Coronary Risk Factors in Children
Stephen R. Daniels
Atherosclerosis
Coronary artery disease and stroke are the most common causes of morbidity and mortality in developed countries. The breakdown of the prevalence of different cardiovascular diseases in the United States is presented in Figure 71.1. Coronary heart disease alone caused ≈1 of every 6 deaths in the United States in 2010. In 2010, 379,559 Americans died of CHD. Each year, an estimated ≈620,000 Americans have a new coronary attack (defined as first hospitalized myocardial infarction or coronary heart disease death) and ≈295,000 have a recurrent attack. From 2000 to 2010, the relative rate of stroke death fell by 35.8% and the actual number of stroke deaths declined by 22.8%. Yet each year, ≈795,000 people continue to experience a new or recurrent stroke (ischemic or hemorrhagic). Overall, the trends have shown a decrease in cardiovascular death since 1970. Of note is the fact that cardiovascular disease mortality has declined faster in men than in women. This means that, at present, more women die of atherosclerotic heart disease than men each year (1).
Clearly, this is an important public health problem. In the past, atherosclerosis has been viewed as a problem of adults and has not been a focus in the pediatric age range. This is because the clinical manifestations of atherosclerosis are often not observed until middle age. However, there is increasing evidence that the process of atherosclerosis begins in childhood and is progressive throughout life.
Atherosclerosis results from deposits of lipid and cholesterol in the intima of the arterial wall. The earliest abnormality is thought to
be the fatty streak (Fig. 71.2). This is an accumulation of lipid-filled macrophages within the intima (2). These lesions are flat and do not obstruct the arterial lumen. The natural history of these lesions is that some progress to raised plaques. This is a result of continued lipid accumulation and a proliferation of macrophages and smooth muscle cells (3). In this lesion, smooth muscle–type cells form a fibrous cap over a deposition of necrotic debris, cholesterol crystals, and ultimately calcification within the arterial wall. It is these raised lesions that result in a myocardial infarction because of either their increasing size and obstruction of the arterial lumen or their rupture, which results in the release of thrombogenic substances from the necrotic core. It has been noted that the fibrous plaques tend to develop at the anatomic site where fatty streaks are formed in children (4). Plaques generally tend to develop in the coronary arteries prior to their appearance in the cerebral arteries.
be the fatty streak (Fig. 71.2). This is an accumulation of lipid-filled macrophages within the intima (2). These lesions are flat and do not obstruct the arterial lumen. The natural history of these lesions is that some progress to raised plaques. This is a result of continued lipid accumulation and a proliferation of macrophages and smooth muscle cells (3). In this lesion, smooth muscle–type cells form a fibrous cap over a deposition of necrotic debris, cholesterol crystals, and ultimately calcification within the arterial wall. It is these raised lesions that result in a myocardial infarction because of either their increasing size and obstruction of the arterial lumen or their rupture, which results in the release of thrombogenic substances from the necrotic core. It has been noted that the fibrous plaques tend to develop at the anatomic site where fatty streaks are formed in children (4). Plaques generally tend to develop in the coronary arteries prior to their appearance in the cerebral arteries.
The early stages of the atherosclerotic process are asymptomatic. The best understanding of this process has come from a series of pathology studies. The earliest pathology studies were performed during the Korean War and the war in Vietnam (5,6). The results of these studies were somewhat surprising in that young and healthy males were found to have both fatty streaks and more advanced raised lesions. Although these early pathology studies documented the presence of atherosclerosis, they did not establish the risk factors for the early stages of this process. This information would come from subsequent studies.
TABLE 71.1 Established Risk Factors for Coronary Artery Disease | ||
---|---|---|
|
There has been substantial epidemiologic research on the risk factors for cardiovascular disease development in adults. Longitudinal studies such as the Framingham study have measured potential risk factors and followed subjects to the development of cardiovascular disease. In fact, investigators have proposed >200 potential risk factors for the development of coronary artery disease. Most of these proposed risk factors have come from cross-sectional correlation studies. However, establishing a causal relationship is substantially more difficult. This requires multiple studies including some with a longitudinal design with follow-up to the cardiovascular end point. It has also been difficult to establish the independence of a particular risk factor because often associations exist among risk factors. After decades of research, a group of risk factors, often referred to as the traditional risk factors, has been established. These risk factors are presented in Table 71.1. As can be seen, some
of the risk factors are potentially modifiable and others are not. Table 71.2 lists novel factors that have strong consideration but are not yet considered established as independent risk factors.
of the risk factors are potentially modifiable and others are not. Table 71.2 lists novel factors that have strong consideration but are not yet considered established as independent risk factors.
TABLE 71.2 Possible Risk Factors for Atherosclerosis and Coronary Artery Disease | ||||||
---|---|---|---|---|---|---|
|
The risk factors for the early aspects of the process of atherosclerosis have been determined by the more recent pathology studies including the Bogalusa study and the Pathobiological Determinants of Atherosclerosis in Youth (PDAY) study. The PDAY study was a multicenter investigation of individuals aged 15 to 34 years who died of accidental causes and suicide unrelated to the known presence of cardiovascular disease (7,8). The investigators performed autopsies to evaluate the extent of atherosclerosis in the aorta and coronary arteries. They used various indicators of risk factor status obtainable at the time of autopsy to define risk. They found that the traditional risk factors, including dyslipidemia, blood pressure elevation, and obesity, were associated with the presence of fatty streaks and of fibrous plaques.
The Bogalusa study investigators were able to obtain autopsies in individuals who had been participants in a school-based risk factor study, were followed longitudinally, and died of accidental causes (9,10). The investigators in the Bogalusa study found that the percent of the surface of the arteries covered with fatty streaks and fibrous plaques increased with increasing age at the time of death. They also found that the extent of coverage of the arteries (aorta and coronary arteries) was associated with elevation of total cholesterol, low-density lipoprotein cholesterol (LDL-C), triglycerides, blood pressure, and body mass index (BMI) during the school-age surveys. Low high-density lipoprotein cholesterol (HDL-C) was also associated with a greater extent of coverage of the arterial surface with fatty streaks and fibrous plaques. An additional important finding was that the prevalence of atherosclerosis increased with an increasing number of risk factors present. This was particularly true for fibrous plaques in the coronary arteries where the presence of three or four risk factors was associated with 7% coverage and the presence of one or two risk factors was associated with 1% and 2% coverage, respectively (10).
Imaging
One factor that has made the study of atherosclerosis difficult is the lack of noninvasive tools to image the early atherosclerotic lesions. In adults, computed tomography (CT) scanning has been used to detect the presence of calcium in the coronary arteries. The presence of calcium deposits has been associated with increased risk for adverse cardiovascular disease outcomes (11). Fewer studies have evaluated this issue in young subjects. Investigators from the Muscatine Study used electron beam CT to evaluate young adults who had participated in a longitudinal epidemiologic study of cardiovascular risk factors as children (12). The subjects were younger than 35 years of age. The prevalence of coronary artery calcium was 31% in men and 10% in women.
The investigators also evaluated the extent to which risk factors were associated with the presence of coronary artery calcium. They found that the risk factors measured most recently were the strongest predictors including systolic blood pressure, BMI, LDL-C, and HDL-C. However, weight in childhood and BMI, diastolic blood pressure, and cholesterol in young adulthood were also associated with increased risk of calcium in the coronary arteries (12).
Gidding et al. (13) studied a group of adolescents and young adults aged 11 to 23 years with heterozygous familial hypercholesterolemia. They found that 7 of 29 subjects had coronary artery calcium. Calcium was more likely to be present when obesity and cholesterol elevation were both present.
Magnetic resonance imaging (MRI) may also be used to evaluate the atherosclerotic process. It has been used to evaluate the presence of atherosclerotic plaques and supravalvar aortic stenosis in young patients with homozygous familial hypercholesterolemia (14). Studies in adults have also demonstrated that high-resolution multicontrast MRI can be used to evaluate whether arterial plaque is unstable and subject to rupture (15). This is important as other noninvasive methods have been unable to characterize this progression of atherosclerosis. This method allows the fibrous cap to be distinguished from the lipid core. It is not currently known if MRI will be useful in following the evolution of fatty streaks to fibrous plaques.
Ultrasound methodology has also been used to evaluate the presence of atherosclerosis. In adults, the measurement of the carotid artery intima-media thickness (IMT) has gained acceptance as a useful method in epidemiologic studies to evaluate atherosclerosis. In adults, it has been shown that increased carotid IMT is associated with cardiovascular risk factors (15) and with incident myocardial infarction (16,17) and stroke (18). However, in a meta-analysis of 41 randomized clinical trials, Costanzo et al. (19) found that regression or slowed progression of carotid IMT induced by cardiovascular drug therapies in adults does not reflect reduction of cardiovascular events. Fewer studies have used ultrasound to evaluate carotid IMT in children and adolescents. Davis et al. (20) studied the young adult Muscatine population aged 33 to 42. These were individuals who had participated in the school surveys when they were younger. In males, childhood total cholesterol and triglycerides were higher in those with elevated carotid IMT. In females, childhood weight, BMI, total cholesterol, and triglycerides were higher in those with high carotid IMT. The Young Finns study had a similar design (21). In multivariable models adjusting for age and sex, they found that childhood LDL-C, systolic blood pressure, BMI, and cigarette smoking were associated with increased carotid IMT in young adulthood. Sanchez et al. (22) performed a study of high school students. They found relationships between LDL-C, HDL-C, systolic blood pressure, diastolic blood pressure, and BMI with carotid artery IMT. Other studies have also found that children with elevated cholesterol levels have significantly elevated carotid artery IMT compared with controls (23). Sorof et al. (24) evaluated 32 children with hypertension. They found a significant association between carotid artery IMT and left ventricular mass after adjustment for age, sex, and BMI. In those patients with hypertension and increased carotid artery IMT, the prevalence of left ventricular hypertrophy was 89% compared with 25% in those with normal carotid artery IMT. Jarvisalo et al. (25) reported a significant association between C-reactive protein (CRP), which is a marker of inflammation, and carotid artery IMT. They also found an association between elevated CRP and impaired brachial artery flow-mediated dilation, which is a measure of endothelial function.
Juonala et al. (26) studied the relationship of cardiovascular risk factors measured in childhood with carotid IMT in adulthood in four large cohort studies: the Cardiovascular Risk in Young Finns Study, the Childhood Determinants of Adult Health Study, the Bogalusa Heart Study, and the Muscatine Study. They found significant relationships between childhood risk factors and adult carotid IMT that were dependent on the age at which childhood risk factors were measured. These data demonstrated
that risk factor measurements obtained at age 9 years or older are predictive of subclinical atherosclerosis in adulthood.
that risk factor measurements obtained at age 9 years or older are predictive of subclinical atherosclerosis in adulthood.
Thus, it does appear that evaluation of the carotid arteries using ultrasound is a useful marker of preclinical atherosclerosis in children and adolescents (27). This method is currently used only in the research setting, but as it is studied in more detail, it may also ultimately be useful as a clinical test. In the meantime, other modalities are being investigated and may prove useful in the future.
Risk Factors for Atherosclerosis
Several risk factors have been established as being important in the development of atherosclerosis and ultimately in the occurrence of myocardial infarction and cerebrovascular disease. Recent research has focused on the lifetime exposure to risk factors and their impact on clinical cardiovascular outcomes. It is becoming clear that establishing and maintaining low risk across a number of behavioral factors, such as cigarette smoking, diet and physical activity and biologic risk factors, such as elevated cholesterol, blood pressure and fasting blood glucose has a very powerful impact on cardiovascular disease development. For example, Berry et al. showed that maintaining low or optimal risk is associated with a 4.7% risk of mortality from cardiovascular disease in men and a 6.4% risk in women compared to a 29.6% and 20.5% risk of mortality through age 80 years, respectively when risk factors are present (28). This suggests the importance of primordial prevention in children and adolescents. Most children and adolescents are born with optimal risk factors. It is maintaining this low-risk status over time through healthful behaviors that is quite important, but difficult. Shay et al. examined the prevalence of low, intermediate and optimum risk factor status in adults (29) and in adolescents (30). They found that fewer than 1% of adults exhibit optimum risk factor status (29). Unfortunately, the experience for adolescents is not much better (30). Only 16.4% of males and 11.3% of females met optimal criteria for six out of seven risk factors and, essentially, no adolescents were at ideal status on all seven risk components. This means that the very valuable commodity of low risk for cardiovascular disease, which is present at birth, is lost over time due to unhealthy behaviors and accumulation of risk factors starting in childhood and adolescence. Dyslipidemia and hypertension are reviewed in detail in sections “Lipids and Lipoproteins” and “Hypertension.” Other risk factors are discussed here briefly.
Diabetes
Diabetes is well established as a major risk factor for cardiovascular disease in adults (31). In the past, most pediatric patients with diabetes had type 1 diabetes mellitus. This was different from the experience with adults in whom the prevalence of type 2 diabetes mellitus was much higher. However, with the increasing prevalence and severity of obesity in the pediatric population, the prevalence of type 2 diabetes has increased dramatically (32). This is of critical importance from the standpoint of cardiovascular disease development. In adults, type 2 diabetes is responsible for more cases of renal failure and peripheral vascular disease than any other disease process (33). The risk of cardiovascular disease in patients with diabetes is increased by as much as fivefold compared with individuals without diabetes (33). It has been estimated that 70% of adult patients with type 2 diabetes die of cardiovascular disease (34), and the 10-year mortality rate for patients with type 2 diabetes is approximately 10 times higher than in a nondiabetic comparison group, with most deaths occurring as a result of coronary artery disease (35,36).
The predisposition to cardiovascular disease in patients with diabetes cannot be overemphasized. This is underscored by the recommendations of the American Diabetes Association (36) and the National Cholesterol Education Program (NCEP) (37,38), which consider the presence of diabetes to be a coronary artery disease risk equivalent. This means that patients with diabetes should be treated with the same aggressive approach to risk factor management that would be recommended in a patient who already has established coronary artery disease or who has had a myocardial infarction.
The risk status for cardiovascular disease in adolescents with type 2 diabetes is not well understood. However, if the progression of atherosclerosis is similar to that seen in adults, it can be anticipated that these patients may develop clinically apparent cardiovascular disease as early as their late thirties and early forties. Unfortunately, because so little is known about the progression of cardiovascular disease in young patients with type 2 diabetes, it is difficult to make evidence-based decisions regarding the optimum clinical strategies to prevent cardiovascular disease. Maahs et al. have reviewed the development of risk for cardiovascular disease in youth with type 1 and type 2 diabetes mellitus (39). A study from Australia documents greater risk of early mortality in those who have onset of type 2 diabetes in youth compared to those with type 1 diabetes (40).
Some results regarding the relationship of diabetes and cardiovascular abnormalities have begun to emerge. Shah et al. (41) evaluated cardiac structure and function in adolescents with type 2 diabetes and those with obesity alone. They found that adolescents with obesity and with obesity-related type 2 diabetes had changes in cardiac geometry consistent with cardiac remodeling. Both groups also had decreased diastolic function when compared to lean controls with the greatest decrease in those with type 2 diabetes.
Urbina et al. (42) reported increased arterial stiffness, a marker for the development of atherosclerosis, in both adolescents with obesity and those with type 2 diabetes mellitus after controlling for other risk factors. Wadwa et al. (43) studied adolescents with type 1 and type 2 diabetes mellitus. They found that youth with type 2 diabetes had increased arterial stiffness compared to those with type 1 diabetes. In their study, increased central adiposity and blood pressure were associated with increased arterial stiffness independent of the type of diabetes. Urbina et al. (44) studied carotid IMT and stiffness in relation to obesity and type 2 diabetes and found that abnormalities in carotid thickness and stiffness are only partially explained by traditional cardiovascular risk factors. This suggests that more research is needed to understand additional factors that may be related to this process. Shah et al. (45) reported that longer duration of diabetes and poorer glycemic control both have an independent association with carotid IMT in adolescents with type 2 diabetes. These results emphasize the need for improved blood glucose control to prevent the progression of cardiovascular disease in patients with type 2 diabetes.
Additional research will be needed to better define the optimum clinical approaches to young patients with type 2 diabetes. Nevertheless, it is important to manage the diabetes with appropriate weight management and blood glucose control methods. It is also important to evaluate cardiovascular risk factors in patients with diabetes and treat those risk factors when present.
Cigarette Smoking
Cigarette smoking is a major independent risk factor for cardiovascular disease (46). Although prevention of cigarette smoking is of the greatest importance, it has also been shown that cessation of smoking can provide a benefit by reducing risk of cardiovascular and lung disease. Investigators from the Multiple Risk Factor Intervention Trial (MRFiT) have reported that cessation of smoking will reduce the risk of the development of cardiovascular disease (47). This reduction of risk begins in the first year after cessation and continues to further reduction as long as 3 years after cessation.
In adolescents, atherosclerotic lesions have been seen with increased prevalence in cigarette smokers as young as 15 years of
age. In addition, LDL-C is increased and HDL-C is decreased in adolescent smokers compared with their nonsmoking counterparts (48). Chronic cigarette smoking may lead to injury of the endothelium, which serves as the nidus for the development of atherosclerosis. It has been estimated that of smoking-related deaths, cardiovascular disease is involved in over one-third, and this process often begins early in life (49).
age. In addition, LDL-C is increased and HDL-C is decreased in adolescent smokers compared with their nonsmoking counterparts (48). Chronic cigarette smoking may lead to injury of the endothelium, which serves as the nidus for the development of atherosclerosis. It has been estimated that of smoking-related deaths, cardiovascular disease is involved in over one-third, and this process often begins early in life (49).
Most individuals who become regular smokers initiate cigarette smoking in childhood and adolescence. Overall, approximately one of five high school students becomes a regular smoker. Many adolescents, while experimenting with smoking, believe that they can control their use of cigarettes. Unfortunately, this is not the case as many cannot quit smoking and continue to smoke on a regular basis. During the period from 1997 to 2003, overall smoking prevalence declined in high school students from >27% to 22% (50). Unfortunately, the prevalence of smoking in girls has increased over time, so now the prevalence is closer to equal for boys and girls (51). In 2009, the prevalence of cigarette smoking in adolescent high school students was 17.2% overall, 19.6% for adolescents boys, and 14.8% for girls (51). The major influences on initiation of smoking appear to be parents and peers smoking regularly (52,53). It has been shown that parent discussion of smoking, rules against smoking, and punishment for use of cigarettes all have a beneficial effect on decreasing adolescent smoking (54,55). Of greatest importance is that adolescents are significantly less likely to initiate smoking when parents quit smoking (56). Studies have also demonstrated an inverse association between physical activity and smoking, suggesting that an increased level of physical activity may protect against smoking initiation (57). These epidemiologic study results suggest important approaches to the prevention of the onset of cigarette smoking. Efforts of prevention should begin in elementary and middle school students because many children are already experimenting with cigarette smoking by age 10 years (58).
Exposure to environmental tobacco smoke may also be associated with increased risk of cardiovascular disease. Moskowitz et al. (59) reported lower HDL-C in boys exposed to environmental tobacco smoke compared with those who were not exposed. Weitzman et al. (60) reported a dose–response relationship between exposure to tobacco smoke measured by serum cotinine and presence of the metabolic syndrome in 12- to 19-year-olds in the National Health and Nutrition Examination Survey III (NHANES III). In this population-based cohort, the prevalence of metabolic syndrome was 1.2% for nonexposed, 5.4% for those exposed to environmental tobacco smoke, and 8.7% for active smokers (60). The prevalence of metabolic syndrome for overweight adolescents was 19.6 for those exposed to environmental tobacco smoke and 23.6 for active smokers. These results emphasize that elimination of cigarette smoking in the household may have a dual benefit by directly reducing cardiovascular risk and by decreasing the risk for initiating active smoking. One of the most striking public health results comes from studies that show that banning smoking in public places, such as restaurants and bars, resulted in a dramatic decline in cardiovascular disease mortality (61,62). These results suggest that exposure to environmental tobacco smoke has a substantial deleterious effect. We are now seeing the emergence of electronic cigarettes. These are battery powered devices that, upon inhalation, activate a pressure sensitive circuit that heats an atomizer and turns liquid, including nicotine, into an aerosol that is inhaled. In general, the health effects of electronic cigarettes have not been well studied. Propylene glycol is a major ingredient in the liquid used in electronic cigarettes. It is generally considered nontoxic, but some products may have diethylene glycol and other contaminants, which may be more problematic. There are still concerns about the effects of nicotine, when it is used acutely and chronically, on the cardiovascular system. Nicotine increases heart rate, blood pressure, and causes vasoconstriction of coronary and other vascular beds (63). Nicotine use has also been associated with endothelial dysfunction (63). The American Heart Association has issued a policy statement on electronic cigarettes that is helpful in considering this potential impact on cardiovascular disease outcomes (64). Clearly, more research is needed in this area in the future.
Obesity/Metabolic Syndrome
The prevalence of obesity in children more than tripled from 1980 to 2006. Since then, the prevalence appears to have leveled off and perhaps even declined slightly in some areas. The prevalence nationally is approximately 17% for both children and adolescents (65), but is greater in some gender/ethnicity groups. This increase in prevalence of overweight or obesity appears to be occurring worldwide.
The Muscatine and Bogalusa studies have shown that obesity in childhood and adolescence is associated with several risk factors for cardiovascular disease including atherogenic dyslipidemia (elevated triglycerides and low HDL-C), hypertension, left ventricular hypertrophy, atherosclerosis, and obstructive sleep apnea (10,66,67). When a child or adolescent is evaluated for overweight, it is important that assessment of cardiovascular risk factors be included as part of the evaluation.
Children and adolescents should be evaluated for overweight by calculating the BMI. This should then be compared with age- and sex-specific percentiles. Obesity is defined as a BMI ≥95th percentile. BMI between the 85th and 95th percentiles is considered overweight (68). Treatment of overweight is difficult but can be accomplished by behavioral, pharmacologic, and surgical approaches (69,70,71,72). The US Preventive Services Task Force has performed a systematic review of pediatric weight management programs (73). They concluded that the available research supports at least short-term benefits of comprehensive medium- to high-intensity behavioral interventions in obese children and adolescents. They also found no adverse impact of comprehensive weight management programs.
Barlow et al. (74) have presented recommendations for prevention, assessment, and treatment of obesity in children and adolescents. These recommendations include a graded approach to weight management depending on the level of BMI and the presence of comorbid conditions, such as diabetes, hypertension, dyslipidemia, obstructive sleep apnea, and nonalcoholic fatty liver disease.
TABLE 71.3 Factors Considered Part of the Metabolic Syndrome | |||||||||
---|---|---|---|---|---|---|---|---|---|
|
An important consideration with obesity is the metabolic syndrome. This is a clustering of risk factors for diabetes and cardiovascular disease that is frequently observed in patients who are overweight, particularly with an increased central distribution of fat (75). Factors that are often included in the metabolic syndrome are listed in Table 71.3. Many definitions of the metabolic
syndrome have been proposed for clinical use in adults. There have also been proposed definitions for children and adolescents. Most investigators have chosen to adopt a schema similar to that used in adults but have used age- and sex-specific percentiles to define clinical cutpoints (76,77,78). However, investigators have chosen a wide range of cutpoints. This often results in a discontinuity between the child/adolescent definition and the adult definition. As might be expected, the choice of different risk factors and different cutpoints results in very different estimates of the prevalence of metabolic syndrome. One approach has been to use the variables in the metabolic syndrome complex as continuous variables rather than defining cutpoints (79). A particular concern about the metabolic syndrome is that it appears not to be a stable diagnosis throughout adolescence with some individuals gaining the diagnosis and others losing it over time (80). Further research is necessary to determine the optimum definition of the metabolic syndrome (81). Nevertheless, clinicians should be well aware of the clustering of cardiovascular risk factors that occurs with obesity. These factors should be evaluated in the child with obesity, and the risk factors should be treated when abnormalities are found. An important issue is whether resolution of obesity, once it has occurred in a child or adolescent, will lower risk of cardiovascular disease. Juonala et al. studied longitudinal data from four prospective cohort studies (82). They defined four groups. First, a group that remained at a normal body mass index from childhood to adulthood. Second, a group that was obese in childhood and remained obese into adulthood. Third, a group that was lean in childhood and became obese in adulthood. Fourth, a group that was obese in childhood, but achieved normal BMI as an adult. They found that the groups that were obese as adults had an increased risk of type 2 diabetes, hypertension, elevated LDL-C, reduced HDL-C, elevated triglyceride levels, and increased carotid IMT. However, those who were obese in childhood, but became nonobese as an adult, had risk of those outcomes that was similar to those who maintained a normal BMI from childhood to adulthood. These results are quite encouraging and emphasize that treatment for overweight and obese children and adolescents is quite important.
syndrome have been proposed for clinical use in adults. There have also been proposed definitions for children and adolescents. Most investigators have chosen to adopt a schema similar to that used in adults but have used age- and sex-specific percentiles to define clinical cutpoints (76,77,78). However, investigators have chosen a wide range of cutpoints. This often results in a discontinuity between the child/adolescent definition and the adult definition. As might be expected, the choice of different risk factors and different cutpoints results in very different estimates of the prevalence of metabolic syndrome. One approach has been to use the variables in the metabolic syndrome complex as continuous variables rather than defining cutpoints (79). A particular concern about the metabolic syndrome is that it appears not to be a stable diagnosis throughout adolescence with some individuals gaining the diagnosis and others losing it over time (80). Further research is necessary to determine the optimum definition of the metabolic syndrome (81). Nevertheless, clinicians should be well aware of the clustering of cardiovascular risk factors that occurs with obesity. These factors should be evaluated in the child with obesity, and the risk factors should be treated when abnormalities are found. An important issue is whether resolution of obesity, once it has occurred in a child or adolescent, will lower risk of cardiovascular disease. Juonala et al. studied longitudinal data from four prospective cohort studies (82). They defined four groups. First, a group that remained at a normal body mass index from childhood to adulthood. Second, a group that was obese in childhood and remained obese into adulthood. Third, a group that was lean in childhood and became obese in adulthood. Fourth, a group that was obese in childhood, but achieved normal BMI as an adult. They found that the groups that were obese as adults had an increased risk of type 2 diabetes, hypertension, elevated LDL-C, reduced HDL-C, elevated triglyceride levels, and increased carotid IMT. However, those who were obese in childhood, but became nonobese as an adult, had risk of those outcomes that was similar to those who maintained a normal BMI from childhood to adulthood. These results are quite encouraging and emphasize that treatment for overweight and obese children and adolescents is quite important.
Physical Activity
Strong et al. (83) have evaluated the effects of physical activity on health in school-aged youth. They identified a number of cardiovascular health issues that are related to diminished physical activity. Cardiovascular fitness has been identified as a risk factor for cardiovascular disease in adults (84). Physical fitness probably has both genetic and environmental influences. Strong et al. (83) identified several correlational studies indicating an association between low levels of vigorous physical activity and low levels of cardiovascular fitness. Experimental studies showed that exercise training can improve cardiovascular fitness in children 8 years of age and older. Generally, the successful programs included continuous vigorous exercise for >30 minutes per session at a minimum of 3 days per week.
Epidemiologic studies in children have generally shown a weak association between the level of physical activity and lipids and lipoproteins. The strongest associations are usually seen with HDL-C (85). The results of studies evaluating the relationship of cardiovascular fitness to lipids and lipoproteins mostly do not show a significant correlation. Intervention studies show a weak but beneficial effect primarily in improvement of triglyceride and HDL-C concentrations, usually in the context of improvement of BMI. One of the reasons for inconsistent results is the fact that different studies used different levels of intensity of physical activity with different frequencies of exercise episodes and different durations of treatment. From the available data, it appears that a minimum of 40 minutes of activity per day 5 days per week for 4 months is required to result in lower triglyceride and increased HDL-C levels (85).
Isometric or resistance exercise may also have a benefit in raising HDL-C levels. Goldberg et al. (85) found decreased LDL-C and increased HDL-C in adolescent boys who underwent a 9-week resistance exercise training program. These improvements were not related to changes in weight or adiposity.
There is no clear association between exercise and lower blood pressure in children with normal blood pressure (86). On the other hand, in adolescents with hypertension, aerobic activity programs of 12 to 32 weeks’ duration have been shown to have a blood pressure–lowering effect. Strength training appears to have little effect on blood pressure in children with hypertension.
These results suggest that children and adolescents with essential hypertension should be encouraged to engage in aerobic activity on a regular basis. It is necessary to continue the physical activity to maintain the beneficial effect. An important aspect of the impact of physical activity is that it is also useful in management of overweight. Because many cardiovascular risk factors cluster with overweight, improvement in BMI is an important mechanism of action for increased physical activity. It has been shown that programs of moderate intensity lasting 30 to 60 minutes per episode with three to seven episodes per week can lead to a reduction in both total body and visceral adiposity in children and adolescents (87). Thus, it is clear that there are numerous beneficial effects to increasing the level of physical activity in children. This may also establish better exercise habits that will last into adulthood.
Summary
There has been increasing interest in the improvement of cardiovascular health (88). There is evidence that individuals who are able to maintain low-risk status with optimum levels of cardiovascular disease risk factors through childhood, adolescence, and adulthood to age 50 have a very low lifetime risk of cardiovascular disease (87). This puts the focus on primordial prevention, which is the prevention of development of risk factors in the first place. Unfortunately, currently the prevalence of ideal cardiovascular health is quite low. Bambs et al. (88) evaluated cardiovascular health in a cohort of 1,933 individuals (mean age 59 years, 44% African Americans, 66% women). They found that only 0.1% met the criteria of seven risk factors and behavioral components. Less than 10% met ≥5 of these components. Thus, the prevalence of ideal cardiovascular health is currently quite low in a community-based sample of middle-aged adults. While there are genetic factors involved, there are clearly behavioral and lifestyle factors that are quite important. It is increasingly clear that pediatricians, family physicians, and pediatric cardiologists must play a critical role in developing and maintaining cardiovascular health. It is also clear that achievement of this will pay great dividends.
Atherosclerosis is a slow but progressive process that begins in childhood and progresses throughout adolescence and into adulthood. There are numerous risk factors for atherosclerosis in children, which generally parallel the risk factors in adults. Pediatricians and pediatric cardiologists should take an integrated approach to the prevention of cardiovascular disease and atherosclerosis (89,90). This type of approach will be necessary for reducing death and disability from cardiovascular disease, which is the major cause of death in the United States.
Lipids and Lipoproteins
Lipids are organic compounds that are not soluble in water but are soluble in organic solvents. Plasma lipids are transported by lipoproteins. This combination of lipids (including cholesterol triglycerides and phospholipids) and protein (called apolipoproteins) allows the lipid component to become soluble in water and blood.
Lipids are important components of cell membranes and also serve as building blocks for some hormones.
Lipids are important components of cell membranes and also serve as building blocks for some hormones.
TABLE 71.4 Lipids, Lipoproteins, and Apolipoproteins | ||||||||||||||||||||||||||||||
---|---|---|---|---|---|---|---|---|---|---|---|---|---|---|---|---|---|---|---|---|---|---|---|---|---|---|---|---|---|---|
|
The principal apolipoproteins are A-1, A-II, B-100, and C-II. Apolipoproteins have several functions. First, they bind with specific receptor sites in cells. They also are cofactors for enzymes involved in lipid metabolism such as lecithin cholesterol acyltransferase and lipoprotein lipase. Third, they function as structural protein for the biosynthesis and secretion of plasma lipoproteins. For example, Apo A-1 has been proposed as an important structural protein for the biosynthesis of HDL.
There are four major classes of lipoproteins including chylomicrons, very low-density lipoproteins (VLDLs), low-density lipoproteins (LDLs), and high-density lipoproteins (HDLs) (Table 71.4).
Chylomicrons are triglyceride-rich particles produced by the intestine. They are the largest lipoprotein. Their primary function is to transport cholesterol and triglycerides from the diet to sites for metabolism or storage (91). Chylomicrons are usually not present during fasting and normally are rapidly cleared after a meal. The clearance occurs as a result of lipoprotein lipase, which creates remnants of chylomicrons. These remnants are thought to be atherogenic and are cleared by the liver.
VLDL particles are also relatively large particles. VLDL is produced by the liver. VLDL transports triglycerides and cholesterol, which is endogenously synthesized, to the periphery (92).
LDL is the major carrier of cholesterol to peripheral tissues. LDL is made up of 45% cholesterol. LDL particles are found in atherosclerotic plaque and are associated with increased risk of cardiovascular disease (93). In particular, small dense LDL particles are considered especially atherogenic. LDL particles are recognized by specific receptors on the cell wall. When LDL particles bind to the receptor on the cell wall, they are then internalized into the cell. It has been estimated that approximately 75% of LDL particles are removed by binding with receptors, whereas the remaining particles are removed by macrophages (Fig. 71.3).
HDLs can be produced in several ways. They can be produced by the liver and the gastrointestinal tract. HDL particles are also produced by catabolism of chylomicrons and VLDL particles. HDL particles are also thought to be heterogeneous.
HDL2 is the subfraction that is thought to be most protective against atherosclerosis (94).
HDL2 is the subfraction that is thought to be most protective against atherosclerosis (94).
TABLE 71.5 Lipid and Lipoprotein Distributions in Children Aged 5 to 19 Years | |||||||||||||||||||||||||||||||||||||||||||||||||||||||||||||||||||||||||||||||||||||||||||||||||||||||||||||||||||||||||||||||||||||||||||||||||||||||||||||||||||||||||||||||||||||||||||||||||||||||||||||||||||||||||||||||||||||||||||||||||||||||||||||||||||||||||||||||||||||||||||
---|---|---|---|---|---|---|---|---|---|---|---|---|---|---|---|---|---|---|---|---|---|---|---|---|---|---|---|---|---|---|---|---|---|---|---|---|---|---|---|---|---|---|---|---|---|---|---|---|---|---|---|---|---|---|---|---|---|---|---|---|---|---|---|---|---|---|---|---|---|---|---|---|---|---|---|---|---|---|---|---|---|---|---|---|---|---|---|---|---|---|---|---|---|---|---|---|---|---|---|---|---|---|---|---|---|---|---|---|---|---|---|---|---|---|---|---|---|---|---|---|---|---|---|---|---|---|---|---|---|---|---|---|---|---|---|---|---|---|---|---|---|---|---|---|---|---|---|---|---|---|---|---|---|---|---|---|---|---|---|---|---|---|---|---|---|---|---|---|---|---|---|---|---|---|---|---|---|---|---|---|---|---|---|---|---|---|---|---|---|---|---|---|---|---|---|---|---|---|---|---|---|---|---|---|---|---|---|---|---|---|---|---|---|---|---|---|---|---|---|---|---|---|---|---|---|---|---|---|---|---|---|---|---|---|---|---|---|---|---|---|---|---|---|---|---|---|---|---|---|---|---|---|---|---|---|---|---|---|---|---|---|---|---|---|---|---|---|---|---|---|---|---|---|---|---|---|---|---|---|---|---|---|---|
|
Normal Levels of Lipids and Lipoproteins
Normal values and distribution for plasma lipids and lipoproteins in American children have been published (95). These values have generally been used in subsequent guidelines to characterize children from a clinical perspective. These values are presented in Table 71.5.
Overall, total and LDL-C are lower in males than in females for most age groups. In children 5 to 10 years of age, HDL-C is lower in girls than in boys. It can be seen that HDL levels then gradually increase in girls and decrease in boys. The changes in concentration of lipids and lipoproteins during puberty can be important from a clinical perspective (96). LDL-C often declines during this period by as much as 10% to 20%. This means that some adolescents may experience a decline from an abnormal value to normal for a period of time. However, in the latter part of puberty, the LDL-C begins to rise and often continues to rise through adulthood. The decline in HDL-C for males during puberty has been shown to extend into the adult ages. In young adults, HDL-C levels are approximately 10 mg/dL higher in women compared with men (38).
Epidemiology
Longitudinal studies such as the Framingham study and the MRFiT study have shown that the level of cholesterol in the blood is an important and independent predictor of coronary heart disease (97,98). It has been estimated that for each 1% increase in cholesterol, there is an approximately 3% increase in risk of cardiovascular disease (99). Studies have also consistently shown that higher levels of HDL-C are associated with a lower risk of cardiovascular disease (100).
There are international differences in levels of plasma cholesterol. In general, there are genetic and environmental influences on plasma cholesterol levels. The nutritional component most related to these differences appears to be the intake of saturated fat. In countries where intake of saturated fat in the diet is low, the blood total cholesterol levels are also low and the incidence of coronary heart disease is low (101).
Lipids and lipoproteins have been demonstrated to track over time. Tracking is an epidemiologic concept in which an individual retains their ranking with respect to their peers over time. For cholesterol, this means that, if tracking is present, a child with an elevated LDL-C will be more likely to become an adult with elevated LDL-C. Conversely, children with low levels relative to their peers will remain low over time. Epidemiologic studies of children and adolescents have shown that cholesterol levels do track, but
that rank order is not maintained as consistently as it is for height and weight (102,103). In a separate analysis of the data from the Muscatine Study, Lauer et al. (66) evaluated factors that affect the relationship between cholesterol levels in childhood and adulthood. They found that cholesterol levels during childhood were important, but that obesity development, cigarette smoking, and the use of oral contraceptives in women had deleterious effects on cholesterol levels in adulthood.
that rank order is not maintained as consistently as it is for height and weight (102,103). In a separate analysis of the data from the Muscatine Study, Lauer et al. (66) evaluated factors that affect the relationship between cholesterol levels in childhood and adulthood. They found that cholesterol levels during childhood were important, but that obesity development, cigarette smoking, and the use of oral contraceptives in women had deleterious effects on cholesterol levels in adulthood.
Magnussen et al. (104) evaluated longitudinal data from the Childhood Determinants of Adult Health Study in which children in Australia were followed from 1985 until follow-up as young adults in 2004. They found that lifestyle changes that occur between youth and adulthood influence whether an individual maintains, loses, or develops high-risk blood lipid and lipoprotein levels in adulthood. The factors that are most important are excess weight gain, physical inactivity, and cigarette smoking.
Factors Causing Dyslipidemia
Genetic
Lipid synthesis and metabolism is quite complex. Because there are numerous steps in the processes, they are vulnerable to genetic abnormalities that lead to dyslipidemia.
The most important and well-understood genetic abnormality is familial hypercholesterolemia. Brown and Goldstein (105) have described the LDL-C receptors that are associated with familial hypercholesterolemia. They have now described numerous mutations of the genes for the LDL receptor, which is a membrane glycoprotein. The most common LDL-C receptor gene mutations often result in a situation where the LDL receptor protein is produced only in small amounts. Other mutations result in the synthesis of precursors of the receptor that are not converted to the complex endoglycosidase H-resistant form. In these mutations, the receptors remain in the endoplasmic reticulum and are not available on the cell surface. A third type of mutation results in receptors that are expressed on the cell surface, but are unable to bind effectively with LDL-C. A fourth kind of mutation results in receptors that are on the cell surface and can bind with LDL-C but are unable to undergo receptor-mediated endocytosis (106). There is also a less common gain of function mutation in PCSK9 that results in the rapid disposal of receptors, making them less accessible to bind with LDL-C (107). These mutations all result in the phenotype of elevation of plasma LDL-C.
Genetic abnormalities causing dyslipidemia are important to understand because they increase understanding of underlying mechanisms, and the prevalence for these disorders is relatively high. The prevalence of the homozygous form of familial hypercholesterolemia is 1 in 1,000,000. The prevalence of the heterozygous form of familial hypercholesterolemia is 1 in 500 (108). In some populations, the heterozygous form is as common as 1 in 300. The heterozygous state is generally fully expressed in the pediatric age range, and it is characterized by total and LDL cholesterol levels of approximately 300 and 240 mg/dL, respectively. These levels may fall somewhat during pubertal maturation. Children and adolescents with the homozygous form of familial hypercholesterolemia have total and LDL cholesterol in the range of 600 to 1,000 mg/dL and 450 to 850 mg/dL, respectively. Homozygous patients develop planar xanthomas (orange-colored skin lesions found on extensor surfaces) by the age of 5 years and develop coronary artery disease between the ages of 10 and 20 years. Patients with homozygous familial hypercholesterolemia often also develop aortic stenosis (109). Patients with the heterozygous form rarely have xanthomas in adolescence. However, they are at risk for coronary artery disease between ages 30 and 50 years. Among individuals who have had a myocardial infarction prior to age 60 years, approximately 5% have the heterozygous form of familial hypercholesterolemia (110).
There are also genetic abnormalities that lead to low HDL-C. These may be due to a primary abnormality in the metabolism of HDL and its major apolipoprotein Apo A-1. This is a heterogeneous group of abnormalities that result in low HDL-C but may or may not lead to premature coronary artery disease (111).
There are also genetic abnormalities that lead to elevation of triglycerides. Familial hypertriglyceridemia is an autosomal dominant disorder but is often not expressed until adulthood (112). The development of obesity, however, can speed its expression. Lipoprotein lipase deficiency is a rare disorder that results in hypertriglyceridemia and can be a cause of pancreatitis and neurologic symptoms (113). The most important aspect of treatment of elevation of triglycerides is very aggressive restriction of dietary fat (114). This is because the level of chylomicrons is very dependent on the intake of total fat in the diet.
Familial combined hyperlipidemia includes the phenotype of elevated plasma LDL-C and triglyceride levels. It has a dominant genetic pattern. It is found in 1% of the adult population and in 10% of survivors of premature coronary artery disease. Children with parents who have familial combined hyperlipidemia may have elevated triglycerides and LDL-C (115). However, the levels of LDL-C are usually lower than in children with heterozygous familial hypercholesterolemia. The specific genetic abnormalities for familial combined hyperlipidemia have not been determined, but it is currently thought to result from multiple genes.
Lipoprotein (a) is a protein that is homologous to plasminogen. It consists of an LDL molecule with its Apo B-100 linked with a disulfide bridge to Apo (a). Higher levels of Lp (a) are associated with increased risk of cardiovascular disease in adults (116). Plasma levels of Lp (a) are inversely associated with the molecular weight of the Apo (a) component (117). Isoforms of Lp (a) are genetically determined. This means that levels of Lp (a) in the plasma are also largely inherited. Generally, levels of Lp (a) >30 mg/dL are associated with increased risk of coronary artery disease and stroke. Because of the homology between Lp (a) and plasminogen, there has been a question of whether increased levels of Lp (a) may also be associated with increased thrombosis. A study in children showed increased risk of thrombosis associated with increased Lp (a) (118). High levels of Lp (a) can be expressed in childhood and adolescence (119).
From a clinical perspective, it is quite difficult to lower LP (a). The only effective pharmacologic treatment is with nicotinic acid, which is difficult for most children and adolescents to tolerate. However, because of the increased risk of atherosclerotic cardiovascular disease associated with increased concentrations of LP (a), it is appropriate to use a more aggressive approach to lower LDL-C, when LP (a) concentrations are elevated.
Diet
Another major factor that influences plasma cholesterol is diet. This has been studied more extensively in adults than in children but seems to have similar effects across ages. The major dietary components that affect plasma cholesterol levels are fat and cholesterol. In particular, it is the amount of saturated fat in the diet that is associated with the level of plasma cholesterol. Saturated fat and cholesterol come primarily from animal-based foods. In addition, there are some plant-based oils that are high in saturated fat, such as palm oil.
It is these relationships that ultimately form the basis for nonpharmacologic treatment of cholesterol elevation. In animal models, those fed with increased levels of saturated fat and cholesterol had increased levels of plasma cholesterol and increased risk of atherosclerosis development (120). Furthermore, reduction of dietary fat and cholesterol has been shown to result in lower plasma cholesterol concentrations and resolution of atherosclerotic plaque (121).
TABLE 71.6 Selected Secondary Causes of Dyslipoproteinemia | ||||||||||||||||||
---|---|---|---|---|---|---|---|---|---|---|---|---|---|---|---|---|---|---|
|
Secondary Causes of Cholesterol Elevation
There are several secondary causes of dyslipidemia. Selected causes and their underlying mechanisms are presented in Table 71.6. In addition to those listed, liver disease and obstructive jaundice, infection, and obesity are important secondary causes of abnormal cholesterol levels.
Abnormalities of lipids and lipoproteins are seen in both type 1 and type 2 diabetes. When control of diabetes is poor, triglycerides and LDL-C are often increased and HDL-C is decreased. Improved control of diabetes with intensive management of blood glucose can result in improved plasma lipids and lipoproteins (122,123,124).
Hypothyroidism is associated with increased triglycerides and LDL-C. HDL-C may also be decreased with hypothyroidism. Although it is usually possible to diagnose hypothyroidism by clinical signs and symptoms, occasionally the diagnosis is made because an abnormal fasting lipid profile is found. Appropriate treatment of hypothyroidism results in improvement of abnormal lipids and lipoproteins.
The nephrotic syndrome is a common secondary cause of dyslipidemia in children. Nephrotic syndrome is characterized by proteinuria, hypoalbuminemia, edema, and hypercholesterolemia. Generally, LDL-C is elevated. There may also be hypertriglyceridemia. In this condition, the liver responds to low albumin in the blood by increasing protein synthesis. This includes increased synthesis of the apolipoproteins. This increase in hepatic cholesterol synthesis leads to a downregulation of LDL receptors in the liver, which in turn leads to a reduced rate of removal of LDL-C from the circulation (125). Usually, the length of exposure to increased LDL-C is short because nephrotic syndrome is easily recognized, is treated, and resolves. This may mean that the impact on the risk of atherosclerosis is low; however, the LDL-C level may be quite high prior to treatment (126).
Patients with chronic renal insufficiency are at high risk for cardiovascular disease (127). The dyslipidemia most commonly seen with chronic renal failure is elevation of triglycerides and a low HDL-C. This may be as prevalent as 30% (128). These abnormalities may contribute to the increased risk of cardiovascular disease seen in patients with chronic renal insufficiency. The hypertriglyceridemia of uremia results from deficiency of lipoprotein lipase or hepatic lipase. Treatment of patients with chronic renal insufficiency should include dietary changes to lower intake of saturated fat, cholesterol, and simple sugars. Increased physical activity may also be helpful in increasing HDL-C.
Secondary dyslipidemia can result from taking certain medications. For example, estrogen- and progestin-containing contraceptives can result in elevation of triglycerides. Retinoic acid results in elevation of triglycerides in some patients. Anabolic steroids can cause increased LDL-C and lower HDL-C (129).
Acute and chronic infections can result in dyslipidemia. The causative organism and the chronicity of infection determine which lipid or lipoprotein is altered and to what extent (130). Gidding et al. (131) reported that HDL-C and apolipoprotein A-1 were significantly reduced after a recent viral infection in school-aged children. This means that a lipid profile should generally not be measured within 2 weeks of an acute infection.
Metabolic Syndrome
Obesity has been associated with a cluster of risk factors for cardiovascular disease called the metabolic syndrome (81,132). The metabolic syndrome includes increased waist circumference and atherogenic dyslipidemia that consists of elevated triglycerides and low HDL-C. Patients with the metabolic syndrome often also have insulin resistance. This is characterized by high circulating levels of insulin while fasting. There are many definitions for use in the clinical setting for diagnosis of the metabolic syndrome in children as well as adults. The prevalence of metabolic syndrome appears to be increasing in children and adolescents as the prevalence and severity of obesity is increasing (133).
The primary clinical approach to treating the metabolic syndrome is improvement in weight through changes in diet and physical activity. It has been shown that with weight management, the components of the metabolic syndrome, including atherogenic dyslipidemia, improve (70).
Clinical Recommendations
Guidelines from the NCEP for Children and Adolescents were first published in 1992 (134). These have been updated since that time, as a substantial amount of research has been done in screening and treatment of dyslipidemia in children (90,135). However, the overall framework presented in the original guidelines remains useful. These guidelines present both a population and an individual approach. The population approach addresses the diet and levels of physical activity that would result in a healthy lifestyle for all children. The individual approach addresses the recommended guidelines for the identification and treatment of children and adolescents who are at the highest level of risk. This approach provides a more aggressive strategy for changes in diet than the population approach. The individual approach has been updated by the National Heart Lung and Blood Institute in a new set of evidence-based guidelines (90).
Population Approach
The overall goal of this approach is to improve the health of the pediatric population. If the recommended diet was adopted broadly, this would result in a lower prevalence of obesity and a lowering of the population mean for total and LDL cholesterol. It has been suggested by Rose (136) that a small shift in the population mean of a risk factor will result in substantially fewer individuals in the high-risk range. In addition, if lower levels of LDL-C are maintained from childhood into adolescence and adulthood, then it is likely that this would result in lower risk over time for this childhood population.
Diet
The United States Department Agriculture (USDA) guidelines provide diet recommendations for all children (137,138). The proposed diet for young children is somewhat different because infants and young children require a higher level of saturated fat and cholesterol in their diet to support development of the central nervous system. The first 2 years of life is a time when myelination of nerves is occurring. This process requires increased dietary fat intake. However, for children for whom there is a concern about obesity or an increased risk of cardiovascular disease, it is prudent to consider a lower saturated fat diet starting at 12 months of age. This may start with the introduction of lower fat milk at that time (139,140). This recommendation is supported by the results of the Special Turku Coronary Risk Factors Intervention Project (141). In this randomized
controlled clinical trial, children were randomized to either a lower saturated fat diet or a routine higher fat diet at 6 months of age or at weaning. The participants of this study have been followed into their teenage years. There were no adverse effects of the lower saturated fat diet while there were beneficial effects on blood cholesterol (142). For older children, the USDA recommends a diet low in saturated fat and cholesterol (Table 71.7). There is evidence that this type of diet will lower cholesterol levels in children and adolescents without having an adverse effect on growth and development (143,144).
controlled clinical trial, children were randomized to either a lower saturated fat diet or a routine higher fat diet at 6 months of age or at weaning. The participants of this study have been followed into their teenage years. There were no adverse effects of the lower saturated fat diet while there were beneficial effects on blood cholesterol (142). For older children, the USDA recommends a diet low in saturated fat and cholesterol (Table 71.7). There is evidence that this type of diet will lower cholesterol levels in children and adolescents without having an adverse effect on growth and development (143,144).
TABLE 71.7 Population Dietary Recommendations for Children and Adolescents | ||
---|---|---|
|
The optimum percentages for dietary intake of macronutrients to reduce LDL-C the most and support normal growth and development are not known. It should also be emphasized that the recommendation of 25% to 30% of calories from fat is not necessarily a daily recommendation but should be a recommendation as an average over several days. This recognizes that daily intake may vary substantially for children based on whether they are in school and where and when they eat (135). Children and adolescents should generally not go below a level of fat intake that is 20% of calories. The purpose of this is to avoid over restriction of fat intake by parents, which could lead to failure to grow and thrive (145).
The population-based diet can also be effective for children with borderline elevation of LDL-C. The population diet can result in a lowering of LDL-C by 3% to 10% (146). Usually, it is necessary for patients to be on the diet for 3 to 6 months to adequately assess its effect.
To achieve the dietary recommendations, 5 to 6 oz/d of lean meats and 24 to 32 oz/d of low-fat dairy products are recommended. Lean cuts of meat include ground or lean meats that have had fat trimmed. Skin should be removed from poultry products. All types of fresh or frozen unbreaded fish are acceptable. Choices for dairy include low-fat or nonfat milk, yogurt, and cottage cheese. Cheeses that contain <6 g of fat/oz (<2 g/oz for the more restrictive diet with <7% of calories from saturated fat) are acceptable. Eggs should be limited to <2 to 4 per week (139,140).
The largest portion of the child’s diet should be made up of whole grains, cereals, fruits, and vegetables. Most of these food choices are high in fiber and low in saturated fat and cholesterol.
One concern with cereals and fruit juices is the intake of increased amounts of simple sugars. This may result in increased levels of plasma triglycerides (147). Other obvious sources of simple sugars are soft drinks and snack foods. Lower-fat and lower-sugar options for snacks include pretzels, graham crackers, and vanilla wafer cookies. Fruit should also be recommended as a healthful snack.
Another issue for the diet of children is the food they eat at school. For those who participate in the school lunch program, it is estimated that nearly 60% of children in the United States eat 25% to 30% of their saturated fat and cholesterol intake at that meal (134). However, schools increasingly offer additional competitive food items as part of their school lunch program. These foods are often higher in fat, saturated fat, cholesterol, and sugar and may be more attractive to children, leading to their increased selection and decreased selection of more nutrient-dense foods.
The population approach to cardiovascular health promotion also includes recommendations for physical activity (148,149). Although the optimum level of intensity is not known, increasing the duration and frequency of physical activity and decreasing sedentary time will allow children more flexibility in their diet. For example, the USDA has established recommendations for discretionary calories for children. These are “extra” calories that might be included in the diet once a healthful diet has been consumed and still maintain acceptable energy balance (150,151). The number of discretionary calories increases with age and with the level of physical activity. It is likely that many children are not getting the optimum amount of physical activity and are spending too much time on sedentary pursuits. The American Academy of Pediatrics has recommended no more than 2 h/d be spent on sedentary activities, including television viewing, computer time, and playing video games (145). This means that many children should have only 150 to 300 discretionary calories each day.
One factor that must be considered in implementing the population approach for both diet and physical activity is the role of socioeconomic status (149). Low-income families may have more barriers to purchasing healthful foods. Supermarkets in the inner city may be less convenient and may have lower availability of fresh fruits and vegetables. There are also concerns about neighborhood safety and the lack of opportunities for physical activity including organized sport or unsupervised free-time games.
Implementation of the population-based approach to cholesterol lowering requires the input and cooperation of a number of types of institutions. The government must be involved in improved food labeling and oversight of food assistance programs. Schools must be involved by creating an improved environment for both eating and physical activity. Health professionals should serve as resources for their communities and schools as they develop educational and other risk-reduction programs. In addition, the media can be helpful in the promotion of a healthful diet and increased levels of physical activity.
Individual Approach
The individual approach is directed at identifying children and adolescents who are at higher risk of future cardiovascular disease and treating them to lower their risk. This approach is probably most important from the standpoint of the pediatrician and the pediatric cardiologist. It is this approach that is focused in the physician’s office.
Identification
To initiate the individual high-risk strategy, it is necessary to identify those children who are at higher risk of cardiovascular disease. This approach is directed at identifying children who are likely to have genetic dyslipidemias, who are at highest risk.
In adults, an AHA/ACC risk score has been used to stratify risk (L). The use of this score is dependent on all adults having their risk factors including cholesterol measured on a regular basis. These values can then be used in an equation to estimate risk of a cardiovascular event over the next 10 years. Unfortunately, the data are not
available to use a similar approach for children and adolescents. To construct a similar risk score for children would require large-scale longitudinal studies with complete follow-up in which risk factor levels are measured in childhood and subjects are followed until the occurrence of cardiovascular end points in adulthood. In fact, it is unlikely that such data will be available. This means that a different strategy will be needed to identify children at high risk.
available to use a similar approach for children and adolescents. To construct a similar risk score for children would require large-scale longitudinal studies with complete follow-up in which risk factor levels are measured in childhood and subjects are followed until the occurrence of cardiovascular end points in adulthood. In fact, it is unlikely that such data will be available. This means that a different strategy will be needed to identify children at high risk.
TABLE 71.8 Cutpoints for Total and LDL Cholesterol Levels in Children and Adolescents | |||||||||||||||||||||||||
---|---|---|---|---|---|---|---|---|---|---|---|---|---|---|---|---|---|---|---|---|---|---|---|---|---|
|
The NCEP pediatric panel originally recommended a targeted approach to screening using the family history of cardiovascular disease or cholesterol elevation as the indicator for evaluation (134). However, since publication of the original NCEP guidelines, several studies have been performed to evaluate this approach (150,151,152,153,154). In general, these investigations have found that from 35% to 46% of adolescents would have cholesterol measurements based on their family history. These studies have also shown that many children with elevated cholesterol will be missed using a screening approach based on their family history. It is likely that this approach will miss 30% to 60% of pediatric patients with elevated cholesterol (155,156,157).
Difficulties with using the family history as a trigger for screening include that the family history may be incomplete or inaccurate. The family history would be more useful if all parents and grandparents knew their cholesterol levels, but, unfortunately, this is often not the case. In addition, parents (and sometimes grandparents) of younger children are often too young themselves to have reached the age when they are at greatest risk for a myocardial infarction or a stroke (157).
These problems with a targeted approach to screening have led to the recommendation of universal screening of all children at 9 to 11 years of age (158). In addition, children aged 2 years or older should have a lipid profile if they have a family history of premature cardiovascular disease (prior to age 55 in men or age 65 in women) or of dyslipidemia or with other cardiovascular disease risk factors, such as diabetes, hypertension, or obesity (90).
For universal screening, it is acceptable to use either a fasting lipid profile or a nonfasting non–HDL-C (total cholesterol—HDL-C). If the non–HDL-C is elevated, it should be confirmed with a fasting lipid profile. The focus of universal screening is to identify children with substantial elevation of LDL-C, usually as a result of a genetic dyslipidemia. However, it may also be useful to identify children with elevated triglycerides or a low HDL-C.
The recommended cutpoints for total, LDL, and non-HDL cholesterol are presented in Table 71.8. These cutpoints are used for children and adolescents aged 2 to 18 years. This use across a broad age range is recommended despite the fact that there is considerable variation of cholesterol with age during growth and development. This is especially true during the period of puberty (158,159,160). Total and LDL cholesterol tend to decline during puberty, meaning that some adolescents will appear normal when, in fact, they will have elevated levels after puberty. However, it has been shown that the proposed single cutpoints work well in practice (161).
The NHLBI has recommended that triglycerides >150 mg/dL and HDL-C <40 mg/dL be considered abnormal for children and adolescents (90).
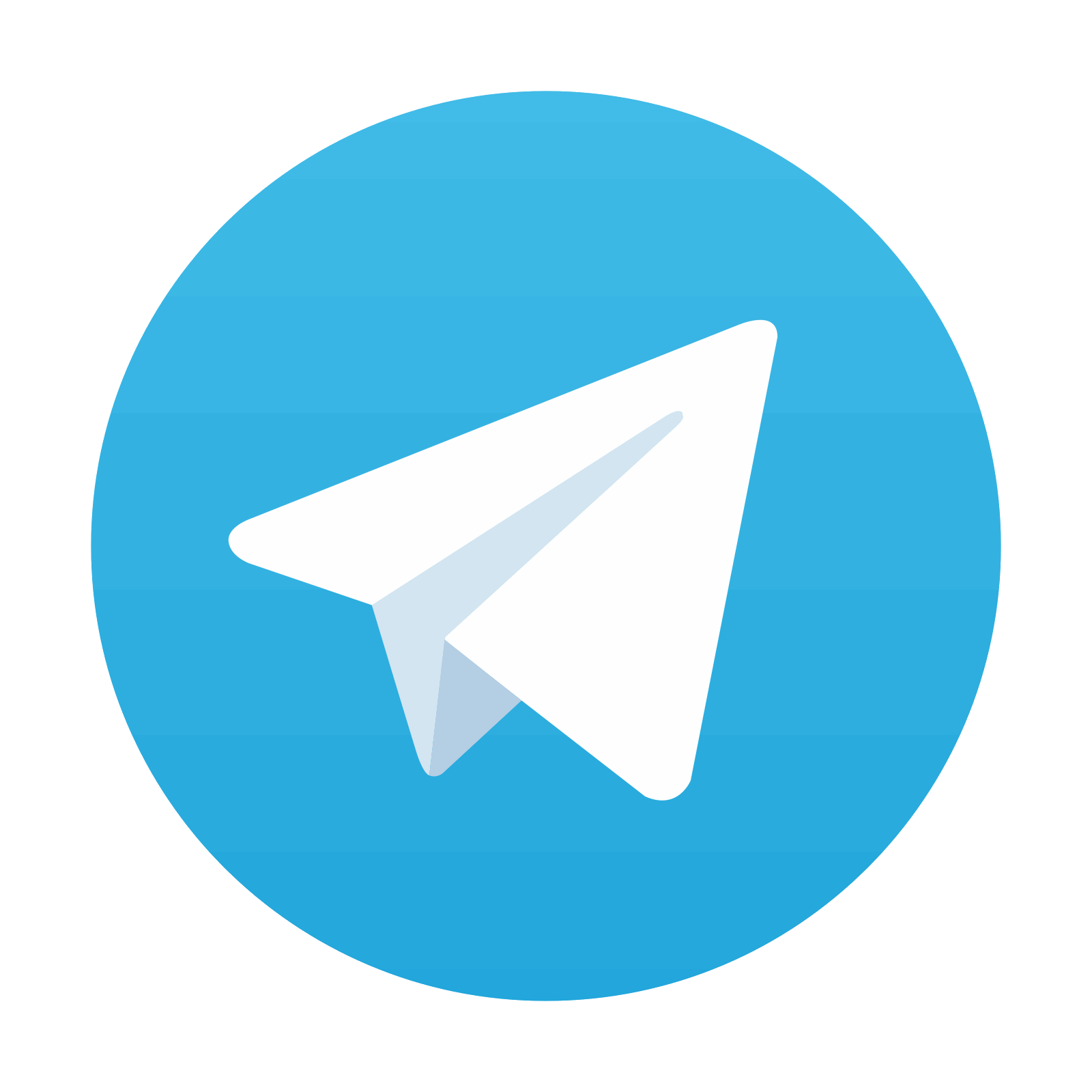
Stay updated, free articles. Join our Telegram channel

Full access? Get Clinical Tree
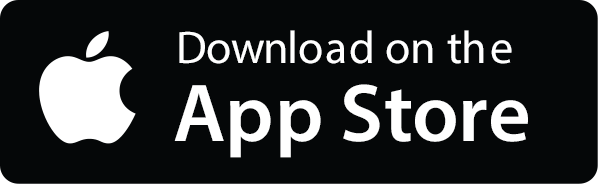
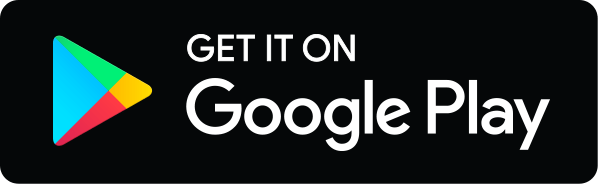
