Fig. 5.1
(a) Charcot foot demonstrating characteristic loss of the plantar arch with a “rocker bottom” deformity. (b) Radiographic image of Charcot foot demonstrating bone destruction and loss of the normal architecture of the foot
Classification
The International Working Group on the Diabetic Foot (IWGDF) and the Infectious Disease Society of America (IDSA) outlined clinical criteria for diagnosing and classifying the severity of diabetic foot infections [7, 8]. This classification can serve as a guide for the type treatment, duration of therapy, and outcome predictions. Grade 1 wounds lack purulence or any manifestation of inflammation and are considered uninfected. Grade 2 indicates the presence of two or more manifestations of inflammation such as purulence, erythema, pain, warmth, or induration. At this severity level cellulitis does not extend beyond 2 cm around any ulcer site, inflammation is limited to skin or superficial subcutaneous tissues, and there is no associated systemic illness. Grade 3 infections occur in systemically and metabolically stable patients who have cellulitis that extends more than 2 cm around any ulcer site, lymphangitic streaking, or infection that spreads below the superficial fascia. Other features of a Grade 3 infection include deep-tissue abscesses, gangrene, or involvement of the muscle, tendon, joint, or bone. Finally, severe or Grade 4 infections are those accompanied by systemic toxicity or metabolic instability such as fever, hypotension, tachycardia, confusion, leukocytosis, acidosis, hyperglycemia, and azotemia.
A prospective study validated this classification system by following 199 infections: 48 % classified as mild, 34 % moderate, and 18 % severe [9]. The study reported a statistically significant correlation between the defined severity of infection and the risk of amputation, anatomic level of amputation, and need for hospitalization. Patients with mild infections are unlikely to develop osteomyelitis and do not require hospitalization or amputations, whereas those with more severe disease warrant aggressive medical and surgical care. The accuracy of clinical assessment decreases in patients with neuropathy, ischemia, and immune dysfunction. This triad of complications can diminish the neuroinflammatory response to infection in patients with diabetes masking the classic physical findings of inflammation and infection.
Diagnosis of Diabetic Foot Infection
Several factors influence the microbiology of diabetic foot infections including the prior use of antibiotics and whether the infection is acute versus chronic and superficial versus deep [3]. Drawing a line between infection and bacterial colonization helps define the potentially complex and polymicrobial microbiology. The mere presence of organisms in a wound does not translate into a clinical infection as all chronic wounds and ulcers open to skin commensals become colonized with multitudes of bacteria. Infection develops as bacteria multiply within the wound, overwhelming host defenses as a result of critical numbers, microbial enzymes, or impaired host immunity. Although a colony count of 105 bacteria per gram of tissue often defines infection, this number may vary depending on the organism [10]. Virulent bacteria such as beta-hemolytic streptococci have enzymes that promote tissue invasion leading to rapidly progressive infections despite quantitatively low bacterial burdens. This clinical scenario demonstrates that infection depends on the type of organism and its characteristics in addition to the absolute number of bacteria present.
The technique used to collect wound cultures plays an important role in defining the microbial etiology of a diabetic foot infection. Culturing the surface of a purulent ulcer should be avoided since it will reflect wound colonization and fail to isolate the causative bacteria. Recommended modalities for obtaining cultures include: scraping or curetting the wound base after debridement, aspirating an abscess, or tissue biopsy. A properly obtained wound culture specimen ensures appropriate antibiotic coverage for the organisms involved based on their susceptibility.
The diagnosis of diabetic wound infections cannot rely on wound culture results alone; it also requires clinical findings consistent with infection. Sotto et al. examined the importance of pathogens versus colonizers in patients with foot ulcers not on prior antibiotics who were growing Staphylococcus aureus as the sole organism from wound cultures [11]. Using the IDSA classification system and oligonucleotide arrays to detect genes encoding various virulence factors and antibiotic resistance profiles, they found that both resistance and virulence factors were more likely to be present in infected versus colonized wounds. Virulence factors were present in only 9 % of Grade 1 (uninfected) patients versus 98 % of those with infected ulcers. Jeandrot examined inflammatory markers, serum procalcitonin, and C-reactive protein as a means of distinguishing mildly infected from noninfected diabetic foot ulcers [12]. C-reactive protein had the highest sensitivity and specificity for distinguishing Grade 2 (mild) from Grade 1 (uninfected) ulcers. Combining C-reactive protein and procalcitonin levels increased the accuracy of predicting infection.
Although it can be challenging, diagnosing osteomyelitis is essential to ensure appropriate treatment. The presence or absence of osteomyelitis often determines the duration of antibiotic therapy and the need for surgical debridement. A bone biopsy provides the most reliable test for osteomyelitis. The diagnosis of osteomyelitis can be made by isolating bacteria from an aseptically obtained bone sample which has histologic features of infection (inflammatory cells, necrosis). Stopping antibiotics 2 weeks in advance of the biopsy can decrease the probability of a false-negative result. A CT or fluoroscopically guided percutaneous bone biopsy has proven to be a safe and accurate alternative to a surgical biopsy. The presence of ischemia increases the risk of nonhealing making an invasive procedure such as a bone biopsy less appealing. If a bone biopsy is not available or contraindicated by ischemia, the diagnosis of osteomyelitis depends on surrogate markers including clinical, laboratory, and imaging findings.
Nonhealing ulcers over bony prominences should raise the suspicion of underlying osteomyelitis. Other clinical findings associated with osteomyelitis include exposed bone and large ulcers. In the appropriate clinical setting, probing to bone can help make the diagnosis at the bedside. The technique involves gently inserting a sterile, blunt metal probe through the ulcer. Striking bone increases the likelihood of osteomyelitis if the prevalence of bone infection is high (>60 %). If the prevalence of bone infection is low (<20 %), failure to probe to bone can eliminate the diagnosis of osteomyelitis.
Osteomyelitis usually causes the erythrocyte sedimentation rate (ESR) to rise. An ESR greater than 70 increases the likelihood of osteomyelitis, while a low ESR can help rule out the diagnosis. Other laboratory values such as C-reactive protein, procalcitonin, and blood leukocyte count have less accumulated evidence linking them to osteomyelitis.
Radiologic evaluation for osteomyelitis usually begins with plain films of the foot in two or three views. Table 5.1 lists X-ray findings consistent with osteomyelitis. The sensitivity of plain X-rays ranges from 28 to 75 % and seems to depend on the timing of the imaging. Long-standing cases are more likely to show radiographic signs of osteomyelitis compared to cases that have only been present for 1 or 2 weeks. Overall the presence or absence of plain X-ray findings can neither confirm nor eliminate the diagnosis of osteomyelitis. Plain X-rays may be more predictive when they document sequential changes over time (greater than 2-week interval between films).
Table 5.1
Plain X-ray findings suggesting osteomyelitis
Periosteal reaction or elevation |
Loss of cortex with bony erosion |
Focal loss of trabecular pattern or marrow radiolucency |
New bone formation |
Bone sclerosis with or without erosion |
Sequestrum: devitalized bone with radiodense appearance that has become separated from normal bone |
Involucrum: a layer of new bone growth outside existing bone resulting from the stripping off of the periosteum and new bone growing from the periosteum |
Cloacae: opening in involucrum or cortex through which sequestra or granulation tissue may be discharged |
Magnetic resonance imaging relies on the presence of marrow edema to provide a faster and potentially more accurate diagnosis of osteomyelitis. Unfortunately, marrow edema is not specific to infection and may be seen with other conditions including gout and neuropathic osteoarthropathy such as Charcot foot which can complicate diabetes mellitus. Distinguishing Charcot arthropathy from infection can be difficult, and this may explain why some MRI studies have reported a specificity of only 60 % [13].
Nuclear medicine offers a variety of techniques for detecting osteomyelitis. Technetium radionuclide bone scans suggest osteomyelitis when increased blood pool activity and radionuclide intensity localizes to the bone. Although these exams are sensitive, the incidence of false-positive bone scans exceeds 50 % in many studies [14]. Combining technetium scans with indium111 white blood cell scans may improve diagnostic accuracy [15]. Recent reports using 18F-fluorodeoxyglucose positron emission tomography as a means of differentiating soft-tissue infections from osteomyelitis have been promising, but larger studies are needed to evaluate this approach [16].
Microbiology of Diabetic Foot Infections
Aerobic gram-positive cocci colonize the skin and frequently cause acute infections. In acutely infected patients, commonly isolated pathogens include Staphylococcus aureus and beta-hemolytic streptococci (groups A, B, C, and G) [17]. In contrast, chronic wounds tend to be polymicrobial with a mixture of aerobic and anaerobic bacteria [17, 18]. The recovery of anaerobes requires appropriate methods of sampling, transportation, and processing of the tissue. Aerobic organisms frequently include gram-positive cocci, namely, staphylococci, streptococci, and enterococci. A wide variety of aerobic gram-negative bacilli can also be isolated from chronic diabetic foot infections including Enterobacteriaceae (Escherichia coli), Proteus, Klebsiella, and Pseudomonas. Pseudomonas aeruginosa is especially prominent in ulcers that are macerated, and anaerobic organisms (Peptococcus, Peptostreptococcus, Clostridia, Fusobacterium, Bacteroides) are often found in the setting of ischemia and gangrene [19].
Hospitalization and prolonged antibiotic therapy can alter the sensitivity profile of organisms. Antibiotic naïve patients tend to have wounds with predominantly gram-positive organisms, whereas patients previously treated with antibiotics have wounds harboring gram negatives with increased antibiotic resistance requiring broader and longer courses of therapy. Richards et al. studied 188 patients with diabetic foot infections and identified 45 individuals with multidrug-resistant organisms [20]. The risk factors for developing multidrug resistance were deep and recurrent ulcers, prior hospitalization, elevated hemoglobin A1C levels, and proliferative retinopathy. After multivariate analysis, only prior hospitalization and proliferative retinopathy remained as significant risk factors for multidrug-resistant organisms. Interestingly, the presence of multidrug-resistant organisms did not affect the time to wound healing.
Osteomyelitis in patients with diabetes usually results from the spread of a contiguous soft-tissue infection. As such, osteomyelitis and diabetic foot infections share a similar microbiologic spectrum. Staphylococcus aureus is the predominant organism with Enterobacteriaceae being more common than Pseudomonas species [21]. In addition to these traditional pathogens, impaired host defenses and necrotic soft tissue and bone may allow low virulence, colonizing organisms such as coagulase-negative staphylococci or Corynebacterium species to become pathogens [6].
Treatment of Diabetic Foot Infections
The treatment of diabetic infections depends on the severity and extent of infection. The current recommendations for treatment of diabetic foot infections are based on expert opinion and consensus rather than well-controlled clinical trials. Nevertheless, it is clear that comprehensive management of diabetic foot infections requires a multidisciplinary team approach. Ideally the team managing these complex infections should include surgeons, podiatrists, infectious disease specialists, endocrinologists, and radiologists.
The absence of well-controlled clinical trials and other factors have made it difficult to provide clear-cut recommendations regarding antimicrobial therapy. There is a lack of standardized definitions for infection, improvement, and cure especially when surgical intervention is included in the protocol. The heterogeneity of the patient population has made study design challenging. Patients vary widely with respect to arterial supply, duration of infection, and preexisting comorbidities. Crouzet et al. reviewed antibiotic regimens for diabetic foot infections to assess the quality of randomized trials conducted between 1999 and 2009. They found great heterogeneity with respect to study design, selection criteria, duration of therapy, and microbiological end points and concluded that further studies were needed to develop standardized outcome definitions and guidelines for therapy.
Since there are no standardized recommendations, treatment decisions for diabetic foot infection must rely on knowledge of the likely pathogens and the spectrum of antibiotics that can reliably provide coverage. Noninfected ulcerations of a diabetic foot do not require antibiotic treatment. An ulcer with a clean base and healthy granulation tissue that does not probe to bone, and has no evidence of erythema, edema, or other signs of inflammation, does not warrant antibiotic therapy. Chantelau et al. studied the effect of oral antibiotics on neuropathic diabetic foot ulcers and found no added benefit from antibiotic treatment as a supplement to standardized therapy of pressure relief and wound care [22].
Mild infections (IDSA classification Grade 2) are usually caused by aerobic gram-positive cocci, predominantly Staphylococcus aureus, and Group B streptococci [23]. Anaerobic organisms are rarely isolated in these patients. Therefore, mild skin and soft-tissue infections can be treated similarly to those in the nondiabetic patient population using antibiotics with activity against gram-positive organisms. Moderate to severe infections (IDSA classification Grade 3 and 4) are likely to be polymicrobial with a mixture of aerobic gram-positive cocci, aerobic gram-negative rods, and anaerobes. In this setting, antibiotics with a broader spectrum of antimicrobial activity are warranted. In one of the largest studies of moderate and severe diabetic foot infections, Lipsky and coworkers conducted a randomized, double-blinded, multicenter trial comparing ertapenem to piperacillin/tazobactam [24]. The clinical success rates for ertapanem and piperacillin/tazobactam were similar (94 % vs. 92 %). Interestingly, although ertapenem does not provide coverage for Pseudomonas or enterococci, the clinical response for patients from whom these organisms were isolated was similar. This result suggests that either these bacteria are not particularly pathogenic in this clinical situation or that their role in polymicrobial infections may be dependent upon the microbial milieu. These findings support the theory that providing coverage for most, if not all of the bacteria present, when combined with appropriate surgical intervention, may be adequate treatment for moderate to severe diabetic foot infections.
Antibiotic Options for Gram-Positive Coverage
Although enterococci and streptococci may be isolated from a diabetic foot infection, it is important to provide coverage for Staphylococcus aureus unless the absence of this organism can be confirmed. Semisynthetic penicillins such as nafcillin or oxacillin are excellent drugs for the treatment of methicillin-sensitive Staphylococcus aureus (MSSA). Although cefazolin can also be used, it may suffer from an inoculum effect that results in clinical failure despite apparent in vitro susceptibility. Vancomycin has emerged as the mainstay for empiric antimicrobial coverage in this setting because of the prevalence of methicillin-resistant Staphylococcus aureus (MRSA). Vancomycin also has activity against streptococci and most isolates of Enterococcus faecalis. Monitoring the serum concentration of vancomycin ensures that the level of drug falls within an optimal therapeutic window and provides a means for adjusting the dose in patients with renal insufficiency.
Linezolid is an oxazolidinone with activity against gram-positive organisms such as staphylococci (including both MSSA and MRSA isolates), streptococci, and enterococci including vancomycin-resistant isolates (VRE). Linezolid has 100 % bioavailability after oral administration. The drug has FDA indications for complicated skin and soft-tissue infections, including diabetic foot infections. Itani compared outcomes of patients with skin and soft-tissue infections receiving vancomycin versus linezolid [25]. Linezolid was associated with a shorter length of stay and duration of antibiotics. Another study compared oral linezolid to vancomycin on patients requiring surgical intervention for complicated skin infections due to MRSA [26]. Linezolid achieved a higher clinical cure rate compared to vancomycin which was associated with more treatment failures and amputations. Although linezolid does not have an FDA indication for osteomyelitis, it does penetrate the bone.
Daptomycin is a lipopeptide with bactericidal activity against gram-positive organisms including MRSA, MSSA, streptococci, enterococci, and VRE. Administered daily, the drug requires weight-based dosing and only comes in a parenteral formulation. Using a rat model of chronic osteomyelitis, Rouse found that parenteral daptomycin or vancomycin significantly decreased the number of bacteria in the bone surrounding the infection site [27]. Another study used microdialysis to investigate the ability of daptomycin to penetrate the soft tissue and bone in diabetic patients with foot infections [28]. They found that multiple administrations of daptomycin achieved a sufficient free concentration in adipose tissue and bone to eradicate gram-positive organisms in diabetic foot infections including those complicated by osteomyelitis.
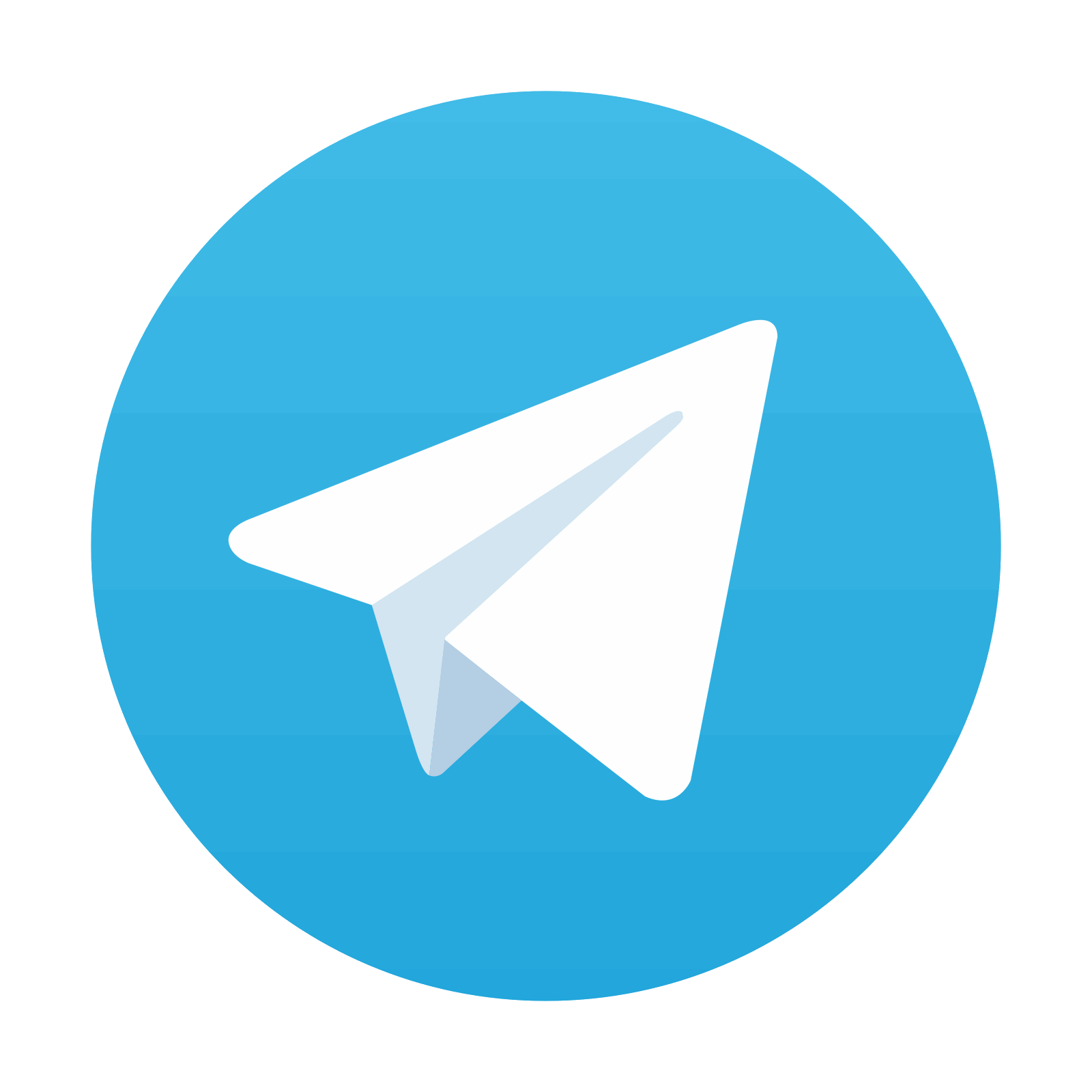
Stay updated, free articles. Join our Telegram channel

Full access? Get Clinical Tree
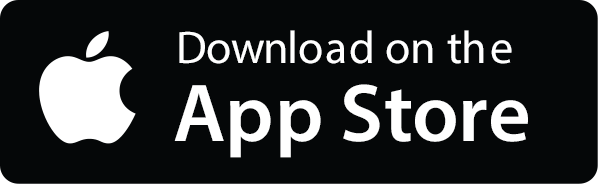
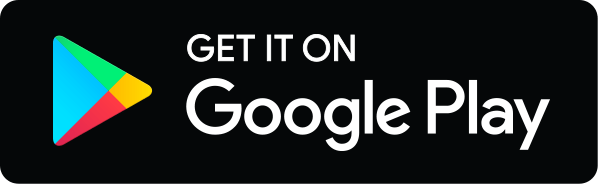