Development and Functional Maturation of the Cardiac Conduction System
Aleksander Sizarov
Antoon F.M. Moorman
Arthur S. Pickoff
In this chapter, current concepts regarding the formation of the cardiac conduction system, along with developmental aspects of cardiac electrophysiology, are summarized. Genetic regulation of the early specification of the conduction system and the electrophysiologic characteristics of the maturing heart are discussed in conjunction with morphologic considerations.
Recognition of the Conduction Tissues in the Postnatal Heart
All cardiac muscle cells possess the capacity to conduct, making the term “conduction system” a bit ambiguous. A small subset of cardiomyocytes, nonetheless, has specific electrophysiologic properties, accompanied by a distinct cellular morphology and pattern of gene expression. These cardiomyocytes make up the so-called specialized conduction system of the heart. The conduction system consists of the sinus node, the atrioventricular (AV) node, the AV bundle (known better in the clinical setting as the bundle of His), its branches, and the ventricular network of Purkinje fibers (Fig. 18.1A). Each time the heart beats, contraction is triggered by a wave of electrical activity spontaneously generated in the sinus node. The depolarizing impulse propagates rapidly through the atrial chamber myocardium, to be collected and delayed in the AV node, and again spreads very rapidly to the ventricular muscular mass through the bundle of His, its branches, and the ramifications of the Purkinje fibers. Comprehensive histologic descriptions of the cardiac nodes and the fast-conducting tracts were published over a century ago (1,2,3,4) and have served as the “golden standard” for the identification of the specialized conduction tissues.
The characteristic aggregation of the cardiomyocytes at specific locations, either as nodes or as tracts, makes it possible to recognize them as anatomic components of the conduction system by routine histology (Fig. 18.1B). In the human heart, the sinus node (SN) occupies a subepicardial position at the lateral aspect of the junction of the superior caval vein (SCV) and right atrium (RA) (5). The node characteristically is horseshoe shaped in the fetus, and usually assumes a more spindle-like shape with development. By light microscopy, the cells of the SN morphologically are distinct from the surrounding atrial myocardium; they are smaller, more compact, and contained within a fibrous matrix (6). A tail of nodal cells extends inferiorly from the SN along the terminal crest of the atrium. The AV node is located within the right atrial wall at the apex of the triangle of Koch, which is bordered by the septal leaflet of the tricuspid valve, the tendon of Todaro, and the mouth of the coronary sinus (7). Histologically, the AV node consists of a zone of loosely arranged “transitional” atrial cells that blend and extend into the surrounding atrial myocardium and, adjacent to the central fibrous body, a compact zone of closely grouped small cells (8). Two inferior extensions from the compact zone have been described that extend toward the hinges of the mitral and tricuspid valves (9,10). The compact zone of the AV node continues to penetrate the central fibrous body, at which point it contacts the bundle of His. Subsequent to penetrating the central fibrous body, at the crest of the muscular portion of the ventricular septum and beneath the membranous septum, the bundle of His gives rise to the right and left bundle branches (1,8,11). These then, course along the surface of the ventricular septum toward the apex of the heart as muscular tracts insulated from the rest of the ventricular myocardium by fibrous tissue. The left bundle branch divides further into three fascicles. Under light microscopic inspection, the cells of the bundle branches appear slightly larger than the surrounding myocardial cells. The terminations of the bundle branches continue as a widespread network of Purkinje fibers, which in the human heart are little different from the adjacent working cardiomyocytes. They are subendocardially localized, and branch into small transmural ramifications (12). The so-called AV ring bundles (13), and septal and retroaortic root branches (14,15), generally are not considered as components of the definitive AV conduction system in the normal adult heart. They still can be traced, however, in neonatal human
hearts (Fig. 18.2), and can persist as histologically recognizable structures. In rare circumstances, these remnants may provide the substrate for some forms of ventricular preexcitation in otherwise normally structured hearts (16). Additionally, in the setting of complex congenital malformations, these remnants may constitute the AV conduction axis at aberrant locations (see below).
hearts (Fig. 18.2), and can persist as histologically recognizable structures. In rare circumstances, these remnants may provide the substrate for some forms of ventricular preexcitation in otherwise normally structured hearts (16). Additionally, in the setting of complex congenital malformations, these remnants may constitute the AV conduction axis at aberrant locations (see below).
The sporadic appearance of individual cardiomyocytes resembling so-called Purkinje cells in the atrial musculature between the cardiac nodes, and in the pulmonary venous sleeves, caused some authors to conclude that these cells constitute specialized conduction tissue at these ectopic locations (17,18). In the postnatal heart, however, the preferential conduction that exists within the atrial musculature is explained by the orientation of the cardiomyocytes, rather than the existence of specialized internodal tracts (19). As discussed later, the maintenance of the primary myocardium phenotype, or insufficient maturation of the working myocardium of the terminal crest, the sleeves around the pulmonary veins, and the ventricular outflow tracts (OFTs) during cardiac development could provide the substrates for abnormal automaticity in the postnatal heart. In terms of gross histology,
however, these areas are indistinguishable from the remainder of the working myocardium (20,21,22).
however, these areas are indistinguishable from the remainder of the working myocardium (20,21,22).
The Sinus Node
Initiation of the Heart Beat
Subsequent to its formation, the primitive heart tube immediately commences regular waves of peristaltic contractions. At the beginning, the initiation of contraction is observed in the middle of the straight heart tube (23), where excitation–contraction coupling of the cardiomyocytes has progressed sufficiently to produce active shortening of the myofibrils. Studies in chicken embryos using voltage-sensitive dyes detecting spontaneous electrical depolarization have demonstrated that pacemaker activity can be identified along the whole primary heart tube prior to any contractile activity (24). However, the earliest spontaneous pacemaking activity always is located at the inflow of the primary heart tube (25) (Fig. 18.3). During further development, the pacemaking activity in already differentiated myocardium is suppressed, while newly added myocardium at the venous pole assures this site remains the dominant pacemaker site (25,26), ensuring efficient unidirectional pumping of the blood. The pacemaking mechanism in the adult SN is very complex, and involves multiple currents through different ion channels (27). It is still incompletely characterized in the embryonic heart. Very early in embryonic life, prior to the development of true pacemaker ion current(s), shuttling of calcium in and out of the sarcoplasmic reticulum through an inositol triphosphate–dependent mechanism may be responsible for pacemaker activity (28). Mouse embryos that are deficient for the hyperpolarization-activated cyclic nucleotide-gated cation channel 4 (HCN4), the major isoform producing the If (“funny” current), have extremely low heart rates and die in utero (29), suggesting an important role of If in the pacemaking mechanism of the embryonic heart.
Development of the Sinus Node
There are numerous comprehensive descriptions of the morphologic changes during development of the SN in the human embryonic and fetal heart (30,31,32,33,34). After the venous sinus has shifted to the right, the walls of its right lateral part become muscular and thickened. This thickened structure, which may be considered as the sinus nodal primordium, is traceable at its specific location along the right side of the sinuatrial junction until the end of the embryonic period, when it already begins to resemble the SN of the postnatal heart (34). Thus, early investigations of the developing SN in mammalian and human embryos were, of necessity, limited to search for clusters of histologically “specialized” cardiomyocytes at the location they occupy in the postnatal heart. Although these studies were based on comparable histologic stainings, they led to very different hypotheses about its development. One such hypothesis claimed the SN to be developed in the human from a so-called sinuatrial ring, which assumed the presence of “specialized” rings of myocardium between segments of the primary heart tube, which, in turn, were believed to develop into the distinct parts of the definitive conduction system (32,33). Numerous molecular studies in experimental animals, and in human hearts, have revealed that this is not the case. The primary heart tube, although consisting of cardiomyocytes, which, due to their primitive phenotype, all possess pacemaker activity (23,35), does not contain the precursors of the cells destined to form the definitive sinus node, as at this stage the cardiomyocytes forming the systemic venous sinus have not yet been added to the heart (see Chapter 1).
From analyses of various transgenic mouse models, it is known that correct expression of several transcription factors is needed for the formation of the SN at its normal location (36,37) (Fig. 18.4). In a pattern strictly complementary to the expression of Nkx2-5 within the developing atrial chambers, the mesenchymal cells at the caudal ventral side of the inflow tract express the T-box transcription factor Tbx18, which drives them to differentiate into cardiomyocytes forming sinus muscle, and ultimately the sinus node (36,38). Genetic lineage analyses have provided strong evidence for the origin of the entire venous sinus from these Tbx18-expressing cardiac progenitor cells. Taking into account that these cells are the precursors of the sinus node, this finding is consistent with the previous observation that the elongating heart tube shows an increase in beat rate (39). Furthermore, Tbx18-deficient mice fail to form the large head component of the sinus node, demonstrating that Tbx18 is crucial for the recruitment of inflow region mesenchyme into the myocardial lineage, which is essential for the formation of the SN (40). Early specification of the sinus nodal primordium in the mouse embryonic heart is regulated by another T-box transcription factor Tbx3 (41), which is expressed in the human embryonic heart in an almost identical pattern. Tbx3 represses the expression of the fast-conducting connexins 40 and 43, thus allowing newly added sinus myocardium to escape from further differentiation toward working myocardium (Fig. 18.5). Forced expression of Tbx3 in the atrial myocardium of the postnatal mouse heart leads to the development of ectopic functional pacemaker tissue, thus identifying Tbx3 as a key regulator of the sinus nodal phenotype (41). The suppression of the formation of the SN at the left side of the sinuatrial junction in the mouse heart is regulated by the transcription factor Pitx2c (36), which, in vertebrates, plays a crucial role in the establishment of the left/right asymmetry of the inner organs, including the heart (42). Accordingly, dual sinus nodes are present in Pitx2c-deficient mice and in humans with right isomerism of atrial appendages (43).
During the midstages of human and mouse gestation, the expression of HCN4 and pacemaker activity becomes confined to the SN itself by an as yet undiscovered mechanism. The myocardium derived from the sinus muscle, with the exception of the SN itself, matures to produce a phenotype comparable to that of the atrial working myocardium, including upregulated expression of connexins 40 and 43, and downregulated expression of HCN4 (36). The failure of complete “atrialization” of this myocardium in some individuals could explain the presence of ectopic automaticity, and the initiation of ectopic atrial tachycardias.
The Av Conduction Axis and Ventricular Conduction System
Development of the AV Conduction System
The myocardium of the primary heart tube is characterized by slow transmission of the electrical impulse (44). With ongoing development, the primary myocardium at specific locations along the outer curvature of the looping heart tube begins to further differentiate and expand to form the atrial and ventricular chambers, which are characterized by fast conduction of the electrical impulse, and matching synchronous contractions (45). The ballooning chambers remain flanked by the primary myocardium of the venous sinus, inner curvature, AVC and OFT, which temporarily escapes further differentiation, and retains the more primitive phenotype displaying high automaticity, slow conduction, and long-lasting contractions (44). The different components of the definitive AV conduction system are, in fact, formed in situ from this primary myocardium (46). The hypothesis that the conduction system may have contributions
from cardiac neural crest cells (47,48,49) has not been supported by overt evidence. In chicken embryos, retrovirus-labeled neural crest cells are not identified in the definitive conduction system (50), demonstrating that the entire conduction system has a myogenic origin. The cells derived from the neural crest do, however, differentiate in part into the autonomic nerves, which, in turn, influence the conduction properties through the AV conduction axis (51).
from cardiac neural crest cells (47,48,49) has not been supported by overt evidence. In chicken embryos, retrovirus-labeled neural crest cells are not identified in the definitive conduction system (50), demonstrating that the entire conduction system has a myogenic origin. The cells derived from the neural crest do, however, differentiate in part into the autonomic nerves, which, in turn, influence the conduction properties through the AV conduction axis (51).
In the embryonic heart it is not possible to recognize the distinct components of the AV conduction axis as can be visualized in the postnatal heart. At such an early stage of development, as in adult lower vertebrates, the common atrium still is in muscular continuity with the developing ventricles through the AVC musculature (52). Nonetheless, the chamber-forming heart already displays coordinated atrial and ventricular contractions and a proper AV delay, which is reflected in the nearly “adult-like” electrocardiogram (53) (Fig. 18.6). Investigators at the beginning of the previous
century attempted to identify the precursors of the AV conduction system in the human embryonic heart (30,31,32,54,55,56,57,58,59). Since standard histology does not permit the unambiguous identification of different tissue types, it is not surprising that their conclusions were far from comparable. The AV node was claimed to represent a part of the original AVC, and it was suggested that the bundle of His arose from the nodal tissue by a process of active growth (30). Others hypothesized that the development of the AV node occurred through further specialization of an earlier appearing bundle of His (31). Still others postulated that the formation of the AV conduction system occurred through fusion of different parts of the “specialized” myocardial rings (32).
century attempted to identify the precursors of the AV conduction system in the human embryonic heart (30,31,32,54,55,56,57,58,59). Since standard histology does not permit the unambiguous identification of different tissue types, it is not surprising that their conclusions were far from comparable. The AV node was claimed to represent a part of the original AVC, and it was suggested that the bundle of His arose from the nodal tissue by a process of active growth (30). Others hypothesized that the development of the AV node occurred through further specialization of an earlier appearing bundle of His (31). Still others postulated that the formation of the AV conduction system occurred through fusion of different parts of the “specialized” myocardial rings (32).
Studies on the changes in expression of neural tissue antigen Gln2 during human cardiac development provided much insight into the development of the proper AV and ventriculoarterial arrangements (see Chapter 1), and, importantly, into the development of the AV conduction system (60). Although the functional significance of the expression of this antigen in the developing heart is still unknown, this marker has demonstrated that the entirety of the AV conduction axis develops from the primary ring myocardium consisting of the AV canal myocardium and the myocardium of ventricular septum, which give rise to the AV node and bundle of His, respectively, as discussed below. The transcriptional repressors Tbx2 and Tbx3 are crucial molecular factors to suppress the further differentiation of the AV canal myocardium (61,62). The combined action of the widely expressed transcription factors Nkx2-5, Tbx5, and Id2 (inhibitor of DNA binding), the latter having a more limited domain of expression, is now known to be crucial for the development of the AV conduction system in the mouse heart (63). In addition, recent lineage analyses in the mouse have provided unambiguous evidence that structures, such as the coronary sinus, epicardium, vestibular spine, and ventricular septal myocardium, do not contribute to the formation of the AV node (64), as had been suggested previously (65,66) (Fig. 18.7). Current studies support the view that the embryonic AV canal, although proliferating very slowly, sufficiently grows to provide the precursor cells for the AV node, AV ring bundles, and lower atrial rims. The same lineage studies also showed that the so-called lower nodal cells of the mouse heart and the ventricular parts of the AV conduction system, including the bundle of His, bundle branches, and the septal branch are not derived from AV canal myocardium, but have a ventricular origin (69). A cross-comparison of the genome-wide expression profiles of the cardiomyocytes from the AVC at E10.5 and the AV node at E17.5 in mouse revealed that the majority of differentially expressed transcripts at the E10.5 AVC had maintained this expression profile at the E17.5 AV node. Moreover, the AV nodal cells showed an extensive neurogenic gene expression profile, which is significantly different from that of the AVC, demonstrating that the AV node substantially specializes during further development (67).
Whereas the AV node differentiates from the AVC myocardium, the bundle of His and its branches, are formed from the cardiomyocytes of the developing ventricular septum. In the postnatal heart, the myocytes making up the fast-conducting bundle of His and its branches are distinct from the slow-conducting AV nodal myocytes in terms of their differential expression of gap junction and ion channel proteins. Although in the embryonic or fetal heart the precursors of the bundle of His and its proximal branches are present from the outset of ventricular septation, they initially are not able to rapidly propagate the electrical impulse, because they still possess comparable electrophysiologic and molecular characteristics as the AV node (68). Thus, despite their distinct developmental origins, the developing human AV node and bundle of His, express similar sets of molecular markers, including Tbx3 and HCN4, and both initially are negative for fast-conducting connexins 40 and 43 (Fig. 18.8). A variety of
origins of the bundle of His have been proposed (69), but it is generally agreed that the bundle forms in situ, by differentiation of the cardiomyocytes on the crest of the developing ventricular septum (46). From the outset, the crest of the ventricular septum is in direct continuity with the myocardium of the AVC dorsally and ventrally, albeit during normal heart development, only the dorsal myocardial continuity further develops into the penetrating bundle of His (69). Along with many other transcription factors Tbx3, once more, plays an important role in this process (70) (Fig. 18.9). Thus, Tbx5 and Nkx2-5 act synergistically within the transcriptional network of the developing bundle of His by cooperatively activating expression of the transcriptional repressor Id2. Cellular birth-dating studies suggest that this factor governs the slowing of proliferation of the cardiomyocytes making up the bundle of His and its branches (63). Interestingly, distinct gene expression programs appear to regulate early versus late development of the bundle of His (71). During early bundle formation, expression of the fast-conducting connexins 40 and 43, and ANF is repressed by Tbx3 in the cells making up the developing bundle. During late fetal stages, however, the expression of connexin 40 and ANF is induced while maintaining the repression of connexin 43, despite the ongoing expression of Tbx3 in the bundle of His. The mechanisms by which this fascinating selective gene regulation is achieved remain to be elucidated.
origins of the bundle of His have been proposed (69), but it is generally agreed that the bundle forms in situ, by differentiation of the cardiomyocytes on the crest of the developing ventricular septum (46). From the outset, the crest of the ventricular septum is in direct continuity with the myocardium of the AVC dorsally and ventrally, albeit during normal heart development, only the dorsal myocardial continuity further develops into the penetrating bundle of His (69). Along with many other transcription factors Tbx3, once more, plays an important role in this process (70) (Fig. 18.9). Thus, Tbx5 and Nkx2-5 act synergistically within the transcriptional network of the developing bundle of His by cooperatively activating expression of the transcriptional repressor Id2. Cellular birth-dating studies suggest that this factor governs the slowing of proliferation of the cardiomyocytes making up the bundle of His and its branches (63). Interestingly, distinct gene expression programs appear to regulate early versus late development of the bundle of His (71). During early bundle formation, expression of the fast-conducting connexins 40 and 43, and ANF is repressed by Tbx3 in the cells making up the developing bundle. During late fetal stages, however, the expression of connexin 40 and ANF is induced while maintaining the repression of connexin 43, despite the ongoing expression of Tbx3 in the bundle of His. The mechanisms by which this fascinating selective gene regulation is achieved remain to be elucidated.
Developmental Mechanisms Underlying the Formation of Accessory AV Pathways
In the normal human adult heart, there are no muscular connections between the atria and ventricles other than the AV conduction axis, which is responsible for the delay of impulse propagation from the atria to the ventricles, this occurring in the AV node, and the uniform and rapid distribution of the impulse to the ventricular chambers through the bundle of His and its branches. With exception of the muscular connection through the bundle of His, the AV myocardial continuity is essentially interrupted at approximately 12 weeks of human development (52). In the fetal and early neonatal human heart, however, multiple tiny myocardial strands have been observed crossing the otherwise fully formed plane of AV insulation (15,72). Persistence of these remnants of the AVC musculature was initially suggested to be the substrate for electrical preexcitation of the ventricles, which may result in the Wolff–Parkinson–White syndrome, the most prevalent type of supraventricular tachyarrhythmias in young children (73). An important characteristic of these bundles is their high conductivity due to the expression of fast-conducting connexin 43 (74), which can lead in specific circumstances to life-threatening arrhythmias. The myocardial strands found in normal neonatal hearts, however, are derived from the AVC, and our own studies, as yet unpublished, suggest they retain the AVC phenotype and are negative for connexins 40 and 43, making it unlikely that the mere failure of their disappearance is sufficient to explain the phenomenon of ventricular preexcitation with rapid AV conduction. They are distinctly different types of pathways.
The Bmp-receptor 1a, also known as Alk3, and the transcription factor Tbx2 have been shown to play an important role in the correct formation of the plane of insulation on the left side of the mouse heart (75,76). In the absence of myocardial Alk3 or Tbx2 expression, not only does connective tissue fail to form between the atrial and ventricular chambers, but also the persisting myocardial strands do become fast conducting, and are thus capable of causing preexcitation. The formation of accessory pathways with fast conduction properties, therefore, must involve both abnormalities in the development of the fibrous insulation and the differentiation of the originally slow-conducting AVC myocardium into fast-conducting working myocardial phenotype.
The bundle of His, which remains the only muscular connection between the atrial and ventricular chambers after completion of the formation of the insulation plane, becomes gradually isolated from
the myocardium of the ventricular septum by a connective tissue sheath originating, most probably, from the epicardially derived tissues of the AV grooves. In fetal hearts, the cardiomyocytes of the AV node and bundle of His remain in contact with the myocardium of the ventricular septum (Fig. 18.10). Persistence of these connections between the AV node or bundle of His and the ventricular myocardium during further maturation in the fetal period may constitute the slow-conducting substrate of one of the variants of so-called Mahaim-type reentry tachycardia, although similar slow-conducting AV connections can be demonstrated along the entire right AV ring, likely representing persisting remnants of the AV ring tissues (16).
the myocardium of the ventricular septum by a connective tissue sheath originating, most probably, from the epicardially derived tissues of the AV grooves. In fetal hearts, the cardiomyocytes of the AV node and bundle of His remain in contact with the myocardium of the ventricular septum (Fig. 18.10). Persistence of these connections between the AV node or bundle of His and the ventricular myocardium during further maturation in the fetal period may constitute the slow-conducting substrate of one of the variants of so-called Mahaim-type reentry tachycardia, although similar slow-conducting AV connections can be demonstrated along the entire right AV ring, likely representing persisting remnants of the AV ring tissues (16).
Development of the Ventricular Conduction Network
In the postnatal heart, the electrical impulse, after being delayed in the AV node, is transmitted via the ventricular conduction network consisting of the bundle branches and Purkinje fibers. The main function of the ventricular conduction network is the rapid propagation and uniform distribution of the impulse to the ventricular muscle mass. The fast-conducting properties of the ventricular conduction system (VCS) are due to the high expression of connexins 40 and 43, which form gap junctions with high conductance properties (77). As with the ventricular working myocardium, the cardiomyocytes of the postnatal VCS are specialized in fast conduction. Unlike the working myocytes, however, they have a poorly developed contractile apparatus, and even display some degree of automaticity, resembling the embryonic primitive phenotype (46). In embryonic hearts that do not have discernible bundle branches or a Purkinje fiber network, fast conduction within the developing ventricles already is present at stages when the trabeculations have appeared. It has been established that the development of the mature pattern of ventricular activation and formation of the Purkinje fiber network are closely linked to the development of the ventricular trabeculations (50). The cavities of the ventricles in the early embryonic heart contain an extensive meshwork of trabeculations, which attach to the thin outer ventricular wall, and, similar to the myocytes making up the mature Purkinje fibers, express the fast-conducting connexins 40 and 43 (31,63,78). Thus, there is a molecular substrate for preferential rapid conduction of the electrical impulse through the ventricular trabeculations to the ventricular musculature in the embryonic heart even without a fully differentiated true ventricular conduction network.
The observation that the so-called Purkinje myocytes express, along with myocardial markers, markers reminiscent of neuronal cells has resulted in much debate about the origin of the VCS. Most of the data regarding the formation of the VCS have come from studies in chicken embryos, where fate-mapping experiments have unequivocally demonstrated that the entire conduction system has a myocardial origin (50). Individual ventricular myocyte precursor cells give rise to a series of progeny that migrate preferentially vertically to form the meshwork of trabeculations (79,80). From mouse studies, it is known that neuregulin-1 and Notch signaling are necessary and sufficient to form the ventricular trabeculations by regulating the relative proportion of the embryonic ventricular cardiomyocytes that form the trabecular and compact myocardium (81,82). Another signaling molecule, endothelin-1, secreted by endothelial cells covering the ventricular trabecular myocardium, probably in response to increasing biomechanical forces such as shear stress and pressure in the walls of the ventricular chambers, has been shown to play an important role in the induction of the Purkinje fiber network in the chicken embryonic heart (83,84). Current models of the development of the VCS involve neuregulin signaling-mediated induction and formation of
ventricular trabecular myocardium and endothelin signaling-mediated differentiation of subendocardial myocytes into Purkinje myocytes (46,50,85) (Fig. 18.11), with recent lineage studies in the mouse supporting a biphasic mode of formation of the VCS (86). Several transcription factors have been shown to play a role in the regulation of the development of rapid conduction within the Purkinje cardiomyocytes by affecting the expression of connexin 40. Expression of this rapid-conducting gap-junctional protein in the peripheral VCS is activated by homeodomain-only protein (Hopx), possibly through the Nkx2-5–dependent mechanism (87). Transcription factor HF-1b, also being expressed in the VCS, upregulates connexin 40 and downregulates expression of accessory potassium channel protein minK. Disruptions of correct connexin 40 expression have been reported in distal, but not proximal His–Purkinje conduction tissues of HF-1b knockout mice, which develop normally and survive to term, but subsequently succumb to lethal ventricular tachyarrhythmias (88,89). Another transcription factor, Irx3, also regulates formation and function of the His–Purkinje system (90). However, the impact of these factors on downstream gene regulation within the VCS remains to be clarified.
ventricular trabecular myocardium and endothelin signaling-mediated differentiation of subendocardial myocytes into Purkinje myocytes (46,50,85) (Fig. 18.11), with recent lineage studies in the mouse supporting a biphasic mode of formation of the VCS (86). Several transcription factors have been shown to play a role in the regulation of the development of rapid conduction within the Purkinje cardiomyocytes by affecting the expression of connexin 40. Expression of this rapid-conducting gap-junctional protein in the peripheral VCS is activated by homeodomain-only protein (Hopx), possibly through the Nkx2-5–dependent mechanism (87). Transcription factor HF-1b, also being expressed in the VCS, upregulates connexin 40 and downregulates expression of accessory potassium channel protein minK. Disruptions of correct connexin 40 expression have been reported in distal, but not proximal His–Purkinje conduction tissues of HF-1b knockout mice, which develop normally and survive to term, but subsequently succumb to lethal ventricular tachyarrhythmias (88,89). Another transcription factor, Irx3, also regulates formation and function of the His–Purkinje system (90). However, the impact of these factors on downstream gene regulation within the VCS remains to be clarified.
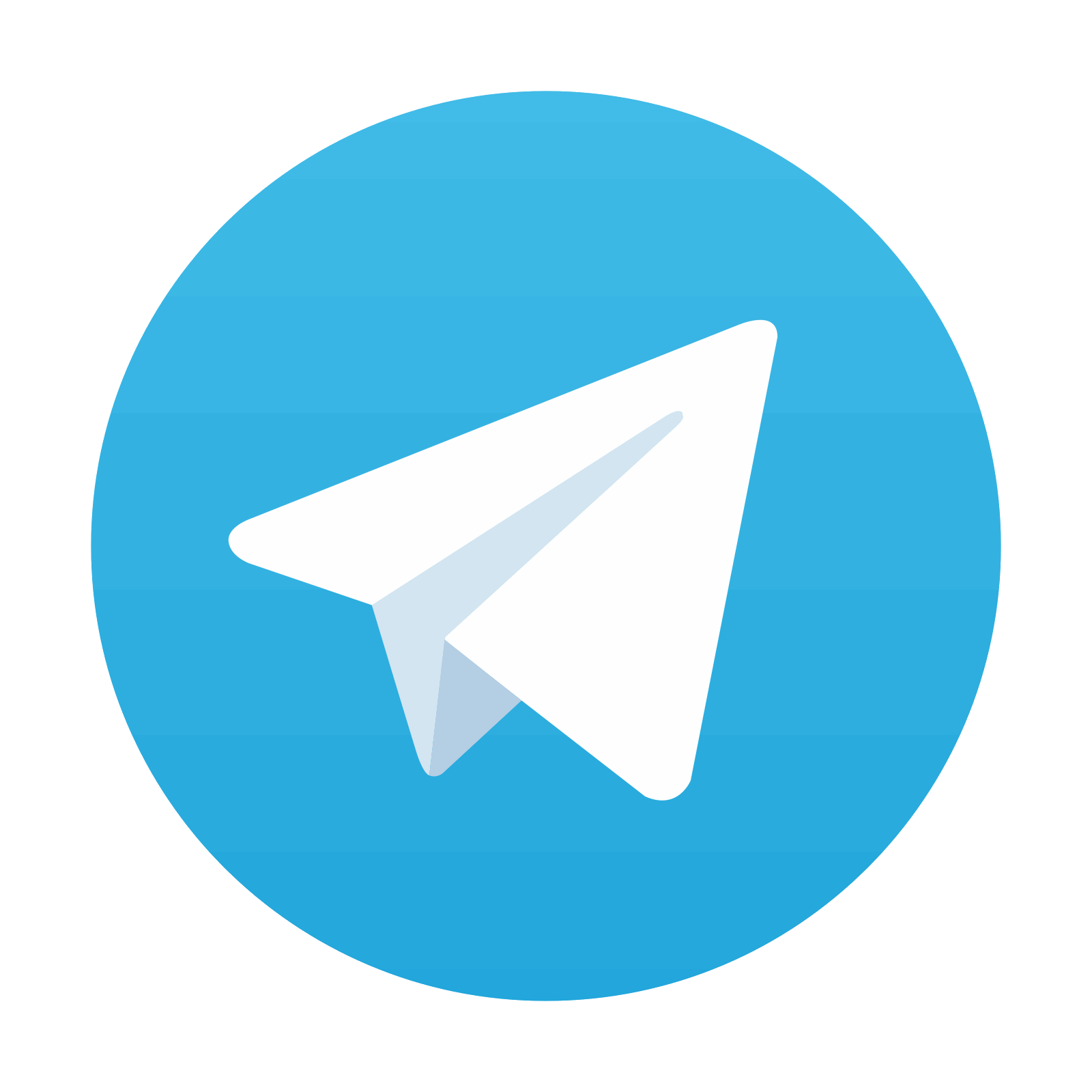
Stay updated, free articles. Join our Telegram channel

Full access? Get Clinical Tree
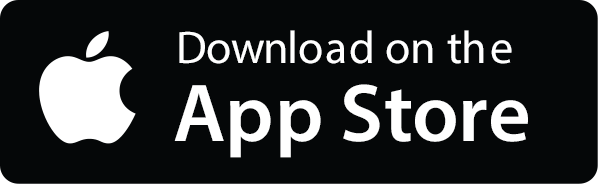
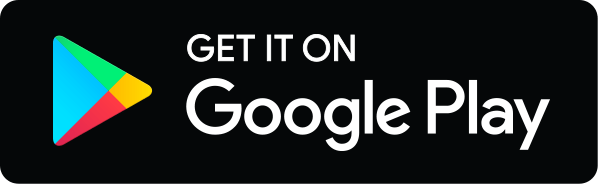