Imaging modalities for peripheral arterial disease (PAD) cover the gamut from to noninvasive approaches to invasive digital subtraction angiography (DSA), which historically has been the reference standard. The advent of percutaneous revascularization has seen shift in imaging strategies to predominantly noninvasive approaches that can provide adequate visualization of arterial stenoses to allow accurate treatment planning. Because of the arterial puncture, DSA requires postprocedural monitoring and has a 2% to 3% major complication rate.1,2 The advent of computed tomography (CT) and magnetic resonance angiography (MRA) has relegated DSA to an adjunctive role that may occasionally be required in some patients at the time of percutaneous revascularization. In this chapter, we will discuss the application of CT technology for the diagnosis of vascular disease in the specific vascular beds (extremities, extracranial vasculature, and thoracic and abdominal aorta).
Current generation CT scanners employ the so-called “rotate/rotate” geometry, in which both x-ray source and detector are mounted onto a rotating gantry and rotate around the patient (Figure 19-1). Key requirement for the mechanical design of the gantry is the stability of both the x-ray source and detector position during rotation, in particular with regard to the rapidly increasing rotational speeds of modern CT systems (0.33 s for 64 slice systems). In a multidetector CT system (MDCT), the detector comprises several rows of 700 and more detector elements, which cover a scan field of view of usually 50 cm. The x-ray attenuation of the object is measured by the individual detector elements. Each detector element consists of a radiation-sensitive solid-state material (such as cadmium tungstate, gadolinium oxide, or gadolinium oxisulfide with suitable dopings), which converts the absorbed x-rays into visible light. The light is then detected by a silicone photodiode. The resulting electrical current is amplified and converted into a digital signal. The detectors laid out in the z-axis are an important determinant of volume coverage (along with other factors, see factors below). The “volume concept” pursued by GE, Philips, and Toshiba aims at a further increase in volume coverage by increasing the number of detectors (256 and beyond) without changing the physical parameters of the scanner compared to the 16-slice version. The “resolution concept” pursued by Siemens uses a set number of physical detector rows in combination with a oscillating x-ray source, enabled by a periodic motion of the focal spot in the z-direction, to simultaneously acquire twice the number of overlapping slices as the number of detectors using an overlapping approach (Figure 19-2).
FIGURE 19-1.
Crossectional view of the inside of a modern CT gantry.
Reproduced with permission from Debabrata M, Rajagopalan S, eds. CT and MR Angiogrpahy of the Peripheral Circulation: Practical Approach With Clinical Protocols. 1st ed. Boca Raton, FL: Informa Healthcare, Taylor and Francis; 2007.

FIGURE 19-2.
Principle of spiral/helical CT-scanning: the patient table is continuously translated while multiple rotations of scan data are acquired. The path of x-ray tube and detector relative to the patient is a helix.
Reproduced with permission from Debabrata M, Rajagopalan S, eds. CT and MR Angiogrpahy of the Peripheral Circulation: Practical Approach With Clinical Protocols. 1st ed. Boca Raton, FL: Informa Healthcare, Taylor and Francis; 2007.

State-of-the-art x-ray tube/generator combinations provide a peak power of 60 to 100 kW, usually at various user-selectable voltages, e.g., 80, 100, 120, and 140 kV. The two basic modes of MDCT data acquisition are axial and spiral (also called helical) scanning. Spiral/helical scanning is characterized by continuous gantry rotation and continuous data acquisition while the patient table is moving at constant speed into the scanner. Axial scanning has no role in computed tomographic angiography (CTA) applications.
The selection of the specific acquisition parameters depends on the employed scanner model. In general, acquisition parameters for peripheral CTA are not significantly different from the ones used for abdominal MRA. A tube voltage of 120 kV with amperage of 200 to 250 mA s (adjustable based on the patient’s body size) are generally used. Tube current may need to be increased in the obese patient to counter attenuation by fat and thoracic wall structures (causes for low signal to noise ratio).
In multidetector CT spiral scans, the volume coverage speed (v, cm/s) can be estimated by the following formula:
The length of table feed per second in relationship to the width of the collimation define the pitch p. The pitch value indicates the degree of gaps in the acquisition (p > 1) or the extent of data overlap (p < 1).
By considering the formula reported above, it is evident that volume coverage can be increased by singularly or concomitantly changing the various scanner parameters (i.e., wider scoll, shorter trot, higher p, and larger M). With current multislice CT, volume coverage speed may be too fast for an adequate visualization of contrast in the entire lower extremity vascular tree, especially in patients with significant stenotic lesions. For instance, a 64-slice scanner (M = 64), with 0.625 mm collimation, pitch of 1.375, and gantry rotation time of 0.5 second can be as fast as 110 mm/s. Such a coverage speed poses a considerable danger of “outrunning” the bolus when the table moves faster than the contrast medium bolus. It has been shown that consistently good results in peripheral CTA can be achieved if maximum acquisition speed does not exceed 30 mm/s. With a 4-slice scanner, a beam collimation of 4 × 2.5 mm, a pitch of 1.5 and 0.5-second gantry rotation time, would provide a volume coverage speed of 30 mm/s. With a 16-slice scanner, a volume coverage speed of 28.8 mm/s is obtained with 16 × 0.75 mm beam collimation at 0.5-second gantry rotation time and pitch of 1.2. The 64-slice scanners (64 × 0.6 mm detector configuration with Siemens scanners) have to be even more slowed down by choosing longer rotation times or reducing the pitch to values below 1. A suitable protocol for a 64-slice CT scanner includes 0.5-second gantry rotation time and pitch 0.8 for a volume coverage speed of 30.7 mm/s. A system with 64 × 0.625 mm collimation (GE scanners) has to be operated at a slower gantry rotation time of 0.7 second and pitch 0.5625 to achieve a volume coverage speed of 32 mm/s.
The prescribed scan volume depends on the vascular bed being examined. The direction of scanning can be switched from either a craniocaudal direction to a caudocranial direction depending on the application.
For most peripheral applications, detectors with submillimeter detector collimation (0.6–0.75 mm) are preferable in view of the size of the anatomic structures that may need to be characterized (e.g., small aneurysms, dissections, etc.). Table 19-1 lists the advantages and disadvantages of CT while Table 19-2 contrasts CT with MRA.
Imaging Characteristics (Advantages and Disadvantages) of CT
Isotropic voxels (0.4 × 0.4 × 0.4) |
MSCTA robust for PAD (PPV > 90%, NPV 99%, SENS 97%, SPEC 96% for popliteal-crural vessels) |
High agreement for occlusion vs. nonocclusion assessment of distal vessels better with MSCTA vs. x-ray DSA |
Calcified segments significant predictor of need for additional imaging and interobserver variability |
Less expensive than MRA |
Relative Advantages and Disadvantages of CTA and MR Angiography (MRA) for the Evaluation of Peripheral Arteries
CTA | MRA |
---|---|
Advantages:
| Advantages:
|
Disadvantages:
| Disadvantages:
|
Radiocontrast injection is perhaps the single most important factor in achieving consistently good results. The goal is to achieve homogeneous “pure arterial” (without venous or tissue contamination) enhancement synchronized with image acquisition.3 Radiocontrast is injected through an appropriately sized (20 G or lower) antecubital vein with the attached IV tubing free of kinks. Saline flushing (30–50 cc) is routinely used after contrast media injection to transport the contrast media from the venous circulation into the arterial system. This saline chaser increases the amount of delivered iodine, contributing to arterial opacification, and reducing the overall amount of contrast media needed.4 The usage of automated dual injectors that provide high flow rates followed by a saline bolus to compact the contrast bolus and to flush it from the peripheral to the central circulation. The imaging process must be timed with peak opacification of the arteries being studied using either by a test-bolus or an automatic bolus triggering in the abdominal aorta.5 Factors that affect transit time such as low cardiac output states and severe PAD can alter filling of the arterial tree. Low osmolality, nonionic contrast agents are generally preferable (350–400 mg of iodine/mL). A volume of 60 to 100 mL (depending on the flow rate and the scan duration) at a flow rate of 4 to 5 mL/s is typically used. The length of the bolus at the very least should correspond to the acquisition time. In the event a “care of tracking bolus” approach is being used, an additional delay before the machine switches to the high-resolution mode (4–5 seconds) may be required.
A test bolus of 10 mL of contrast is administered at the same flow rate as being planned for the CTA. A premonitoring slice with 5mm collimation may be obtained in a large vessel in proximity to the vascular bed that needs to be imaged. Repeated scans are obtained with lowest possible kV after a scan delay of 6 to 10 seconds (total duration being 30 seconds). The time to peak enhancement is evaluated from the scans. The scan delay for the CTA is calculated using the time to peak enhancement to which another 2 to 5 seconds are added; the longer the time to peak enhancement, the longer the time delay for contrast infusion.
A region of interest is placed in a large vessel in proximity to the vascular bed of interest, with a predefined threshold of 50 to 70 Hounsfield units (HU) to initiate the CTA acquisition. The contrast medium infusion and the scanner are activated at the same time with monitoring scans starting after a scan delay of 6 to 10 seconds in order to allow contrast to travel from the peripheral circulation to the central circulatory system. The CTA acquisition mode is automatically activated from the bolus-tracking algorithm after a short pause (5–6 seconds depending on the CT scanner). Breathing instructions are given to the patient during this pause (4–5 seconds) if indicated (as may be case for thoracic or abdominal imaging).
All radiocontrast medias are intrinsically nephrotoxic with low or isoosmolar agents being least offensive. Risk factors for contrast nephropathy including prior renal dysfunction, concurrent use of nephrotoxic drugs, chronic heart failure, diabetes mellitus, low body mass, and advanced age should be identified a priori including estimation of the glomerular filtration rate (GFR) using the modified diet and renal disease (MDRD) equation. Patients at risk (GFR < 40 mL/min) may benefit from an alternate imaging modality or radiocontrast media prophylaxis.6
There are a variety of treatment strategies to prevent contrast-mediated nephrotoxicity. Most treatment regimens require the use of IV hydration, which constitutes the single most efficacious intervention.7 At least 1 L of 0.9% NaCl solution starting several hours precontrast and continued postdye load to facilitate urine output is recommended with repeat chemistry analysis being performed in high-risk patients. Hydration with sodium bicarbonate versus sodium chloride solution was proven superior in preventing kidney dysfunction in a previously published study.8 Another prophylactic strategy is administration of the thiol-containing antioxidant, N-acetylcysteine.9 In contrast, recent statistical reviews have demonstrated mixed results regarding the beneficial effect of acetylcysteine in prevention of contrast-induced kidney injury with results yielding no statistical advantage.10,11 Other adverse effects to contrast media include systemic adverse reactions such as nausea and vomiting, a metallic taste in the mouth, and generalized warmth or flushing. These reactions are usually non–life-threatening, self-limited problems, which require no intervention except adequate communication with patients prior to the procedure.12 On rare occasions, iodinated contrast media can cause anaphylaxis (itching, urticaria, angioedema, and bronchospasm, or arterial hypotension and shock) within minutes after administration.13 Although the incidence of severe anaphylaxis reaction is low, an epidemiological study of severe anaphylactic/anaphylactoid reactions among hospital patients found intermediate risk for anaphylaxis with contrast media. The incidence of anaphylaxis/anaphylactoid reactions due to contrast media in this report was 71 with high-osmolar contrast media and 35 with low-osmolar contrast media per 100 000 procedures.14 To prevent such reactions, patients should have a meticulous allergy history taken, which elicits details from previous contrast exposure. Isoosmolar agents should be given preference if available. Some institutions will give prophylactic agents to retard against an allergic response. Recommendations for high-risk patients who must receive radiographic contrast media include use of a lower osmolar agent, pretreatment with a corticosteroid, and an H1-antagonist, discontinuation of beta-blockers if the patient is taking any, and bedside availability of appropriate medications and equipment to treat anaphylaxis.15 After the test, the patient is reminded to drink sufficient fluids. Observation for a period of 20 to 30 minutes postprocedure is required for patients with known allergies or symptoms during the scan.
PAD is a manifestation of atherosclerosis in the arteries of the lower extremities. PAD affects 8 million Americans and is associated with significant morbidity and mortality.16,17 The presence of PAD implies vascular disease at other sites including the cardiovascular and cerebral vascular beds. High-risk groups such as those with traditional cardiac risk factors, which include smoking, hyperlipidemia, and diabetes as well as end-stage renal disease (ESRD), should be evaluated for PAD to prevent associated vascular events. Early diagnosis and treatment of PAD is necessary principally to prevent adverse cardiovascular outcomes including death, myocardial infarction, amputations, and stroke and secondarily to reduce adverse limb outcomes including functional consequences of disabling claudication. The diagnosis of PAD is based in most cases on a careful history and physical examination, supplemented by determination of ankle brachial index. In some cases additional testing is required for the diagnosis and may include a variety of noninvasive modalities. Noninvasive imaging with CTA and magnetic resonance angiography are useful for the diagnosis but in most cases are invaluable in the treatment planning of patients with PAD.18
In general, approaches for reconstruction of peripheral nongated CTA images follow a similar protocol irrespective of the circulatory bed and will be expounded here. When reconstructing axial images from a CTA, a smooth convolution kernel (filter) is preferable (B25f or B30f). The kernel is an important parameter as the image resolution is mainly determined by the convolution kernel chosen for image reconstruction and not by the in-plane voxel size. Typical convolution kernels used for peripheral CTA studies provide a resolution of 8–10 line-pairs/cm, corresponding to 0.5 to 0.6 mm object size in the scan plane that can be resolved. All relevant information will be displayed as soon as the image voxel size is smaller than the minimum object size that can be resolved with the selected convolution kernel. For peripheral CTAs, this is usually achieved when the field-of-view (FOV) for image reconstruction is not larger than 250 to 300 mm. Further reducing the FOV will reduce the in-plane voxel size, but will not increase image resolution. Only in special cases, such as high-resolution thorax imaging with a large FOV of 350 to 400 mm, will the in-plane voxel size be the limiting factor for in-plane resolution. Reconstruction increment defines the overlap of the axial images during reconstruction. Overlap of 20% to 50% (reconstruction increment of 0.5–0.8) has been recommended for peripheral angiographic applications.
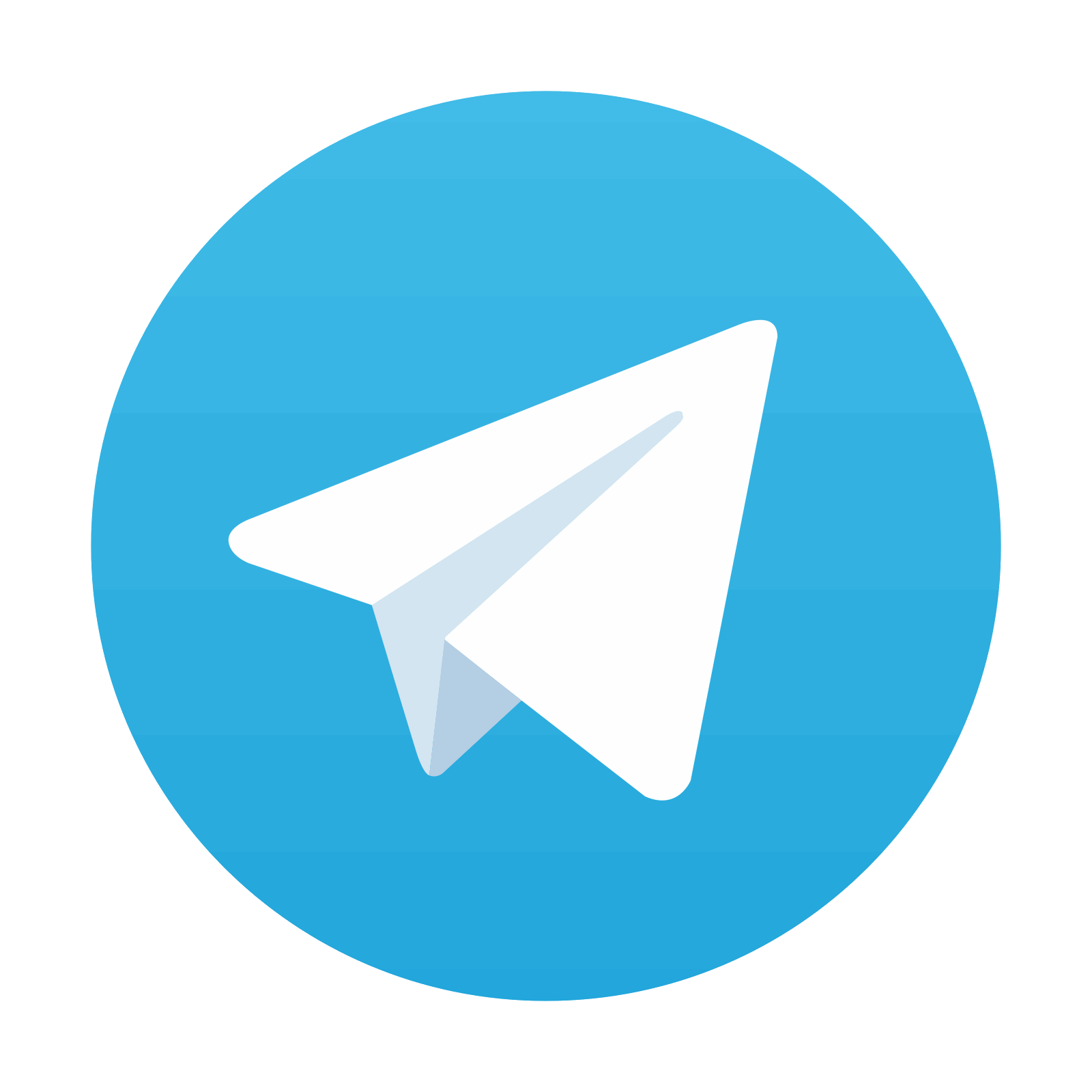
Stay updated, free articles. Join our Telegram channel

Full access? Get Clinical Tree
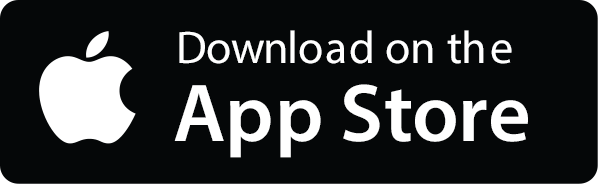
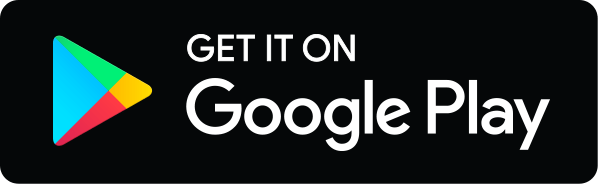