Acquired
Congenital
Age
Antithrombin deficiencies
Malignancy
Proteins C & S deficiencies
Surgery/trauma
Factor V Leiden
Immobilization
Prothrombin 20210A
Oral contraceptives
Blood group non-O
Hormone replacement therapy
Hyperhomocysteinemia
Pregnancy
Factor XI, IX, VII, VIII, X, and II excess
Obesity
Dysfibrinogenemia
Neurological disease
Dysplasminogenemia
Cardiac disease
Plasminogen activator inhibitor-1
Antiphospholipid antibodies
Reduced activity of heparin cofactor II
When a DVT occurs in a patient with no known risk factors, the diagnosis is considered an idiopathic DVT presumably provoked by a transient risk factor such as surgery or trauma. In a prospective, multicenter registry of 2,119 patients with venous thromboembolism (VTE), 43 % were deemed to have idiopathic DVT [15]. Approximately 42 % of the patients with idiopathic DVT had temporary risk factors with immobilization (15 %), surgery (14.4 %), and severe medical illness (8.2 %) occurring most frequently.
Most patients have more than one thrombotic risk factor at the time of their presentation. Fifty percent of thrombotic events in patients with inherited thrombophilia occur in the presence of an additional acquired risk factor (e.g., surgery, prolonged bed rest, pregnancy, oral contraceptives). Some patients have more than one form of inherited thrombophilia or more than one form of acquired thrombophilia and appear to carry an even greater risk for thrombosis. In a population-based study, 53 % of patients with VTE had three or more of the following risk factors: >48 h of immobility in the preceding month, hospital admission, surgery, malignancy, infection in the past 3 months, or current hospitalization [16].
Genetic Risk Factors
The most frequent causes of an inherited (primary) hypercoagulable state are factor V Leiden mutation, prothrombin gene mutation, protein S deficiency, protein C deficiency, antithrombin deficiency, and dysfibrinogenemia. The pathophysiology and genetics of each of those disorders are beyond the scope of this chapter; however, their associated thrombotic risk has been assessed in two ways: evaluation of patients with DVT and evaluation of families with thrombophilia [17]. In a Spanish study of 2,132 consecutive unselected patients with VTE, 12.9 % had an anticoagulant protein deficiency (7.3 % with protein S deficiency, 3.2 % with protein C deficiency, and 0.5 % with antithrombin deficiency) [18].
Acquired Risk Factors
According to the previously cited population-based study, many patients with an episode of VTE have more than one acquired risk factor for thrombosis [16]. The most prevalent preexisting medical characteristics include the following: greater than 48 h of immobility in the preceding month (45 %), hospital admission (39 %), surgery (34 %), malignancy (34 %), infection in the past 3 months (34 %), and current hospitalization (26 %). Of the 587 episodes of VTE, only 11 % had no preexisting medical characteristics present, while 36 and 53 % had one to two and three or more risk factors, respectively.
Malignancy
Patients with cancer often harbor a hypercoagulable state due to the production of substances with procoagulant activity (e.g., tissue factor and cancer procoagulant). The risk of VTE in such patients peaks during the initial hospitalization and onset of chemotherapy, as well as at the time of disease progression [19]. Approximately 20 % of patients with symptomatic DVT have a known active malignancy. In a retrospective study of over 63,000 patients admitted to Danish nonpsychiatric hospitals from 1977 to 1992 for a diagnosis of VTE, 18 % had received a diagnosis of cancer (other than non-melanoma skin cancer) prior to the thromboembolic event [20]. The majority of cancers associated with thromboembolic events are clinically evident and have been previously diagnosed at the time of the event. In the Danish study, 78 % of the cancers were diagnosed before the event with the most common sites being lung (17 %), pancreas (10 %), colon and rectum (8 %), kidney (8 %), and prostate (7 %) [20].
Surgery
Thrombotic risk increases during surgery, particularly orthopedic, neurologic, and cancer surgery [21–23]. The 2012 American College of Chest Physicians (ACCP) guidelines estimate that patients undergoing orthopedic surgery have a 2.80 % risk of having a symptomatic thrombotic event if untreated with initial thromboprophylaxis during the first 2 weeks following surgery, with a cumulative 35-day risk of 4.3 % if untreated during the entire perioperative period [24].
The ACCP guidelines for nonorthopedic surgery stratify patients into categories based on their estimated untreated VTE risk [25]. Outpatient or same-day surgery patients make up the very low-risk category (<0.5 % VTE risk in the absence of thromboprophylaxis). The low-risk category (1.5 % VTE risk) applies to patients having spinal surgery for nonmalignant disease. Patients undergoing gynecologic (noncancer), cardiothoracic surgery, and those having spinal surgery for malignant disease fall into the medium-risk category. The high-risk category (6 % VTE risk) includes patients having bariatric surgery, surgery for gynecologic cancer, a craniotomy, and major trauma surgery.
Trauma
The risk of thrombosis increases after all forms of major trauma [26–29]. A study of 716 patients admitted to a regional trauma unit found lower extremity DVT in 58 % of patients with adequate venographic studies, 18 % of whom had proximal vein thrombosis [27]. Venous thrombi were detected in 54 % of patients with a major head injury, 61 % of patients with a pelvic fracture, 77 % of patients with a tibial fracture, and 80 % of those with a femoral fracture. The sequence of events that trigger activation of the coagulation system following surgery or trauma remains unclear. Conditions which favor venous thrombosis include decreased venous blood flow in the lower extremities, diminished fibrinolysis, immobilization, release or exposure of tissue factor, and depletion of endogenous anticoagulants such as antithrombin [30]. A large population-based study investigated the VTE risk following a minor injury defined as one not requiring surgery, a plaster cast, hospitalization, or extended bed rest at home for at least 4 days [31]. A minor injury occurring in the preceding 3–4 weeks increased the DVT risk three- to fivefold overall and 50-fold in carriers of factor V Leiden.
Pregnancy
Pregnancy increases the risk of thrombosis due in part to obstruction of venous return by the enlarged uterus and the hypercoagulable state associated with pregnancy. Estimates of the age-adjusted incidence of VTE range from 5 to 50 times higher in pregnant versus nonpregnant women with an absolute incidence of 1 in 500–2,000 pregnancies (0.025–0.10 %) [32, 33].
Drugs
While several drugs increase the risk of venous thrombosis, oral contraceptives have the greatest impact because of their widespread use. Among young women, oral contraceptive use represents the most important cause of thrombosis [34]. Observational studies, the HERS trials, and a meta-analysis published before the Women’s Health Initiative, identified an association between hormone replacement therapy (HRT) and venous thromboembolism (VTE). These studies reported that HRT caused an approximately twofold increase in VTE risk, which appeared to be greatest in the first year of treatment [35–38]. Other medications associated with increased risk of VTE include tamoxifen and bevacizumab.
Immobilization
Immobilization favors venous stasis which increases the risk of DVT and subsequent PE. Warlow et al. evaluated the effect of immobilization by using radiolabeled fibrinogen to study patients with unilateral lower extremity paralysis [39]. They found that DVT developed in 60 % of the paralyzed limbs compared with a DVT rate of only 7 % in the contralateral normal leg which acted as an internal control. Prolonged immobilization during air travel may increase the risk of DVT; however, its clinical impact remains unclear. A meta-analysis reported a slightly increased rate of asymptomatic DVT after long haul air travel defined as flight duration greater than 7 h. None of the reviewed studies reported any symptomatic DVTs, deaths, or pulmonary emboli [40].
Prior Episode of VTE
In an outpatient prospective cohort study, the risk of recurrence after an acute episode of venous thrombosis was 18, 25, and 30 % at 2, 5, and 8 years, respectively [42]. In a community epidemiologic study, a history of VTE conferred a relative risk (RR) of 7.9 for VTE recurrence [43]. The association between lower extremity superficial venous thrombophlebitis (SVTP) and DVT risk is less clear. A retrospective study showed a DVT rate of 2.7 % following an episode of SVTP compared to 0.2 % in control patients (OR 10.2). This relationship became less robust and lost statistical significance when the authors controlled for a previous history of VTE [41].
Antiphospholipid Antibodies
The presence of antibodies directed against phospholipid-bound plasma proteins characterizes the antiphospholipid syndrome. Patients can present clinically with venous or arterial thrombosis, recurrent fetal loss, and occasional thrombocytopenia. The disorder may be classified as primary or associated with systemic lupus erythematosus and other connective tissue diseases. In one large series, antiphospholipid antibodies were present in 4.1 % of 2,132 consecutive patients presenting with DVT [18].
Anatomic Factors Associated with Increased Risk of DVT
May-Thurner syndrome involves hemodynamically significant compression of the left common iliac vein between the overlying right common iliac artery and the underlying vertebral body. Although this anatomic pattern is common in asymptomatic subjects, May-Thurner syndrome has been implicated in patients with unprovoked left iliofemoral DVT or chronic venous insufficiency [44]. The incidence of May-Thurner syndrome peaks in women between the ages of 20 and 50, and it may be more common in those with reduced left common iliac vein diameters and/or severe degrees of iliac vein compression. Recurrent episodes of DVT may respond poorly to treatment with anticoagulation alone requiring catheter-directed thrombolysis and balloon angioplasty with intravascular stenting, especially in those with limb-threatening thrombosis.
Paget-Schroetter syndrome, also referred to as spontaneous upper extremity venous thrombosis, usually results from an underlying compressive anomaly at the thoracic outlet. Compression of the subclavian vein usually occurs between the first rib and a hypertrophied scalene or subclavius tendon or between the tendons themselves.
Diagnosis
The clinical diagnosis of DVT is unreliable because the nonspecific signs and symptoms of venous thrombosis can be easily confused with other disease processes. Lower extremity swelling, the most common physical sign of DVT, has several nonvenous causes including inflammation from infection and soft tissue injury. Furthermore, nonocclusive venous thrombus can remain asymptomatic until it embolizes or completely occludes the vein. Despite these diagnostic challenges, a thorough history and physical examination can help correctly identify DVT when it is present and rule it out when it is absent. Venous duplex ultrasound provides a noninvasive, objective tool for detecting DVT and guiding treatment.
Clinical Assessment
Wells et al. developed a clinical model to determine the probability of DVT prior to definitive diagnostic testing in symptomatic outpatients [45]. Patients were stratified into high-, moderate-, or low-probability groups based on a score derived from their clinical characteristics (Table 9.2). Applying this scoring system to 529 patients, they reported that a DVT was detected in 85 % of patients in the high pretest probability category, 33 % in the moderate, and 5 % in the low category. They also demonstrated that using a clinical model in conjunction with ultrasound would decrease the number of false-positive and false-negative diagnoses if ascending phlebography was used when the ultrasound result and pretest probability disagreed. These investigators subsequently integrated d-dimer testing with clinical probability to conserve vascular laboratory resources [46].
Characteristic | Score |
---|---|
Active cancer | 1 |
Paresis, paralysis, or recent immobilization of lower limb | 1 |
Bedridden >3 days or major surgery <4 weeks | 1 |
Localized tenderness | 1 |
Entire leg swollen | 1 |
Calf swelling >3 cm (compared with asymptomatic limb) | 1 |
Pitting edema | 1 |
Collateral superficial veins | 1 |
Alternative diagnosis as likely or greater than DVT | −2 |
In a prospective multicenter management study, Elf et al. theorized that if a patient was categorized as low pretest probability and a moderate sensitivity d-dimer test was negative, DVT could be ruled out without further diagnostic testing [47]. In a study of 110 patients, only one patient who had both low pretest probability and negative d-dimer developed an episode of venous thromboembolism within the next 3 months. Others have reported similar results in outpatients with low pretest probability score and negative d-dimer results [48]. A negative d-dimer, however, cannot be used to exclude DVT in patients with a high pretest probability score, as up to 20 % of these patients have a DVT confirmed subsequent to test results [48].
Venous Duplex Ultrasound
Venous duplex ultrasound is the diagnostic method of choice for most patients being evaluated for acute DVT. Table 9.3 lists the duplex criteria used to detect a DVT, the most important of which is compressibility of the vein being examined. Duplex ultrasound examination of a normal, patent vein demonstrates a black lumen that can be completely compressed by exerting pressure on the ultrasound probe. Acute thrombus has the same density as blood and therefore the lumen of a thrombosed vein also appears black (anechoic) by ultrasound examination. The distinguishing characteristic of an acute DVT is the inability to completely compress the vein under the probe. In the setting of an iliofemoral thrombosis, the more distal lower extremity veins may be patent but non-compressible because of the high venous pressures caused by the proximal occlusion. The sensitivity and specificity reported in any single study of venous duplex ultrasound depend on the number of isolated iliac and calf vein thromboses included in the patient sample, as these are more difficult to diagnose.
Table 9.3
Venous duplex diagnosis of acute DVT: criteria
Component | Criteria |
---|---|
B-mode imaging | Noncompressibility of vein |
Visible intraluminal thrombus | |
Dilated veins | |
Enlarged branch veins (collaterals) | |
Doppler | Loss of respiratory phasicity |
Loss of spontaneous venous signal | |
Abnormal augmentation | |
Elevated flow velocity (wind tunnel sound) in main vein | |
Elevated flow velocity in branch veins (collaterals) |
Additional sonographic signs of acute DVT include dilated veins and enlarged venous collaterals. As thrombus ages, the density increases, making it more echogenic and visible by ultrasound. A negative venous duplex examination reliably excludes DVT, and withholding anticoagulation on the basis of one or more negative ultrasound evaluations is a safe and well-established practice [49–51]. The most reliable physical sign of acute DVT is unilateral leg edema which warrants a definitive ultrasound examination. In most cases, the patient should receive empiric anticoagulation therapy if the ultrasound examination will be significantly delayed.
In the past, venous duplex ultrasound exams focused on compression of the common femoral and popliteal veins assuming that the diagnosis of calf vein DVT was unreliable and clinically inconsequential. More recent studies demonstrate that DVT frequently begins in the calf veins [52, 53] and that isolated calf DVT progresses into the popliteal vein in 25–30 % of cases [53–56]. Moreover, pulmonary emboli have been reported in 8–34 % of patients with isolated calf vein thrombosis [57]. Although the accuracy of venous duplex for detecting calf vein thrombosis varies, some reports cite sensitivity and specificity rates of 90 and 100 %, respectively [58, 59].
The diagnostic sensitivity of venous ultrasound may vary between symptomatic and high-risk asymptomatic patients. In a study of postoperative orthopedic patients, 24 % of symptomatic and 88 % of asymptomatic patients had isolated calf vein thrombosis [60]. In the symptomatic group, venous duplex ultrasound had 85 % sensitivity and 86 % specificity, but in the asymptomatic group, the sensitivity dropped to 16 % although the specificity was 99 %.
Ultrasound diagnosis of an isolated iliac vein thrombosis can be challenging. The physical finding of unilateral lower extremity edema extending distally from the inguinal ligament should raise clinical suspicion for an isolated iliac vein thrombosis. In contrast, patients with internal iliac vein thrombosis or nonocclusive thrombus of the common iliac or proximal external iliac veins often remain asymptomatic. Compressibility, the most accurate ultrasound test for DVT, cannot be used to evaluate the iliac veins or inferior vena cava because of their location in the pelvis and retroperitoneum. Diagnosing an iliac vein or caval thrombosis therefore depends on indirect ultrasound findings including color flow imaging and venous velocity profiles, in addition to the grayscale image itself. Unfortunately, patients with abdominal obesity or extensive bowel gas present technical challenges to imaging the iliac veins and cava, and up to 24 % of these patients have a nondiagnostic exam [61].
Phlebography and Venography
In the past, ascending phlebography had widespread use as a diagnostic tool for detecting lower extremity DVT. Although it required venous access in the foot and could cumbersome to perform, ascending phlebography proved to be an accurate test with high sensitivity and specificity. Weinmann and Salzman reported a 2–3 % risk of contrast-induced thrombosis following phlebography; however, the use of nonionic contrast, elevation of the leg, and flushing with heparinized saline reduces this risk considerably [62]. Ascending phlebography now plays a very limited role in diagnosing DVT having been replaced by other noninvasive imaging modalities, most notably venous duplex ultrasound.
Computerized tomographic (CT) and magnetic resonance (MR) venography have several advantages, particularly in imaging patients with a suspected pelvic DVT. Both types of cross-sectional imaging can detect abdominal, pelvic, and chest pathology (pelvic masses, abdominal or thoracic malignancies) that may be etiologically associated with the development of venous thrombosis. CT and MR venography can also show iliac vein compression resulting from the overlying iliac artery (May-Thurner syndrome). Disadvantages of CT and MR venography include radiation exposure (CT), high cost, and limited availability.
d-Dimer Tests
After factor XIII stabilizes cross-linked fibrin during clot formation, autogenous plasmin breaks down the fibrin, releasing the fragments into the blood stream. d-dimer represents one type of fragment which can be detected with a blood test [63]. A positive d-dimer test indicates the presence of thrombus; however, it cannot determine the thrombus location or its clinical significance. In practice, the d-dimer test acts as a sensitive but nonspecific diagnostic tool which has clinical utility in excluding the diagnosis of DVT in patients with low thrombotic risk. A negative d-dimer test in a patient with a low clinical suspicion results in a 99 % negative predictive value for DVT [45, 47, 64]. In this clinical situation, no further testing is required and anticoagulation can be safely withheld. Other conditions can elevate d-dimer levels including inflammation, recent surgery, infection, and pregnancy, making the d-dimer test more accurate in the outpatient setting.
The evaluation of patients with suspected venous thrombosis begins with a thorough clinical assessment to gauge the pretest probability of DVT. The d-dimer blood test along with venous duplex ultrasound provide adjunctive diagnostic tools that can help exclude or confirm the presence of DVT.
Treatment Strategies
Appropriate treatment of acute DVT can significantly improve short- and long-term patient outcomes. Several studies highlight the short-term benefit of early and sustained therapeutic anticoagulation for acute DVT. Other studies focusing on the duration of anticoagulation show that longer treatment times lower the risk of recurrent DVT. A new generation of oral anticoagulants promises greater ease of use and may eliminate some of the logistic barriers to outpatient anticoagulation therapy. The key to making sound treatment decisions is recognizing that not all venous thromboses are the same. Some patients do well with anticoagulation alone (e.g., isolated calf vein thrombosis), whereas patients with extensive iliofemoral DVT often benefit from a strategy of thrombus removal followed by effective anticoagulation. The treatment strategies outlined in the following sections are based on the level and extent of venous thrombosis, the known natural history of acute DVT, and the recognized benefits of therapy.
Importance of Anticoagulation
The goal of immediate anticoagulation with heparin is to interrupt ongoing thrombosis while long-term oral anticoagulation with warfarin attempts to prevent recurrent DVT. Brandjes et al. demonstrated the importance of initial therapeutic anticoagulation by randomizing patients to either prompt anticoagulation with heparin followed by vitamin K antagonists (VKA) or VKAs alone [65]. Patients receiving a VKA alone had recurrent thrombotic events three times more frequently than those who received heparin immediately. The authors associated this finding with the fact that patients treated with warfarin alone had a delay of several days before they reached therapeutic anticoagulation. Hull et al. confirmed the need for early therapeutic anticoagulation [66]. They reported a 24.5 % rate of recurrence in patients whose treatment with heparin failed to achieve therapeutic levels within the first 24 h, compared with a 1.6 % recurrence rate in those reaching an early therapeutic level. Current guidelines favor low-molecular-weight heparin (LMWH) over unfractionated heparin (UFH) in the initial period and advise that heparin overlap a VKA for a minimum of 5 days to ensure therapeutic anticoagulation. Studies on the duration of suggest that the longer anticoagulation is continued, the lower the risk of recurrence. Decisions on the length of treatment must always strike a balance between the risk of recurrent thrombosis and the risk of bleeding.
The emergence of new oral anticoagulants may change the paradigm of care for acute DVT. Randomized clinical trials on direct inhibitors of factor Xa (rivaroxaban, apixaban) or factor IIa-thrombin (dabigatran) show that these compounds are effective and safe in the management of patients with venous thromboembolic disease [67, 68]. The new oral anticoagulants have several advantages including the fact that they are rapidly absorbed, require no monitoring, have fewer drug and food interactions than VKAs, and do not require parenteral heparin. The enhanced safety profile and ease of use associated with the new oral agents may ultimately shift the risk-benefit analysis in favor of prolonged anticoagulation to prevent DVT recurrence [68–71].
Determining Treatment by Type of DVT
Calf Vein Thrombosis
Although patients with isolated calf vein thrombosis have the lowest thrombotic burden and the best overall prognosis, they still carry a risk of thrombus extension or thromboembolic sequelae. A randomized trial of idiopathic calf DVT compared 3 months of therapeutic anticoagulation with 5 days of anticoagulation followed by placebo. Thrombus propagated or recurred in 19 % of patients who received only 5 days of anticoagulation compared to no major thromboembolic complications observed in patients anticoagulated for 3 months [54]. Current guidelines recommend 3 months of anticoagulation for symptomatic calf DVT. For asymptomatic calf DVT, 2 weeks of serial ultrasound Doppler examinations should be performed and anticoagulation should be initiated if thrombus extension occurs [72]. We recommend treating isolated calf DVT with anticoagulation and compression stockings unless the patient is at high risk for bleeding. In patients with a high bleeding risk, we recommend compression and ambulation with serial ultrasound surveillance.
Femoral Vein Thrombosis
Patients with isolated femoral vein thrombus, particularly in the mid or upper thigh, typically do well with anticoagulation alone due to collateral venous drainage from the popliteal and profunda femoral veins to the common femoral vein. Often, functional valves exist above and below the vein segment involved with thrombus. Recanalization of the thrombus does not play a critical role as long as the popliteal and common femoral veins remain free of obstructive thrombus.
Popliteal Vein Thrombosis
Patients with occlusive femoropopliteal vein thrombosis extending into the popliteal vein “trifurcation” frequently have severe acute symptoms. Occlusion of the axial venous drainage of the calf causes distal venous hypertension and significant postthrombotic morbidity. We consider active patients with symptomatic femoropopliteal DVT to be candidates for catheter-directed thrombolysis followed by compression and 3–6 months of anticoagulation.
Iliofemoral DVT
Although a DVT in any location can result in postthrombotic complications, an iliofemoral DVT poses the threat of debilitating PTS symptoms especially when patients are treated with anticoagulation alone [4, 73]. Anatomically, the common femoral, external iliac, and common iliac veins make up the single outflow venous channel for the entire lower extremity. Thrombotic obliteration of this lone outflow channel therefore results in the highest venous pressures and the most severe postthrombotic morbidity. Labropoulos et al. studied arm-foot pressure differentials at rest and during postocclusive reactive hyperemia in postthrombotic patients who were treated with anticoagulation alone at the time of their acute DVT [74]. They found that iliofemoral DVT patients had the highest resting and hyperemic venous pressures. Qvarfordt and Eklof et al. provided objective evidence that immediate thrombus removal reduced venous pressure in patients with iliofemoral DVT [75]. They recorded lower extremity compartment pressures (a surrogate for venous pressures) in patients with acute iliofemoral DVT before and after venous thrombectomy and found very high compartment pressures preoperatively which normalized postoperatively.
Another important but generally overlooked observation in patients with iliofemoral DVT is the risk of recurrence. Douketis and colleagues found that patients with iliofemoral DVT had a greater than twofold risk of recurrent venous thromboembolism compared to patients with infrainguinal DVT (11.8 % vs. 5.2 %) [76]. Several clinical outcome studies show that recurrent DVT increases the frequency and severity of postthrombotic syndrome.
Treatment with a Strategy of Thrombus Removal
Thrombus removal attempts to minimize the clinical impact of acute DVT by reducing the risk of PTS and recurrent thrombosis. The rationale behind thrombus removal can be traced to natural history studies of acute DVT treated with anticoagulation alone. Efficient endogenous fibrinolysis, which resolved thrombus within 60–90 days, restored venous patency and preserved valve function [77]. Extended follow-up in the same patient cohort showed that patients whose thrombus did not resolve had a much higher rate of recurrent DVT [78–80].
PTS occurs in 25–46 % of patients following acute DVT and represents a major source of morbidity which significantly reduces patients’ quality of life [4, 42, 73, 81–83]. Factors that increase the risk of PTS include iliofemoral DVT, residual thrombus, and recurrent thrombosis. Thrombus removal attempts to counteract the risk factors for PTS by restoring venous patency, preserving valve function, and reducing the rate of DVT recurrence. Aziz et al. [12] reported that recurrent DVT following catheter-directed thrombolysis for iliofemoral DVT correlated with the quantity of residual thrombus remaining after treatment. Patients experiencing successful thrombolysis had significantly less recurrence than patients with >50 % of their initial thrombus burden remaining after thrombolysis.
Treatment strategies designed to eliminate thrombus from the deep veins include operative venous thrombectomy, catheter-directed thrombolysis, and percutaneous pharmacomechanical thrombolysis. An algorithm that illustrates our suggested strategy of thrombus removal is shown in Fig. 9.1.
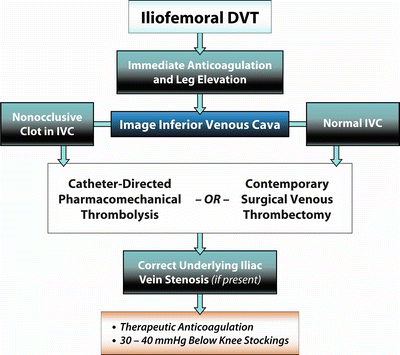
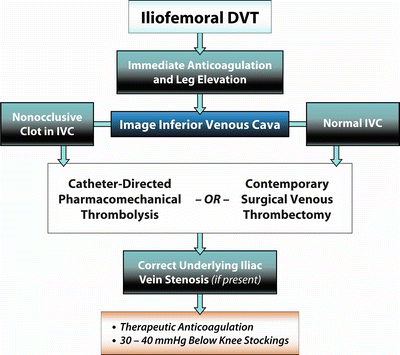
Fig. 9.1
Algorithm illustrates suggested strategy of thrombus removal for patients with iliofemoral DVT
Surgical Venous Thrombectomy
Surgical venous thrombectomy represents the first technique developed for eliminating venous thrombus. Although it is an invasive procedure, surgical venous thrombectomy is effective in removing thrombus and decreasing the risk of PTS. Plate et al. randomized patients to surgical venous thrombectomy plus anticoagulation versus anticoagulation alone [6, 84, 85]. They found that patients randomized to thrombectomy had higher venous patency rates, improved venous hemodynamics, and a reduced risk for developing PTS. More recent studies confirm that venous thrombectomy significantly reduces early morbidity in patients with iliofemoral DVT who present with severe symptoms including phlegmasia cerulea dolens. Comerota and Gale described the basic principles and operative techniques of contemporary surgical venous thrombectomy [86]. Technical improvements include the use of venous thrombectomy catheters, infrainguinal thrombectomy, fluoroscopic-guided iliocaval thrombectomy with completion intraoperative phlebography, correction of underlying venous outflow stenoses, construction of an arteriovenous fistula, and immediate and prolonged therapeutic anticoagulation in the postoperative period with catheter-directed heparin infusion into the thrombectomized venous system. The long-term benefits of venous thrombectomy stem from its ability to restore proximal venous patency and preserve the competence of venous valves. Both of these benefits depend upon the initial technical success of the procedure and the avoidance of recurrent thrombosis.
Catheter-Directed Thrombolysis
In recent years, catheter-directed thrombolyis (CDT) has emerged as a less-invasive alternative to surgical venous thrombectomy. CDT targets patients with extensive iliofemoral DVT and shares the same treatment objectives as surgical thrombectomy. Both strategies attempt to eliminate thrombus in order to relieve acute pain and suffering and reduce long-term postthrombotic morbidity. Technical success after CDT for acute DVT ranges from 80 to 90 % [87]. In a cohort-controlled observational study of patients with iliofemoral DVT, Comerota et al. showed that CDT significantly improved the quality of life compared to treatment with anticoagulation alone [83].
Pharmacomechanical Thrombolysis
One drawback of CDT using the infusion technique involves the long treatment times required to adequately clear the clot. Sillesen et al. [88] reported the results of standard CDT using drip technique in a young patient cohort (mean age of 31 years) with iliofemoral DVT who had a short duration of symptoms (less than 6 days). At hospital discharge and 1-year follow-up, 93 % of their patients had patent veins and normal venous valve function. These results required a mean treatment time of 71 h, which may exceed the capacity of many health systems and the patience of many physicians. Integrating percutaneous mechanical thrombectomy into the treatment regimen can accelerate thrombolysis while reducing the dose of lytic agent and shortening overall treatment time. Pharmacomechanical thrombolysis, the combination of lytic infusion and mechanical thrombectomy, has gained popularity as an alternative to the standard drip method of CDT.
A number of studies compared percutaneous mechanical thrombectomy alone with treatment regimens that combine mechanical clot removal with enzymatic thrombolysis [89, 90]. While percutaneous mechanical thrombectomy alone achieved limited success, significant benefit was observed when plasminogen activators were incorporated into the mechanical clot disruption.
Parikh et al. evaluated the technique of ultrasound-accelerated thrombolysis in 53 patients with acute DVT (60 % lower extremity) [91]. After a median infusion time of 22 h, 70 % of the patients had greater than 90 % lysis, 91 % had complete or partial lysis, and only 4 % of patients developed a major complication. When compared to historical controls, there was a significant advantage of ultrasound-accelerated lysis in reducing the lytic dose and shortening treatment times.
Baker et al. reported different results in a single-center retrospective analysis of CDT (N = 19) versus ultrasound-accelerated thrombolysis (N = 64) in patients with iliofemoral DVT [92]. The baseline parameters, extent of DVT, and time since onset of symptoms did not differ between groups. Both treatment groups had a similar and substantial resolution of thrombus load (CDT = 89 %, ultrasound = 82 %; P = 0.560). There were no significant differences in the lytic drug infusion rate, dose of plasminogen activator, infusion time, or use of adjunctive procedures between groups. Major and minor bleeding complications were observed in 8.4 and 4.8 % of patients and were not statistically different between groups. Resolving the conflicting data regarding ultrasound-accelerated thrombolysis will require an appropriately designed randomized trial.
The technique of isolated segmental pharmacomechanical thrombolysis (ISPMT) employs a double-balloon catheter known as the Trellis catheter (Covidien, Mansfield, MA). After inflating the two balloons, thrombolytic agent is directly infused into the isolated venous segment. A dispersion wire is then advanced into the catheter, causing the catheter to assume a spiral configuration between the two balloons. The catheter is activated to spin at 3,500 rpm for 20 min and is then aspirated to remove the macerated and dissolved thrombus. After deflating the balloons repeat venography assesses venous patency. If the results are satisfactory, the catheter can be repositioned to treat additional vein segments. If thrombolysis is inadequate, treatment can be repeated. Although some physicians use up to 10 mg of recombinant tissue plasminogen activator (rtPA) per segment, the authors feel that this represents an unnecessarily excessive dose. Using 2–3 mg of rtPA in the infusate should yield the same efficacy without exposing the patient to the potential risk of a systemic lytic effect if multiple runs were required. This is supported by personal observation and the evolution of catheter-directed thrombolysis during the past 10–12 years. Overall, the interventional community has started to recognize the success of lower doses of rtPA delivered in higher volumes of infusion (50–100 cc/h). Future studies may show that even 2–3 mg of rtPA is overdosing if smaller doses prove to be equally effective.
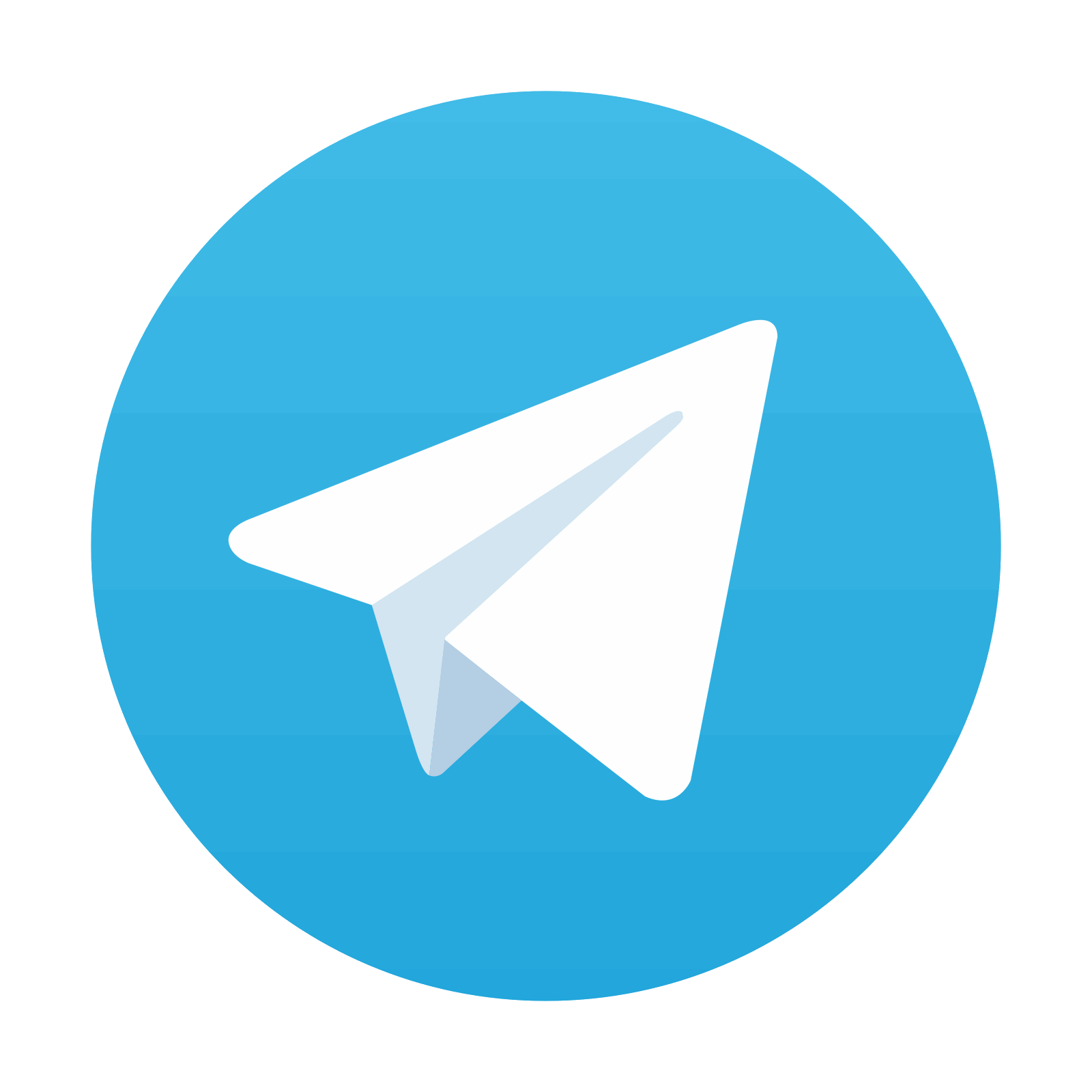
Stay updated, free articles. Join our Telegram channel

Full access? Get Clinical Tree
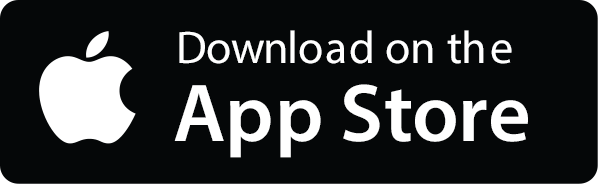
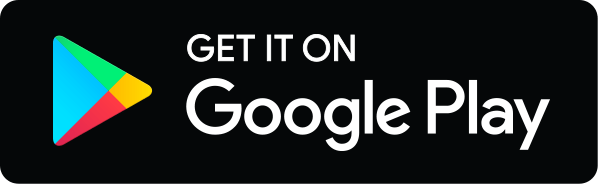