Fontaine
Rutherford
Stage
Clinical
Grade
Category
Clinical
I
Asymptomatic
0
0
Asymptomatic
IIa
Mild claudication
I
1
Mild claudication
IIb
Moderate to severe claudication
I
2
Moderate claudication
I
3
Severe claudication
III
Ischemic rest pain
II
4
Ischemic rest pain
Critical limb ischemia
IV
Ulceration or gangrene
III
5
Minor tissue loss
III
6
Major tissue loss
While the presence of PAD implies an imbalance of arterial blood supply versus demand, the arterial disease observed in CLI is severe enough that perfusion fails to meet the resting metabolic requirements of the tissues. The chronic lack of blood flow initiates a cascade of pathophysiologic events that ultimately leads to rest pain or trophic changes of the lower extremity or both. Critical limb ischemia usually results from severe atherosclerotic stenoses or occlusions at two or more levels of the lower extremity arterial tree. This multi-level disease pattern severely diminishes flow through collateral beds leading to limb-threatening ischemia. Other less common etiologies of CLI include atheroembolic or thromboembolic diseases, vasculitis, in situ thrombosis related to hypercoagulable states, popliteal artery entrapment, trauma, cystic adventitial disease, and thromboangiitis obliterans [2]. Regardless of the underlying cause, the pathogenesis of CLI involves a complex array of changes to the macrovascular and microvascular systems and surrounding tissues of the lower extremity [3].
Clinical Manifestations
Claudication
Intermittent claudication occurs due to relative muscle ischemia from a transient imbalance between arterial blood supply and demand. Patients with claudication have reproducible discomfort of a defined group of muscles that is induced by exercise and relieved with rest. The lower extremity discomfort predictably occurs one level distal to the anatomic location of arterial occlusive lesions; however, symptoms can vary widely in distribution and severity. Buttock and hip discomfort usually correlates to aortoiliac disease, whereas thigh discomfort is associated with aortoiliac or common femoral artery disease, upper calf discomfort is associated with superficial femoral artery disease, lower calf pain is associated with popliteal artery disease, and discomfort at the level of the foot or instep is associated with tibioperoneal arterial disease. Claudication, even in its most severe form, does not imply limb-threatening ischemia or impending CLI. Symptoms of claudication usually remain stable over time, and very few patients with claudication progress to CLI. The majority of patients with CLI do not have a history of slowly worsening claudication. Instead, they have debilitating ischemic ulcers and gangrene at the time of their initial presentation.
Rest Pain
Ischemic rest pain is characterized by severe burning pain localized to the forefoot and toes of a chronically ischemic lower extremity. Such diffuse pedal ischemia is associated with a systolic arterial blood pressure less than 40 mmHg at the ankle and less than 30 mmHg at the toes [4]. The discomfort associated with rest pain is often refractory to analgesics and can be prompted or aggravated by elevation of the lower extremity. Patients frequently experience ischemic rest pain at night owing to the relative elevation of the lower extremity during the recumbent or reclining position assumed while trying to sleep. Limb dependency, on the other hand, typically results in symptomatic improvement or sometimes complete resolution of discomfort in the lower extremity. This tendency to keep the ischemic lower extremity in a dependent position explains why some patients with CLI present with symptoms of lower extremity edema.
Ischemic Ulcer
An ischemic ulcer forms after an initial break in the integrity of the skin as a result of soft tissue trauma. Such trauma is typically mild and occurs at sites of increased focal pressure in the lower extremity such as the lateral malleolus, metatarsal heads, or distal extent of the toes. Due to impaired oxygenation and inadequate blood flow to the site of injury, breaks in the skin fail to heal and persist as chronic, painful erosions [5]. Ischemic ulcers are usually dry and punctate and may be associated with other signs of chronic lower extremity ischemia, including rest pain, hair loss, skin atrophy, nail hypertrophy, and pallor. The aching or burning pain that patients with ischemic ulcers experience results from both chronic severe ischemic neuropathy and the presence of sensory nerves at the site of the ulcer.
Gangrene
Tissue necrosis, or gangrene, occurs when the vascular supply of the lower extremity fails to maintain cellular viability. The lower extremity may appear cyanotic with anesthetic tissue that is associated with or progresses to frank necrosis. The extent of gangrene can range from a single digit to the entire lower extremity depending on the severity of underlying PAD and local resting metabolic requirements. Patients may initially describe severe pain due to a variety of factors, including ischemic neuropathy, osteomyelitis, coexisting ascending infection, or associated ischemic injury to the skin and subcutaneous sensory nerves. Pain typically abates with the progression of gangrene due to ischemic necrosis of the sensory nerves and surrounding tissues.
Gangrene is described as either dry or wet based on clinical examination. Dry gangrene appears gradually and progresses slowly. Tissue becomes cold and has a characteristic hard, dry texture that ultimately sloughs off. Dry gangrene can result from embolization to the toe or forefoot and usually produces a clear demarcation between viable and necrotic tissue. A clearly demarcated digit or forefoot may autoamputate without proximal progression of gangrene. Due to the rather slow and emotionally disturbing nature of demarcation, an elective amputation of the involved portion of the lower extremity provides a more acceptable alternative. Most patients have inadequate arterial perfusion to heal the distal amputation site and therefore require a revascularization procedure for limb salvage.
Wet gangrene is a life-threatening complication of an untreated infected wound, which requires emergency surgery. Infection of the extremity by saprogenic microorganisms such as Clostridium perfringens produces soft tissue edema and cessation of local arterial and venous blood flow. The stagnant blood pools in the lower extremity and promotes further rapid bacterial proliferation. Bacterial toxins are absorbed by the surrounding tissue of the lower extremity resulting in fulminant sepsis and death if not treated promptly with intravenous antibiotics and debridement or amputation. Wet gangrene represents the progression of coagulative necrosis to liquefactive necrosis, with the affected limb featuring a moist, edematous, soft, and often blistering appearance.
Patient Evaluation
Diagnosis
Clinical History
The evaluation of a patient with PAD begins with a detailed history and careful physical examination. Although patients with PAD may report limitations in exercise performance and walking ability, the 2005 American College of Cardiology/American Heart Association (ACC/AHA) guidelines on PAD suggest that only 10–35 % of patients present with classic claudication symptoms (Fig. 7.1) [2]. Less than 3 % of patients present with symptoms of CLI. Instead, the majority of patients with PAD are either asymptomatic or complain of atypical leg pain at initial clinical presentation. An estimated 5–10 % of patients with asymptomatic PAD or claudication will progress to CLI within 5 years.
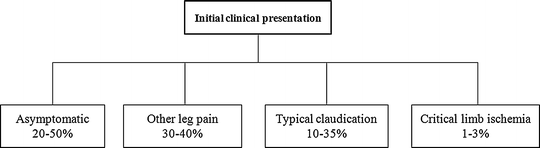
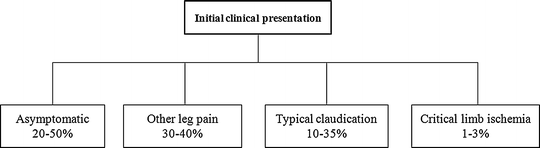
Fig. 7.1
Initial clinical presentation of patients with peripheral arterial disease (PAD)
Identification of risk factors for PAD can aid in distinguishing symptoms of PAD from other less common causes of lower extremity ischemia such as radiation fibrosis, fibromuscular dysplasia, popliteal entrapment, vasculitis, and adventitial cystic disease. Non-arterial and nonvascular pathologies should also be considered in the differential diagnosis of lower extremity discomfort, including deep venous thrombosis, peripheral neuropathy, spinal stenosis, and musculoskeletal disorders. Because atherosclerosis is a systemic disease, the risk factors for PAD mirror those of coronary artery disease. In patients with a history of hypertension, tobacco use, diabetes mellitus, chronic renal failure, and dyslipidemia, PAD is more likely to be the underlying cause of their lower extremity complaints [6–9]. The 2005 ACC/AHA guidelines also identified the following groups to be at increased risk for lower extremity PAD: age ≥70 years, age 50–69 years with a history of smoking or diabetes, and age 40–49 with diabetes and at least one additional risk factor for atherosclerosis, leg symptoms suggestive of claudication with exertion or ischemic pain at rest, abnormal lower extremity pulse examination, and those patients with known atherosclerosis at other anatomic sites (e.g., coronary, carotid, or renal artery disease) [2].
Physical Examination
Clinical findings consistent with PAD include cool temperature of the leg, absent or diminished pulses in the legs or feet, thin or shiny appearance of the skin, hair loss, nonhealing wounds or gangrene, pallor with elevation of the extremity, dependent rubor (foot redness with dependent position), and hypertrophic changes of the toenails. Patients may also demonstrate restricted mobility as a result of numbness, weakness, or heaviness in the muscles of the leg. Examination in patients with suspected lower extremity arterial disease should include a thorough search for the presence and strength of all lower extremity pulses, as well as evaluation for cardiac murmurs, bruits, and aneurysms. Pulses should be assessed on both legs at all levels and any abnormalities correlated with lower extremity symptoms to determine lateralization of occlusive disease. A diminished or absent femoral pulse suggests inflow disease due to aortoiliac occlusive lesions. A normal femoral but absent distal pulse suggests preserved inflow but impaired infrainguinal outflow to the leg.
Despite the utility of the pulse examination, physical exam of the femoral artery pulse has been shown to be little better than relying on subjective symptoms of claudication to diagnose large-vessel PAD. Moreover, the absence of pedal pulses tends to overdiagnose PAD as evidenced by the fact that only 18 % of patients with abnormal posterior tibial pulses have additional objective evidence of PAD [10]. The diagnosis of PAD in suspected patients must therefore be confirmed using noninvasive screening tests and, if needed, other hemodynamic or imaging studies.
Routine Noninvasive Vascular Screening Studies
The resting ankle-brachial systolic pressure index (ABI) should be performed in all patients with a clinical history and/or physical exam suggestive of PAD. This chapter describes the technique for measuring an ABI.
The normal reference range for ABI is 0.90–1.2. A decreased ABI in symptomatic patients confirms the presence of hemodynamically significant peripheral arterial occlusive disease between the heart and ankle, with a lower ABI correlating with more severe occlusive disease. An ABI of less than 0.90 is 95 % sensitive in identifying angiographically confirmed PAD [1, 11]. Patients with claudication generally have an ABI in the range of 0.40–0.90, whereas those with rest pain or tissue loss have an ABI in the range of 0.20–0.50 and zero to 0.40, respectively. It is important to note, however, that over 50 % of patients with an abnormal ABI will fail to demonstrate typical symptoms of claudication or CLI due to the presence of other major comorbidities [1]. In addition to its ability to detect PAD, the ABI may also function as a marker for systemic atherosclerotic disease. Several studies have noted a strong correlation between ABI and mortality [12–14], with one report even noting a near linear relationship between ABI and fatal and nonfatal cardiovascular events [13].
Significant arterial calcification causes blood vessel incompressibility which will falsely elevate the ABI. In such cases, a toe-brachial index may be used to diagnose PAD as digital vessels are often spared from calcification. The toe-brachial index is calculated by dividing the toe systolic pressure by the highest brachial systolic pressure and can be measured by a noninvasive vascular lab using small blood pressure cuffs. A normal toe-brachial index is greater than 0.70. Most cases of CLI are associated with ankle systolic blood pressures less than 50 mmHg or toe systolic blood pressures less than 30 mmHg. Foot lesions usually heal when toe pressure exceeds 30–40 mmHg (or slightly higher in diabetic patients).
To evaluate PAD in more detail, the noninvasive vascular lab can perform segmental limb systolic pressures, plethysmography, Doppler waveform analysis, and exercise testing. More details of these exams can be found in this chapter.
Pre-intervention Imaging
Imaging studies are not required to confirm the diagnosis nor should they be used as first-line screening tests for CLI or PAD. The primary role of imaging studies involves treatment planning for patients who require revascularization for limb salvage. Imaging exams create an arterial road map that helps determine what, if any, endovascular and surgical therapy options are feasible.
Duplex Ultrasound
Duplex ultrasound provides a reliable and inexpensive imaging option to evaluate arterial occlusive disease and other vascular pathologies. Capabilities of duplex ultrasonography include the ability to differentiate stenoses from occlusions, characterize the occlusive nature of specific arterial segments, evaluate for arterial trauma, assess adequacy of surgical intervention, and provide surveillance for bypass grafts. Duplex ultrasound scanning usually proceeds from proximal to distal, recording artery diameter, presence and character of atherosclerosis, and velocity spectral waveform. In stenotic lesions that significantly reduce the vessel diameter, arterial flow becomes turbulent with increased systolic and diastolic velocities. Velocity criteria can classify the severity of the lesion with greater than 80 % accuracy depending on the arterial segment scanned.
Although the exam quality depends on the technical skill of the sonographer, duplex ultrasound has proven to be a sensitive and specific test for arterial occlusive disease. A meta-analysis of 14 studies demonstrated the sensitivity and specificity of duplex scanning for ≥50 % stenosis or occlusion to be 86 and 97 % for aortoiliac disease and 80 and 98 % for femoropopliteal disease [15]. In a recent prospective, blinded study, Eiberg et al. [16] compared duplex ultrasound scans to the angiographic findings in 42 patients with claudication and 127 patients with CLI. The interobserver agreement between duplex ultrasound and angiography was generally good with both techniques independent of the severity of PAD. Duplex ultrasound was noted to perform better in the supragenicular arteries than in the infragenicular arteries but still compared favorably with angiography down to the level of the tibial vessels. Several additional studies reported a similar correlation between these two imaging studies [17–19]. Such findings support duplex ultrasound as a useful noninvasive alternative to conventional contrast angiography, particularly among those patients with renal failure and contrast allergies.
Computed Tomographic Angiography
Multidetector computed tomographic angiography (CTA) allows for the rapid acquisition of high-resolution, contrast-enhanced arterial images. The CTA images can then be reviewed in multiple projections and reconstructions to illustrate artertial anatomy, localize occlusive disease, and detect vessel wall calcification. The abundance of information gained from CTA has made it a widely used exam for treatment planning. A meta-analysis of 12 studies evaluating 9,541 arterial segments in 436 patients showed that multidetector CTA detected greater than 50 % segmental stenosis with a pooled sensitivity and specificity of 92 and 93 %, respectively [20]. The diagnostic performance of CTA was noted to be lower in the infrapopliteal arteries but not significantly different from that observed in the aortoiliac or femoropopliteal arterial systems. More recently, a meta-analysis published in 2009 of 20 studies similarly compared the diagnostic performance of CTA to the reference standard contrast angiography for the evaluation of 19,092 lower extremity arterial segments in 957 symptomatic patients [21]. The overall sensitivity and specificity of CTA in that analysis was 95 and 96 %, respectively.
The drawbacks of CTA include its high cost and the associated exposure to both ionizing radiation and intravenous nephrotoxic contrast agents. CTA requires an intravenous bolus of more than 100 mL of iodinated contrast in the average adult, making it less desirable for patients with chronic renal insufficiency (estimated glomerular filtration rate (eGFR) <60 ml/kg/min). An imaging pitfall specific to CTA involves the use of narrow window settings to optimize image quality in the presence of arterial wall calcifications or previously placed stents. These imaging settings may overestimate the degree of stenosis or suggest a spurious arterial occlusion. In patients with diabetes or ESRD, extensive atherosclerotic calcification within small crural or pedal arteries may make it impossible to detect a patent vessel lumen regardless of the window/level selection. Fortunately, most of these artifacts are easily identified by examining additional views, complementary imaging modalities, or source images [22].
Magnetic Resonance Angiography
Three-dimensional gadolinium-enhanced magnetic resonance angiography (MRA) offers an increasingly common noninvasive option to assess the peripheral vasculature without the need for sedation, catheterization, ionizing radiation, or iodinated contrast agents. The sensitivity and specificity for the detection of greater than 50 % arterial stenoses or occlusions using this modality exceed 90 % [23–25]. Owen and colleagues [26] conducted a prospective comparative study of 30 patients who underwent both MRA and contrast angiography. Evaluation of 390 arterial segments in these patients demonstrated a higher sensitivity in distal segments (92–96 %) compared with more proximal segments (69–79 %). Specificity was similar in both distal (90–91 %) and proximal segments (86–96 %).
Magnetic resonance angiography generates a three-dimensional data set that can be reformatted to reproduce digital subtraction angiography-like vascular images that feature details pertinent to prognosis and treatment planning, including those related to the thrombus characteristics, plaque composition, presence of dissection, or extent of arterial wall inflammation. An MRA’s ability to image arteries and the surrounding musculoskeletal structures also makes it ideal for evaluating patients with popliteal artery entrapment syndrome.
There is increasing evidence to suggest that some patients with renal failure who are exposed to gadolinium-based magnetic resonance imaging contrast agents may develop a rare but serious fibrosing disorder referred to as nephrogenic systemic fibrosis (NSF). This disorder is characterized by hardening of the skin and development of fibrotic nodules and plaques. In its most severe form, NSF may result in systemic fibrosis affecting solid organs such as the lungs, heart, and liver [27, 28]. Current clinical guidelines advise against the use of gadolinium in patients with an eGFR less than 30 ml/kg/min. An MRA is also not appropriate for claustrophobic patients or patients with pacemakers or metallic implants.
Contrast Angiography
Digital subtraction angiography (DSA) remains the gold standard imaging modality in peripheral vascular surgery. Angiography should usually be reserved for patients who are expected to undergo attempted revascularization. The exam begins with percutaneous needle access to the common femoral artery in the contralateral limb to allow complete imaging of the inflow, diseased segment, and outflow vessels. DSA involves the generation of fluoroscopic images by subtracting a pre-contrast image from images taken after the administration of an intra-arterial contrast medium, thereby producing a net image showing only contrast-filled blood vessels. The combination of excellent spatial and temporal resolution and a large field of view allows for rapid imaging of the entire peripheral arterial tree. A complete evaluation of the aorta, iliac, femoral, popliteal, and tibioperoneal vessels may be performed simultaneously or, more commonly, sequentially with overlapping runs. In addition, the presence and extent of collateral blood flow can be readily evaluated with this form of imaging. In some cases, measuring the pressure gradients across an arterial lesion (usually in the aortoiliac segment) can increase the arteriogram’s sensitivity and gauge the response to intervention. Hemodynamically significant lesions have a resting systolic pressure gradient which exceeds 20 mmHg.
The clinical utility of angiography must be balanced against the fact that it represents an invasive form of imaging. Angiography requires arterial access, ionizing radiation, and nephrotoxic contrast exposure. Potential complications of angiography include distal atheroembolization, arterial dissection, and access site complications (e.g., pseudoaneurysm, hematoma, thrombosis, arteriovenous fistula). DSA imaging requires a stationary target, and any patient movement will produce significant artifact. Optimizing technical factors such as frame rate, contrast injection volume, and C-arm projection angle will also produce the highest-quality images.
Cardiac Risk Stratification
Cardiovascular events are the most significant complication in patients undergoing vascular surgery. Lower extremity revascularization is classified as a high-risk surgery according to the ACC/AHA preoperative assessment guidelines and carries a combined incidence of cardiac death and nonfatal myocardial infarction of greater than 5 % [2]. The increased rate of cardiovascular complications stems from the fact that patients in need of revascularization belong to a subgroup of high-risk patients who have a corresponding high incidence of CAD and left ventricular systolic dysfunction compared to matched controls [29–31]. Additional clinical predictors of increased perioperative cardiovascular risk include unstable coronary syndromes, decompensated congestive heart failure, significant arrhythmias, and severe valvular heart disease [32]. In this patient population with compromised baseline cardiac function, physiologic stressors associated with vascular surgery such as blood loss, volume shifts, and hemodynamic fluctuations may have a greater impact which further increases their risk of perioperative myocardial ischemia.
The 2007 ACC/AHA guidelines recommend that the estimation of perioperative risk should integrate major, intermediate, and minor predictors of cardiac risk, functional capacity, surgery-specific risk, and, when indicated, the results of noninvasive tests, including stress testing and echocardiography (Table 7.2) [1, 32]. This systematic approach subjects only a minority of patients ultimately to invasive preoperative evaluation, while some patients can forego noninvasive evaluation completely. According to the ACC/AHA guidelines, patients without active cardiac symptoms scheduled for low-risk vascular surgery and those with good functional capacity require no preoperative testing beyond a 12-lead electrocardiogram [2, 33]. Patients with poor or unknown functional capacity or cardiac symptoms are managed according to the number of clinical risk factors. Patients with one or two risk factors can proceed with surgery unless testing will alter management, whereas noninvasive testing is generally recommended for patients with three or more risk factors. Additional evaluation allows refinement of the initial risk estimate and may include exercise or pharmacologic stress testing, resting echocardiography, and/or preoperative cardiac catheterization and coronary revascularization. The aforementioned guidelines are based on preoperative evaluation of patients undergoing elective surgery. Patients requiring urgent or emergent vascular procedures are unlikely to derive benefit from delaying surgery for additional testing and medical management and should proceed directly to surgery.
Table 7.2
Clinical predictors of cardiac risk
Major | Unstable coronary syndromes |
Unstable or severe angina | |
Recent myocardial infarction | |
Decompensated heart failure | |
Significant arrhythmias | |
Severe valvular disease | |
Intermediate | History of heart disease |
History of compensated or prior heart failure | |
History of cerebrovascular disease | |
Diabetes mellitus | |
Renal insufficiency | |
Minor | Advanced age (>70 years) |
Abnormal electrocardiogram, | |
e.g., left ventricular hypertrophy, ST-T abnormalities | |
Rhythm other than sinus | |
Uncontrolled systemic hypertension |
Treatment
Information pertaining to the natural history of non-revascularized lower extremities in patients with CLI is extrapolated from the placebo arms of pharmacotherapy trials performed on patients with unreconstructable peripheral arterial occlusive disease. Unlike the often benign nature of intermittent claudication, nearly 40 % of patients with CLI will progress to amputation within 6 months in the absence of revascularization [34]. Given this dismal prognosis, revascularization plays a prominent role in the overall treatment plan for functional patients with CLI (Fig. 7.2). The primary treatment goals for CLI include relief of ischemic rest pain, healing of ischemic ulcers, limb salvage, improvement of patient function and quality of life, and increased survival. Concomitant medical therapy provides an important adjunct to revascularization as it plays an essential role in controlling ischemic neuropathy, treating or preventing coexisting infections in critically ischemic lower extremities, halting progression of systemic atherosclerosis, and managing the underlying cardiovascular risk profile of patients.
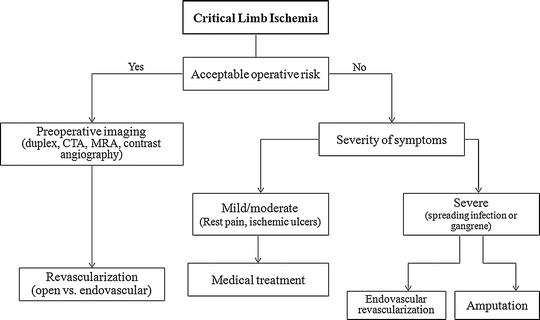
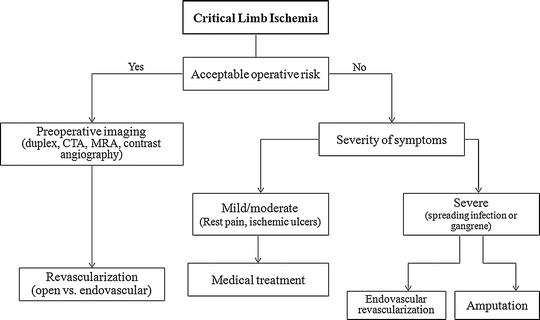
Fig. 7.2
Algorithm for managing patients with critical limb ischemia
It is important to emphasize that medical and surgical treatments for CLI are not mutually exclusive. In fact, it is imperative that all patients with CLI receive medical therapy, whether or not they are candidates to undergo invasive revascularization. To the extent possible, patients should commit to regular exercise, healthy eating habits, and aggressive risk factor modification, including smoking cessation, blood pressure control, reduction in fasting blood glucose levels, and lipid-lowering therapy, as indicated. Antiplatelet and statin therapy also appears to be beneficial. Strict compliance with medical therapy will have a profound impact on the patient’s prognosis and the ultimate success of any revascularization strategy.
Revascularization
Despite a paucity of level I evidence to support proposed treatment algorithms in PAD, surgical bypass has long been considered the gold standard method of revascularization across the PAD spectrum. Continued development of catheter-based technology has expanded the revascularization options such that endovascular intervention is now considered to be a suitable primary therapeutic alternative. The utilization of one approach over another may reflect a generational change in the treatment of lower extremity ischemia, with recently trained vascular surgeons performing a disproportionately higher number of endovascular procedures compared to their older colleagues. The role of endovascular therapy in the treatment of CLI continues to evolve, and its ultimate role will be determined by ongoing technical refinements, growing clinical experience, and the clarification of long-term outcomes and costs.
The Bypass versus Angioplasty in Severe Ischemia of the Leg (BASIL) trial is the only randomized controlled trial to date comparing the efficacy of balloon angioplasty-first versus bypass surgery-first treatment strategies in patients with CLI [35]. In their initial 2005 report, BASIL investigators noted no difference in short-term clinical outcomes but did find that surgery was associated with increased morbidity (57 % vs. 41 %) and greater expense during the first 12 months. Post hoc analysis showed that beyond 2 years from intervention, patients initially randomized to bypass surgery were more likely to be alive and without limb amputation. To further explore this finding, patients were monitored for an additional 2.5 years and analyzed on an intent-to-treat basis, and results were published in 2010 as an extension of the BASIL study [36]. This analysis revealed that patients who survived for 2 years and who were initially randomized to bypass surgery gained a significant 7.3 months (95 % CI, 1.2–13.4 months, P = 0.02) of additional life expectancy and an additional nearly significant 5.9 months (95 % CI, 0.2–12.0 months, P = 0.06) of amputation-free survival over the subsequent follow-up period compared to those initially randomized to the angioplasty-first treatment strategy.
It is important to note several limitations in the BASIL study. First, the study cohort in the BASIL trial was limited to those with CLI due to infrainguinal occlusive disease. Results in such patients cannot be generalized to those with CLI resulting from aortoiliac occlusive disease. Second, the BASIL trial began its 5-year enrollment in 1999, and the results must be interpreted with caution given that over a decade of technological advancement has passed since the study began. Moreover, stenting of the infrainguinal arteries was not considered standard of care in the United Kingdom at the time of the trial, evidenced by the fact that only 9 out of 224 patients randomized to the angioplasty-first strategy had angioplasty combined with stent placement.
The updated ACC/AHA guidelines for the management of PAD stem from the BASIL trial and are consistent with recommendations made by the BASIL trial investigators [33]. Patients with CLI who have a life expectancy or course of disease projected to exceed 2 years are probably better served with a bypass surgery-first strategy, preferably using autogenous vein. Those with CLI who have a life expectancy of less than 2 years, and potentially those who do not have autogenous vein for use as a conduit, are probably better served with an angioplasty-first strategy given that their limited survival precludes them from achieving the potential longer-term benefits of surgery. Moreover, patients deemed to have limited preoperative survival may be more vulnerable to suffer postoperative morbidity and mortality, thereby making the less expensive, minimally invasive nature of endovascular therapy a more appropriate option in this subgroup of patients.
Determining the optimal method of revascularization in CLI is based on a balance between the risks of the specific intervention and the degree and durability of improvement that can be expected from the intervention [1]. The efficacy of any revascularization attempt depends on the arterial inflow, distal outflow, size and length of the diseased arterial segment, degree of systemic disease, and type of procedure performed. Adequate inflow and sufficient outflow are essential in order to maintain long-term patency of any arterial reconstruction. Among patients with concomitant inflow (suprainguinal) and outflow (infrainguinal) disease, the ACC/AHA guidelines recommend that inflow lesions be treated first independent of the chosen treatment strategy [2]. Subsequent revascularization of outflow disease is indicated if the ABI remains less than 0.8 in the presence of ongoing infection, ischemic ulcers, or gangrene.
The Lower Extremity Grading System (LEGS) score offers an outcome-based standardization tool for lower extremity arterial interventions in patients with either claudication or CLI [37]. The score consists of five objective criteria: angiographic pattern of disease, presenting complaints, functional status of the patient, medical comorbidities, and technical factors. The composite LEGS score determines the recommended interventional treatment strategy which may involve endovascular therapy, open surgery, or major limb amputation. Prospective studies demonstrated that patients treated according to the LEGS algorithm had higher rates of limb salvage, patency of arterial reconstruction, and maintenance of ambulation compared to those treated with strategies contrary to LEGS.
Patients with claudication generally have superior patency rates compared to patients with CLI across nearly all forms of arterial reconstruction for chronic lower extremity arterial disease. Since claudicants have less severe arterial occlusive disease and longer life expectancy relative to those with CLI, these patients tend to contribute more to data directed at long-term clinical outcomes. As a result, studies focused primarily on cohorts with low-risk claudicants will report disproportionately better patency and survival data compared to those limited to higher-risk patients with CLI. Most studies report success of revascularization as a function of primary patency, implying uninterrupted patency without the need for re-intervention, or secondary patency, defined as patency of the initially treated vessel following a re-intervention to restore patency after occlusion.
Aortoiliac (Suprainguinal) Revascularization
Severe aortoiliac occlusive disease has been traditionally treated using a variety of open surgical approaches, including aortobifemoral bypass, aortoiliac endarterectomy, iliofemoral bypass, and extra-anatomic bypass. Of these, aortobifemoral bypass has long been considered to be the gold standard treatment for hemodynamically significant aorto-bi-iliac disease in low-risk patients given its safety and efficacy (mortality rate less than 5 %; 5-year patency rates greater than 80 %) [38–41]. The bifurcated bypass graft in this procedure originates from the infrarenal abdominal aorta and routes one limb to each femoral artery via either a transperitoneal or retroperitoneal approach. A preoperative CTA is essential to assess the calcium and thrombus content of the proximal aorta. An aorta with thrombus and plaque extending to the level of renal arteries requires a proximal thromboendarterectomy with a suprarenal aortic clamp in place and the renal arteries controlled with clamps or vessel loops. Once the proximal aortic cuff has been cleared, the clamp can be moved to an infrarenal position and the proximal anastomosis can be performed in an end-to-end or end-to-side fashion. An end-to-end configuration is favored when the aorta is completely occluded to the level of the renal arteries or when severe aortic calcification precludes safe placement of a side-biting clamp. Aortic occlusive disease associated with aneurysmal dilatation also requires an end-to-end reconstruction. An end-to-side configuration has the advantage of maintaining pelvic perfusion in patients with occluded or diseased bilateral external iliac arteries. Preserving distal aortic flow may also be important in patients with a large patent inferior mesenteric artery or accessory renal arteries arising from the infrarenal aorta. The presence of femoral artery occlusive disease requires the addition of a common femoral endarterectomy and profundoplasty. Late complications include graft thrombosis (5–30 %), graft infection (0.5–3.0 %), aortoenteric fistula (<3 %), and anastomotic pseudoaneurysm (1–5 %), which is more common in patients with an end-to-side anastomosis.
More recently, a laparoscopic aortobifemoral bypass has been described as a less invasive alternative to open surgery. Tiek et al. [42] conducted a multicenter randomized controlled trial of 28 patients with TASC C or D aortoiliac occlusive lesions who underwent either open or laparoscopic aortobifemoral bypass. The laparoscopic group had a shorter mean hospital length of stay (5.5 vs. 13.0 days), earlier return to normal daily activities, and fewer postoperative complications compared to the open group. While early studies confirmed the safety of the laparoscopic aortobifemoral bypass, it has not been widely performed and the long-term patency of this approach remains unclear. Future studies may help determine whether laparoscopic aortic surgery is appropriate for a subset of patients with aortoiliac occlusive disease.
Prior to the availability of prosthetic graft material in the 1970s, aortoiliac endarterectomy served as the standard procedure for the treatment of aortoiliac occlusive disease. In a large single-center series of patients who survived over a decade after undergoing either aortoiliac endarterectomy (n = 39) or aortobifemoral bypass (n = 166), 10-year primary patency rates were 89 % for aortoiliac endarterectomy and 78 % for aortobifemoral bypass [43]. Graft infection or aneurysmal formation occurred in 5 % of aortobifemoral bypass cases. Aortoiliac endarterectomy has the advantage of potentially avoiding the need for prosthetic material; however, external iliac arteries often prove difficult to endarterectomize. Investigators therefore concluded that aortoiliac endarterectomy is preferable to aortobifemoral bypass in the following circumstances: (1) patients whose aortoiliac occlusive disease does not involve the external iliac arteries; (2) male patients with aortoiliac occlusive disease who, in addition to claudication, have erectile dysfunction and stenotic internal iliac origins; (3) patients with aortoiliac disease including the external iliac arteries who are not candidates for aortobifemoral bypass because of infection risk or small vessels; (4) patients with localized aortoiliac disease; and (5) patients after removal of an infected aortobifemoral bypass graft (with or without an enteric fistula) that had initially been placed end to side for aortoiliac occlusive disease.
Iliofemoral bypass and femorofemoral crossover bypass are additional open surgical options in patients with aortoiliac occlusive disease and are typically indicated in patients with unilateral iliac disease or in patients deemed not suitable for aortobifemoral bypass. In cases of bilateral iliac disease, a femorofemoral bypass may also be constructed as an adjunct to aortoiliac endarterectomy, iliofemoral bypass, or successful unilateral iliac angioplasty and stenting. Iliofemoral bypass appears to be a safe, effective, and durable bypass. Carsten and colleagues [44] reported a 10-year experience comprising 40 patients who underwent iliofemoral bypass grafting for unilateral aortoiliac disease, 58 % of whom had CLI. There were no perioperative deaths in their series, and limb salvage rates in patients with CLI were 85 and 79 % at 1 and 5 years. In addition, a multicenter randomized trial involving 143 patients with TASC type C or D aortoiliac lesions found that late patency was higher after direct iliofemoral bypass compared to femorofemoral crossover bypass in good-risk patients with unilateral iliac occlusive disease not amenable to angioplasty (93 % vs. 73 %). This study underscores the effectiveness of iliofemoral bypass, and the authors concluded that crossover bypass grafting should be reserved for high-risk patients with unilateral iliac occlusion not amenable to percutaneous intervention.
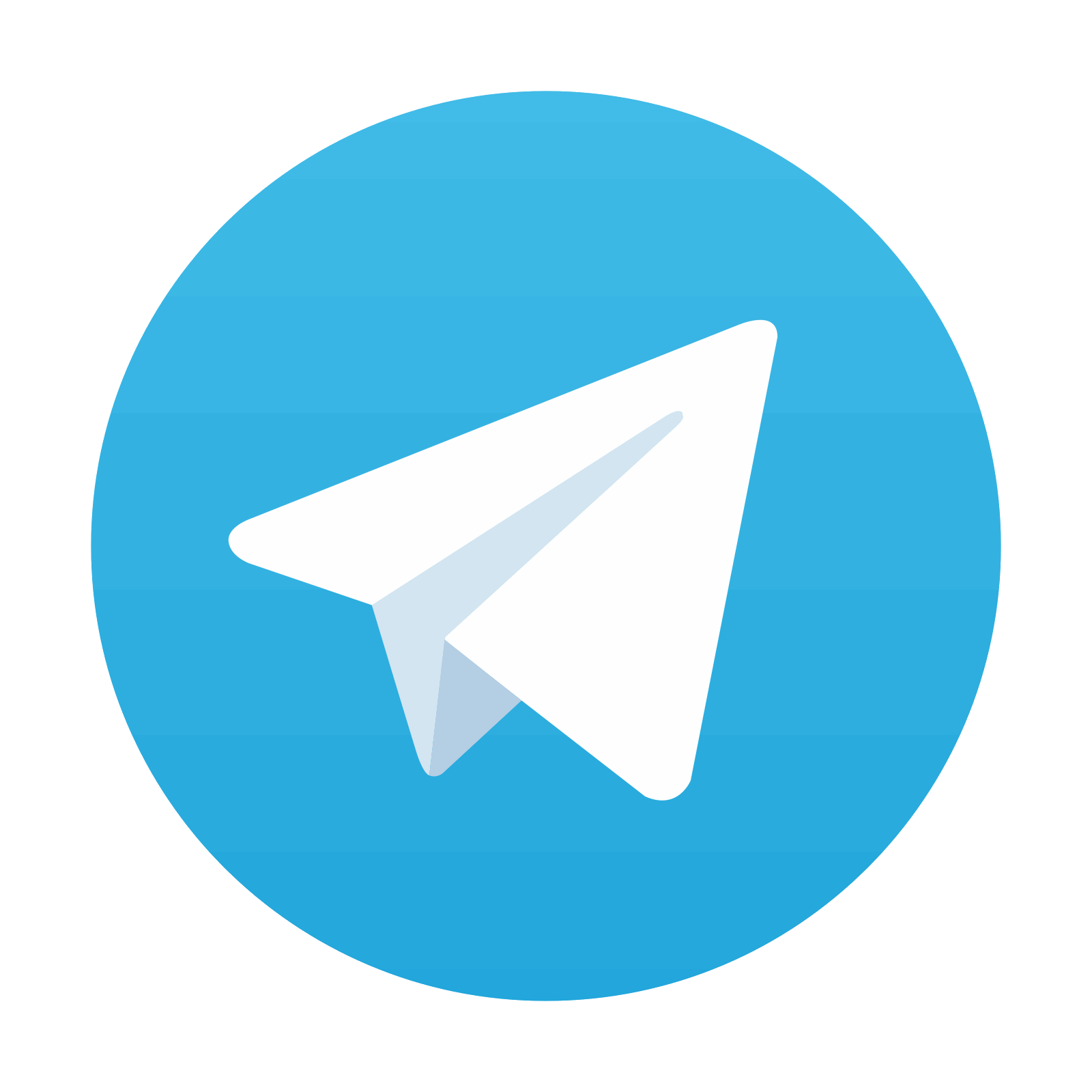
Stay updated, free articles. Join our Telegram channel

Full access? Get Clinical Tree
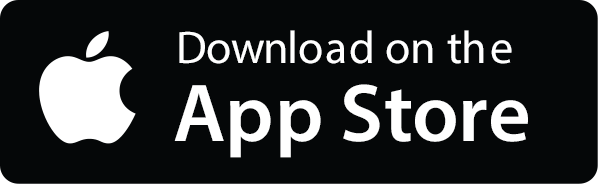
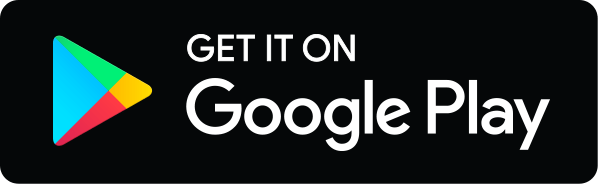