an endothelial specific NO synthase (ecNOS). The expression of ecNOS has been shown to be reduced in response to hyperglycemic and hyperinsulinemic conditions.27 Also, loss of NO homeostasis at the microcirculatory level creates a proinflammatory environment with liberation of damaging oxygen free radical species into the vasculature and surrounding tissues.
Another effect of hyperglycemia is the nonspecific glycosylation of proteins, so-called ts (AGEs). AGEs impair the actions of NO by stimulating the formation of free oxygen radicals that react with NO and convert it to a pro-oxidant. AGEs also displace disulfide cross-linkages in collagen proteins thereby diminishing the charge in the capillary basement membrane and altering its diffusion properties.28 These basement membrane alterations contribute to increased vascular permeability and inflammation. AGEs activate and upregulate the expression of endothelial AGE receptors—these add to the local inflammatory state by increasing leukocyte chemotaxis and transformation into foam cells which contribute to increasing local oxidative stress.22 One result of this increase in inflammation is an increase in C-reactive protein which is strongly related to widespread acceleration of atherosclerosis and promotion of endothelial cell apoptosis.29 These and other mechanisms result in the impaired microvasculature marked by a characteristic thickening of the capillary basement membrane which does not affect arteriolar luminal diameter or blood flow but does impair nutrient and substrate flow into the adjacent tissues. This, coupled with autonomic dysfunction at the capillary level described earlier, severely hinders the hyperemic response to injury, inflammation, and infection.
The macrovascular component of PAD in diabetics is caused by atherosclerosis. The atheromatous changes occur in a similar fashion as in nondiabetics, but in an accelerated way. This acceleration could be because of the previously described diabetes-driven increases in inflammation that worsen the course of “normal” plaque pathophysiology, changes in platelet and coagulation system function, and the high coincidence of hypertension among diabetics caused by diabetic nephropathy.
Another common finding among diabetics is extensive medial calcification of the arteries. This is a process that can occur either at or separate from sites of atheromatous plaque, and in diabetics is characteristically found throughout the arteries of the legs. There are several different disease states and proposed pathways for this abnormal calcification of the media; in diabetics both hyperinsulinemia and hyperglycemia are implicated. Both have been shown to alter gene and protein expression in endothelial and VSMC that directly result in “osteoblast-like” activity of the VSMC and perictye cells of the artery.30 An example is the abnormal expression of proteins like osteopontin by these cells. Osteopontin coupled with an environment of chronic inflammation, high presence of oxygen free radicals, and C-reactive protein within the vessel wall lead to the deposition of calcium phosphate complexes that mineralize within the media. Although this is generally a nonobstructive lesion it leads to noncompliant arteries unable to augment flow in response to increased demand and, depending on the luminal diameter of the vessel, long segmental stenoses that disturb normal blood flow.
As mentioned before, the formation of atheromatous plaques in diabetics is similar in most regards to nondiabetics, but the pattern of involvement has a unique characteristic in diabetics. Despite sometimes the presence of widespread calcinosis are found, the larger iliofemoral arteries are commonly spared of hemodynamically significant disease. However, in diabetics the popliteal and infrapopliteal vessels are more frequently involved than the larger arteries and more frequently diseased compared to nondiabetics.31 The foot vessels are relatively spared in diabetics, even in the face of severe tibial level disease, which is important to the success of revascularization.32
In addition to the effects on the endothelium and VSMC, diabetes also leads to a hypercoagulable state through alterations in platelet function, coagulation, and blood rheology. Platelet uptake of glucose is unregulated in hyperglycemia and results in increased oxidative stress which enhances platelet aggregation. These platelets also have increased expression of glycoprotein Ib and IIb/IIIa receptors which are important in thrombosis and adhesion. The coagulation system is affected by diabetic-related increases in tissue factor expression by VSMC and endothelial cells, and increases in plasma concentrations of Factor VII. Hyperglycemia is also associated with a decreased concentration of antithrombin and protein C, impaired fibrinolytic function, and excess plasminogen activator inhibitor-1.33 Blood rheology is altered as a consequence of an increase in viscosity and fibrinogen content caused by hyperglycemia.
In summary, the effects of PAD in diabetics confer alterations in the microvascular functioning and macrovascular supply that lead to ischemia. Because of the synergistic consequences of both processes, the actual degree of ischemia can be greater than suspected and even relatively minor trauma or infection can be made worse because of vascular insufficiency. The contribution of neuropathy with even moderate levels of ischemia is particularly worrisome as these “neuroischemic” feet are more prone to ulceration and infection.34,35
The presence of infection in a diabetic foot is usually a consequence of the neuropathy and ischemia already described (i.e., it typically follows an ulcer) and the impaired host defenses brought upon by hyperglycemia and hyperinsulinemia. Skin breakdown and ulceration allow a portal of entry for bacteria. Inadequate blood flow, blunted hyperemic response, and dysfunctional delivery of nutrients and immunologic cells create an environment ideally suited for bacterial overgrowth. Foot wounds are the most common diabetes-related cause for hospitalization. Infected diabetic foot wounds precede two-thirds of lower extremity amputations, and diabetics have at least a 10-fold greater risk of being hospitalized for soft tissue and bone infections of the foot than individuals without diabetes.36 Among diabetics the risk for developing an infection is related to control of diabetes, presence of neuropathy, presence of PAD, chronicity of wound (>30 days), a recurrent wound, and a traumatic wound.35,37
The hyperglycemic state enhaces conditions that promote infection in diabetic patients. Studies have shown that nearly every component of the immunological system is negatively affected by hyperglycemia38 (Table 42-1). For example, leukocyte adhesion and transmigration to the site of injury is impaired by poor chemotaxis signaling and receptor activity. The intracellular signaling necessary for bactericidal and phagocytic activity is also interrupted. When glucose levels exceed 200 mg/dL, tissue glycosylation occurs, which impairs wound healing by increasing the collagenase activity that reduces the collagen content at the site of tissue injury. Poor vascular supply and oxygenation provides a fertile environment for development of a synergistic microbial infection. Tight blood glucose control, especially in ill and infected patients, has been shown to reduce morbidity and mortality and should be viewed as an important component in the management of diabetic foot infections.39,40
Immune System Component | Effect |
---|---|
Phagocytosis | Impaired phagocytosis by neutrophils |
Chemotaxis | Impaired migration by neutrophils |
Apoptosis | Increased neutrophil apoptosis after LPS challenge |
ROS generation | Reduced generation of ROS, reduced killing |
Adhesion/transmigration | Increased expression of adhesion molecules on endothelium and leukocytes |
Complement cascade | Increased plasma level of complement products; |
Decreased complement function | |
Cytokine network | Increased early proinflammatory cytokine levels (TNF-α, Il-1β, IL-6)—this effect reversed in hyperinsulinemia |
The microbiology of diabetic foot infections is unique, gram-positive, gram-negative, and anaerobes can be responsible (Table 42-2). Staphylococci and streptococci are the most common pathogens. However, infection caused by gram-negative and anaerobic organisms occurs in approximately 50% of patients and quite often infection is polymicrobial (>3 organisms).37 S. aureus is the most common organism, and methicillin-resistant Staphylococcus aureus (MRSA) is increasingly found in infected ulcers. Because of the poor immune response to infection and inflammation even bacteria regarded as skin commensals may cause severe tissue damage. These include gram-negative organisms such as Citrobacter, Serratia, Pseudomonas, and Acinetobacter species. Proper evaluation and antimicrobial treatment will be covered below, but it is advisable to send swabs or tissue for culture after initial debridement in all patients with deep ulcers or any evidence of local infection (warmth, swelling, cellulitis, foul smelling drainage, pus). Deeper wounds should raise the possibility of more than a soft tissue infection and osteomyelitis should be considered. In osteomyelitis, superficial swab cultures do not reliably identify bone bacteria, but percutaneous bone biopsy seems to be safe for patients with diabetic foot osteomyelitis.37 Necrotizing soft tissue infections are also more common in diabetics, and an understanding of the anatomy and muscular compartments of the foot is essential in understanding the pattern of spread and control.
Foot-Infection Scenario | Pathogens |
---|---|
Cellulits without an open skin wound⋆ | β-Hemolytic streptococcus and Staphylococcus aureus |
Infected ulcer and antibiotic naive⋆ | S. aureus and β-hemolytic streptococcus |
Infected ulcer that is chronic or was previously treated with antibiotic therapy† | S. aureus (includes MRSA) and β-hemolytic streptococcus, and Enterobacteriaceae |
Ulcer that is macerated because of soaking† | Pseudomonas aeruginosa (often in combination with other organisms) |
Long-duration nonhealing wounds with prolonged, broad-spectrum antiobiotic therapy†, ‡ | Aerobic gram-positive cocci (S. aureus, MRSA, coagulase-negative staphylococci, and enterococci), Enterobacteriaceae, Pseudomonas spp.,Acinetobacter spp., Stenotrophomonas spp., fungi |
“Fetid foot”: extensive necrosis, gangrene, malodorous† | Mixed, aerobic gram-positive cocci, Peptostreptococcus, nonfermenting gram-negative rods, Escherichia coli, Citrobacter, Proteus, Serratia, Bacteroides |
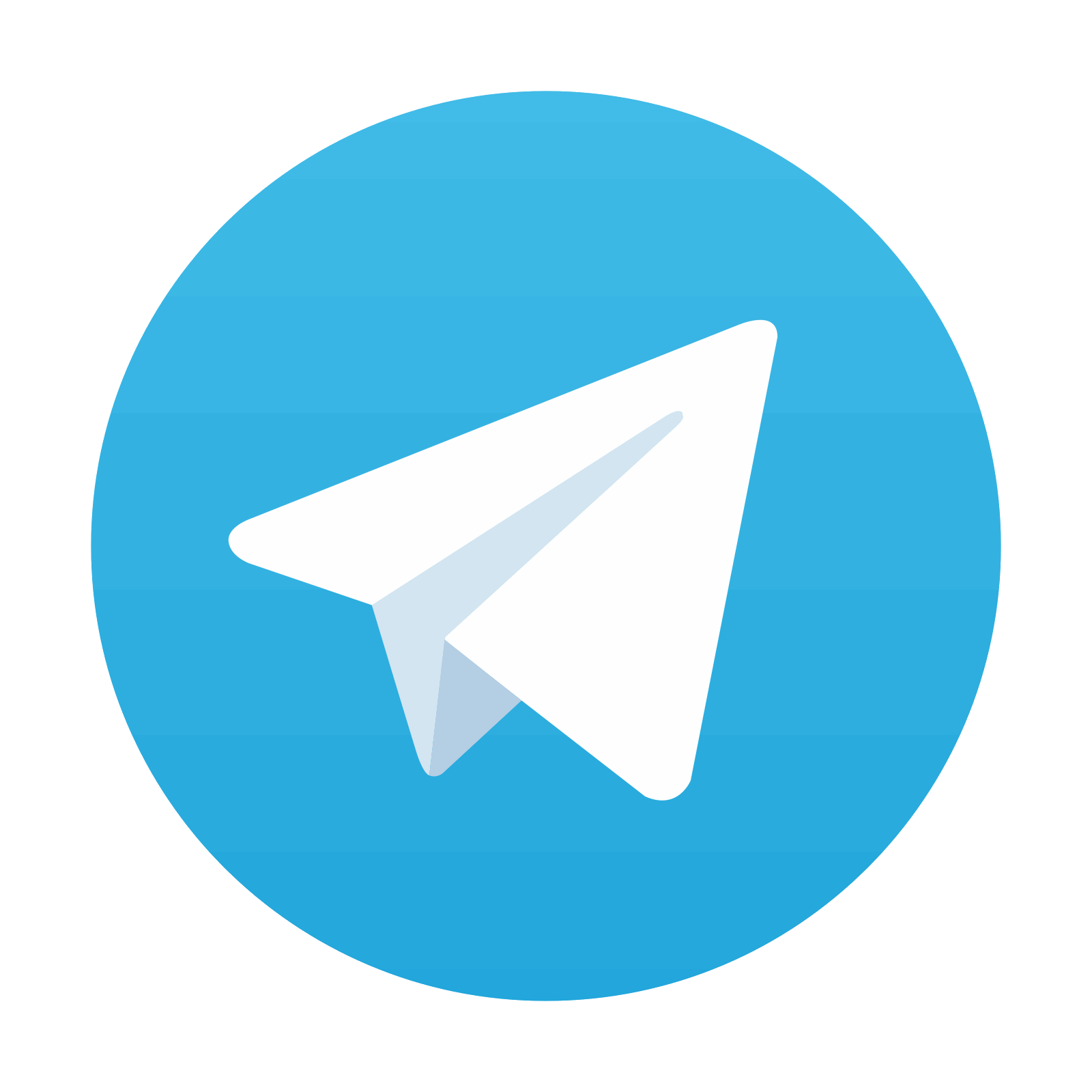
Stay updated, free articles. Join our Telegram channel

Full access? Get Clinical Tree
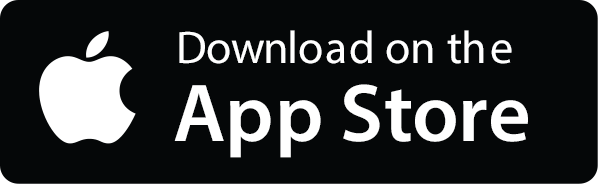
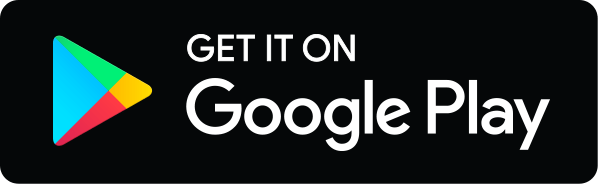
