Background
The purpose of this study was to evaluate the potential of combined use of transthoracic Doppler echocardiographic coronary flow velocity reserve (CFVR) measurements and findings of retrograde coronary flow in the three main coronary arteries for the assessment of borderline (angiographic diameter stenosis, 50%–75%) and high-grade (angiographic diameter stenosis, 76%–100%) coronary artery stenoses.
Methods
A total of 108 patients scheduled for coronary angiography because of chest pain or acute coronary syndromes were studied. CFVR was measured during adenosine-induced hyperemia in the mid to distal segments of the left anterior descending coronary artery, the marginal branches of the left circumflex coronary artery, and the posterior descending coronary artery, with peak CFVR (pCFVR) <2.0 implying hemodynamic significant stenosis. CFVR results were compared with results from quantitative coronary angiography, with stenosis severity in the left main and three major coronary arteries divided into three groups: (1) diameter stenosis 0% to 49%, (2) diameter stenosis 50% to 75%, and (3) diameter stenosis 76% to 100%.
Results
In patients with antegrade flow in the relevant coronary artery segment, CFVR was successfully measured in the mid to distal left anterior descending artery, the marginal branches of the left circumflex artery, and the posterior descending artery in 97%, 63%, and 75% of patients, respectively. CFVR was significantly different among the stenosis groups, with pCFVR of 2.79 ± 0.77 in group 1, 2.01 ± 0.72 in group 2, and 1.50 ± 0.69 in group 3 ( P < .001 among groups). Angiography confirmed retrograde flow in seven of the nine arteries found by transthoracic echocardiography. Findings of pCFVR <2.0 or retrograde coronary artery flow correctly identified 42 of 49 patients with stenoses in group 3, with sensitivity, specificity, and positive and negative predictive values of 86%, 70%, 70%, and 85%, respectively. In group 2, pCFVR values were widely distributed above or below the defined pCFVR cutoff value.
Conclusions
CFVR measurement in the mid to distal left anterior descending artery was feasible in almost all patients, and in the marginal branches of the left circumflex artery and the posterior descending artery in two thirds and three quarters of patients, respectively. Use of the combined echocardiographic criteria had high precision for diagnosing severe coronary stenoses (diameter stenosis, 76%–100%). The functional significance of angiographically borderline stenoses (diameter stenosis, 50%–75%) may be further differentiated by the use of CFVR measurements. ( ClinicalTrials.gov NCT00281346)
Quantitative coronary angiography (QCA) is regarded as the gold standard for the evaluation of coronary artery disease, with a significant coronary stenosis generally defined as luminal diameter reduction ≥50%. Anatomic evaluation of the degree of stenosis does not always reflect the functional significance of a stenosis, especially in borderline stenoses (diameter stenosis in the range of 40%–75%). Recent guidelines recommend functional testing for patients with coronary disease with angiographic borderline coronary stenoses of uncertain functional significance. Findings during cardiac catheterization of a damped poststenotic coronary flow reserve (CFR) (the ratio of hyperemic blood flow to resting blood flow) of <2.0 or a fractional flow reserve of <0.75 to 0.80 imply that a coronary stenosis is hemodynamic significant. However, these examinations are expensive, invasive, and available only during cardiac catheterization. Several studies have recently documented strong correlations between invasively measured CFR and coronary flow velocity reserve (CFVR) (the ratio of hyperemic blood flow velocity to resting blood flow velocity) measured by transthoracic coronary Doppler echocardiography (TTE), documented for the left anterior descending coronary artery (LAD), the posterior descending coronary artery (PDA), and the left circumflex coronary artery (Cx). A CFVR value <2.0 measured by TTE during adenosine infusion has been shown to indicate one or more hemodynamic significant stenoses located more proximally in the coronary artery, documented for all three major coronary arteries. Coronary occlusion may be detected by seeing retrograde flow in the coronary artery during TTE. Most studies, however, have evaluated CFVR (measured by TTE) only in single coronary arteries in limited patient cohorts and have not included transthoracic echocardiographic findings of retrograde coronary artery flow. Furthermore, there is a paucity of studies comparing CFVR obtained by TTE with various degrees of coronary obstruction as defined by QCA.
The aim of this study was to assess using TTE the feasibility and accuracy of diagnosing high-grade stenoses and occlusions in all three main coronary arteries using CFVR or findings of retrograde coronary flow during the process of visualizing the individual coronary segment for CFVR measurements, using QCA as the gold standard.
Methods
Study Population
Patients were included in the study if they fulfilled the following criteria: (1) already scheduled for coronary angiography because of documented or suspected stable or unstable coronary disease, (2) age >18 years, and (3) met no exclusion criteria. The exclusion criteria were (1) previous aortocoronary bypass surgery, (2) presumed insufficient acoustic windows because of severe emphysema or severe overweight, (3) significant valvular disease, (4) atrial fibrillation, (5) contraindications to adenosine administration, and (6) administrative reasons.
The study protocol was approved by the Regional Committee for Medical and Health Research Ethics and the Norwegian Data Inspectorate. All participants gave written, informed consent. (This study is registered at ClinicalTrials.gov under identifier NCT00281346.)
Six patients did not enter the study, because of insufficient acoustic windows ( n = 3), lack of consent ( n = 2), or aortic stenosis ( n = 1). We included 115 patients in the study, but 7 patients were later excluded from further analysis because of protocol violations: aortic stenosis ( n = 2), atrial fibrillation ( n = 2), patient refusal of coronary angiography ( n = 2), and no indication for coronary angiography ( n = 1). The final study group consisted of 108 patients. Clinical characteristics of the patients are presented in Table 1 . All patients took their medications on the day of the echocardiographic study.
Variable | Value |
---|---|
Age (y) | 63.1 ± 9.5 |
Heart rate (beats/min) | 63 ± 7.4 |
BMI (kg/m 2 ) | 26 ± 3.6 |
Men | 79 (73%) |
Total cholesterol (mmol/L) | 4.9 ± 1.1 |
Blood pressure (mm Hg) | |
Systolic | 142 ± 20 |
Diastolic | 82 ± 12 |
Medical history | |
Hypertension (>140/90 mm Hg) | 61 (55%) |
Current smoking | 29 (27%) |
Diabetes | 11 (10%) |
Previous CAD | 23 (21%) |
ACS | 35 (32%) |
Cardiac medications | |
Aspirin | 97 (90%) |
Thienopyridines | 38 (35%) |
Low–molecular weight heparin | 30 (28%) |
β-blockers | 85 (79%) |
Statins | 87 (81%) |
Calcium antagonists | 21 (19%) |
ACE inhibitors/ARBs | 24 (22%) |
Organic nitrate, daily maintenance | 13 (12%) |
Transthoracic Coronary Flow Measurements
Patients were examined using an Acuson Sequoia C512 (Siemens Medical Solutions USA, Inc., Mountain View, CA) ultrasound system connected to standard 4V1C and 7V3C transthoracic transducers. Contrast agent was not used. CFVR measurements in unstable patients could not be performed earlier than the day after hospital admission, and these patients had to be stable without ongoing angina, ischemia, or need for intravenous nitroglycerin. The coronary arteries were investigated using color Doppler mapping with data postprocessing mix function, which makes the colors transparent. The velocity scale of color Doppler was set to 0.24 m/sec but was actively changed to provide optimal images. The methodology of visualization of the mid to distal LAD and PDA was described in detail previously. With the patient in the left lateral decubitus position, the mid and distal LAD could be seen from modified parasternal short-axis and long-axis views or from modified apical two-chamber and three-chamber views, focusing on the anterior interventricular sulcus ( Figure 1 A1). From modified apical two-chamber views focusing on the posterior interventricular sulcus, the PDA could be seen coursing toward the apex ( Figure 1 C1). From modified apical four-chamber views focusing on different levels of the lateral wall of the left ventricle, the marginal branches of Cx (CxMb) could be visualized coursing in distal direction on the epicardial surface toward the transducer ( Figure 1 B1). Whenever possible, the most inferior marginal branch viewed was used for measurements.

The coronary artery flow velocity waveform appears as a complex of a small wave in systole and a large trapezoid wave in diastole ( Figures 1 A2, 1 B2, and 1 C2). When color flow Doppler recordings indicated reversed coronary artery flow (the functional diagnosis of coronary obstruction more proximal in the coronary artery) in the mid to distal LAD, CxMb, or PDA, we distinguished the retrograde coronary artery flow from coronary venous flow by finding inverted coronary flow velocity waveform ( Figure 2 ). In contrast, the coronary venous flow appears as a prominent systolic flow wave. If retrograde coronary artery flow was found in the mid to distal LAD, CxMb, or PDA, we concluded that the artery was occluded more proximally, and CFVR measurements were not performed.

When the mid to distal LAD, CxMb, or PDA was found with antegrade flow, the CFVR of the individual arterial segment was investigated. Blood flow velocities were measured using pulsed-wave Doppler with 1.75-MHz to 3.5-MHz frequencies in a sample volume of 1.5 to 5 mm, with the sample volume positioned on the laminar color flow Doppler signal. The sample volume was positioned distally to any visualized turbulent color flow Doppler signal, because turbulent color flow Doppler signals might represent stenosis. Adenosine was administered by intravenous infusion (0.14 mg/kg/min over 2 min) to record spectral Doppler signals during hyperemia ( Figures 1 A3, 1 B3, and 1 C3). Peak and mean diastolic flow velocities were measured, and CFVR was calculated as the ratio of hyperemic to basal peak CFVR (pCFVR) and mean CFVR flow velocities. Angle correction was used during velocity measurements to keep the angle between blood flow and Doppler beam as small as possible, but the angle was not altered between baseline and adenosine infusion measurements. We tried to find at least three consecutive cardiac cycles to average flow velocities, both at baseline and during adenosine infusions. With suboptimal quality of Doppler registrations, the CFVR procedure was repeated once or twice, and the best series were used for measurements. A predefined cutoff value of <2.0 for pCFVR or mean CFVR was used for significant stenosis, in accordance with earlier studies.
For each segment (mid to distal LAD, CxMb, and PDA), four different outcomes were defined: (1) the coronary segment was defined with retrograde flow, (2) the coronary segment was not visualized, (3) the coronary artery was defined with antegrade flow with CVFR impossible to measure, and (4) CVFR was satisfactorily measured. Stop frames and clips were digitally recorded for offline analysis.
Coronary Angiography
Coronary angiography was performed using standard techniques. All angiographic studies were digitally stored with later offline reviewing and measurements, blinded to the findings by TTE. Disagreements in interpretation were resolved by consensus of the two cardiologists responsible for the angiographic readings. All angiograms were classified according to left or right dominance. The severity of coronary stenoses in the left main coronary artery (LM) and three major coronary arteries was determined by QCA. The angiograms were analyzed using a 16-segment model of the coronary arteries. Each coronary artery segment was categorized in one of the following three groups: (1) diameter stenosis 0% to 49%, (2) diameter stenosis 50% to 75%, and (3) diameter stenosis 76% to 100%. Collateral flow to occluded arteries was graded according to the Rentrop classification (grade 0 = no visible filling of any collateral channel, grade 1 = filling of side branches of the occluded artery, grade 2 = partial filling of the epicardial vessel, grade 3 = complete collateral filling of the epicardial vessel). However, because the purpose of the study was to evaluate the accuracy of diagnosing stenoses and occlusions in the main coronary arteries by TTE compared with coronary angiography, the presence of one-vessel, two-vessel, or three-vessel disease was defined by findings of diameter stenoses ≥50% in the main course of the coronary arteries. Diameter stenosis ≥50% in the LM was defined as two-vessel disease unless in case of left dominance, for which it was defined as three-vessel disease.
Reproducibility
To assess interobserver measurement variability, two experienced observers (J.V. and E.H.) evaluated data from 12 random cases in a blinded manner. Intraobserver variability was similarly tested by one experienced observer (J.V.) 2 weeks apart, blinded to previous results. For the reproducibility studies, four separate coronary flow velocity waveform recordings were selected for measurements in each patient. Both interobserver and intraobserver measurement variability were expressed as the mean difference in percentage and the coefficient of variation of the differences between the measurements for each parameter.
Statistical Analysis
Continuous variables are presented as mean ± SD. Categorical variables are presented as fractions and percentages. Comparisons of mean values were performed using Student’s t tests for normally distributed parameters. One-way analysis of variance was used to analyze data when assessing intergroup differences. Logistic regression analyses were used to explore relationships between success rate for CFVR measurements and demographic and clinical variables. Linear regression analyses were used to explore the relationship between CFVR and baseline characteristics. Variables with P values < .10 in univariate testing were entered in multivariate forward analyses. Sensitivity, specificity, and positive and negative predictive values to detect diameter stenoses ≥50% or ≥76% in the presence of CFVR <2.0 were assessed using standard formulas. P values < .05 were considered statistically significant. All analyses were performed using SPSS for Windows version 15.0 (SPSS, Inc., Chicago, IL).
Results
QCA
There were 35 patients with and 73 patients without unstable coronary disease ( Table 1 ). For stable patients, the mean time from echocardiographic examination to angiography was 24.7 ± 31.7 days. In the group with unstable coronary disease, the mean time from echocardiographic examination to coronary angiography was 9.5 ± 23.1 days. However, 2 patients in this group had longer delays for coronary angiography because of intercurrent disease, and the mean time from echocardiographic examination to angiography was 4.3 ± 3.4 days for the remaining 33 patients.
Table 2 lists quantitative coronary angiographic findings of stenoses in the main coronary arteries (segments 1–8, 11, 13, and 15 in the American Heart Association’s 16-segment model ) in groups 2 and 3. Eight patients were found to have dominant Cx, supplying the PDA from the distal Cx, and in one patient, co-dominance was demonstrated by angiography. Among the patients with left dominance, only a distal stenosis in group 2 was found in the Cx. On the basis of findings in the main course of the coronary arteries, one-vessel, two-vessel, and three-vessel disease occurred in 38, 21, and 14 patients, respectively. Additionally, coronary angiography revealed at least one significant stenosis in diagonal and/or marginal branches in 53 of these patients. Seven patients had at least one significant stenosis in diagonal and/or marginal branches, without stenoses in the main arteries. These stenoses were located in small branches with vessel diameters <2 mm.
Segment | Stenosis group 2 (DS, 50%–75%) | Stenosis group 3 (DS, 76%–100%) |
---|---|---|
LM | 4 | 0 |
pLAD | 21 | 12 |
mLAD | 15 | 5 |
dLAD | 4 | 2 |
pCx | 6 | 4 |
mCx | 5 | 5 |
dCx | 3 | 2 |
pRCA | 14 | 13 |
mdRCA | 14 | 18 |
PDA | 4 | 3 |
Feasibility of CFVR Measurements
There were no serious adverse effects during adenosine infusion. For the patient group with unstable coronary disease, the mean time from symptom start of acute coronary syndromes (ACS) to CFVR measurements was 3.9 ± 3.0 days. Figure 3 presents the artery-based study protocol, with results. According to protocol, CFVR was not investigated if the coronary artery segment used for CFVR measurements showed retrograde flow by TTE, because this was interpreted as more proximal occlusion of the artery. Retrograde flow was identified by TTE in the mid to distal LAD in 2 patients, while 1 patient was found with retrograde flow in the CxMb ( Figure 2 ). In the PDA, retrograde flow was identified in 5 patients, and another patient was found (while investigating for PDA flow) with retrograde flow in the right coronary artery (RCA) just proximal to the PDA. Coronary angiography confirmed the findings of retrograde flow in all arteries except two PDAs found angiographically with antegrade flow. The seven arteries correctly identified with retrograde flow were all proximally occluded, with Rentrop grade ≥2 collateral circulation. The mid to distal LAD, CxMb, and PDA could not be visualized in 1, 35, and 22 patients, respectively, while the same artery segments could be seen but without satisfactory CFVR measurements in 2, 5, and 3 patients, respectively. CFVR was satisfactorily measured in the mid to distal LAD, CxMb, and PDA in 103 (97%), 67 (63%), and 77 (75%) patients, respectively, with the numbers in parentheses denoting the percentages of patients with CFVR measurements among patients not found with retrograde flow in the relevant coronary artery segment. CFVR was satisfactorily measured in all three coronary territories in 50 patients (46%). Analyses showed no statistical differences in the degree of feasibility of CFVR measurements when adjusted for baseline characteristics of the study cohort, left or right dominance, or clinical presentation, with the exception of reduced feasibility of CFVR measurements in the LAD in patients with ACS ( P = .01).

CFVR Compared to Angiography
At QCA, each main coronary artery could have more than one stenosis, with the most tight stenosis defining the degree of stenosis. CFVR measurements in mid to distal LAD evaluated both the LAD and LM because of the functional unity of LAD and LM, and findings of LAD and LM were therefore analyzed together. Distal branches from the Cx were more difficult to visualize by TTE than the more proximal branches, implying that the CxMb CVFR measurements primarily evaluated stenoses in the proximal and mid segments of the Cx and less often stenoses in the distal segment of the Cx. Hence, stenoses in the distal segment of the Cx (segment 15 in the American Heart Association’s 16-segment model ) were excluded from CFVR CxMb analyses, with the proximal and mid segments of the Cx and the LM analyzed together because of functional unity. Any angiographic stenosis in hypoplastic right coronary arteries was excluded from the analysis. Four of the 77 patients with CFVR measurements in the PDA had left dominance, all with normal PDA CFVR measurements (pCFVR ≥ 2.0) and without angiographically significant stenoses in proximal segments (LM, Cx, and PDA). Among coronary arteries with CFVR measurements, 48 arteries (28 LADs and LMs, 5 proximal and mid segments of the Cx and LMs, and 15 PDAs) had stenoses in group 2, and 37 arteries (15 LADs and LMs, 3 proximal and mid segments of the Cx and LMs, and 19 PDAs) had stenoses in group 3. During adenosine-induced hyperemia, the mean heart rate increased from 63 ± 7.4 to 68 ± 10.4 beats/min. Peak and mean diastolic coronary flow velocities at baseline and during hyperemia for the different stenosis groups are listed in Table 3 , with corresponding CFVR measurements. Two models were examined to further evaluate peak and mean CFVR: an ordered logistic model with diameter stenosis 50% to 100% as the response variable with peak and mean CFVR as continuous covariates and an ordered model with diameter stenosis 50% to 100% as the response variable with peak and mean CFVR as dichotomous covariates. In both models, pCFVR was highly significant ( P < .001 in both), while mean CFVR was not significant ( P = .78 and P = .53). Therefore, pCFVR was used for further analyses. Peak CFVR was significantly different among the stenosis groups, with pCFVR of 2.79 ± 0.77 in group 1, 2.01 ± 0.72 in group 2, and 1.50 ± 0.69 in group 3 ( P < .001 among groups). Within each stenosis group, no statistical differences were found between pCFVR measurements in the different arteries. Peak CFVR measurements were not related to baseline characteristics of the study cohort, left or right dominance, or clinical presentation, with the exception of inverse correlation between age and pCFVR for the LAD ( P = .008). Figure 4 shows the spread of pCFVR measurements for the different stenosis groups. Most pCFVR measurements in group 1 were above the cutoff value of 2.0, while most of pCFVR measurements in group 3 were below the cutoff value. However, group 2 was more heterogenous, with 26 pCFVR measurements below and 22 measurements above 2.0 ( Figures 4 and 5 ). The negative predictive value of pCFVR ≥2.0 in stenosis group 1 was 65%, while the positive predictive value of pCFVR <2.0 in stenosis group 3 was 70%. The sensitivity, specificity, and positive and negative predictive values of pCFVR <2.0 for the detection of diameter stenosis ≥50% were 76%, 86%, 92%, and 65%, respectively. There was no statistically significant difference in the distribution of the various risk factors in the different stenosis groups or in patients with pCFVR above or below 2.0 within each stenosis group.
PDV (m/sec) | MDV (m/sec) | Delta | CFVR | Delta CFVR | |||||
---|---|---|---|---|---|---|---|---|---|
Baseline | Hyperemia | Baseline | Hyperemia | Peak | Mean | Peak | Mean | ||
Group 1 (DS, 0%–49%) | |||||||||
mdLAD ( n = 60) | 0.31 ± 0.10 | 0.89 ± 0.29 | 0.23 ± 0.08 | 0.65 ± 0.21 | 0.57 ± 0.18 ∗ | 0.42 ± 0.14 ∗ | 2.79 ± 0.77 | 2.83 ± 0.83 | −0.04 ± 0.07 † |
CxMb ( n = 59) | 0.34 ± 0.08 | 0.89 ± 0.18 | 0.25 ± 0.06 | 0.67 ± 0.15 | 0.56 ± 0.10 ∗ | 0.42 ± 0.09 ∗ | |||
PDA ( n = 43) | 0.28 ± 0.08 | 0.70 ± 0.20 | 0.21 ± 0.06 | 0.53 ± 0.16 | 0.42 ± 0.12 ∗ | 0.32 ± 0.10 ∗ | |||
Group 2 (DS, 50%–75%) | |||||||||
mdLAD ( n = 28) | 0.35 ± 0.10 | 0.69 ± 0.28 | 0.25 ± 0.08 | 0.51 ± 0.22 | 0.35 ± 0.18 ∗ | 0.26 ± 0.15 ∗ | 2.01 ± 0.72 | 2.01 ± 0.74 | 0.01 ± 0.04 † |
CxMb ( n = 5) | 0.39 ± 0.12 | 0.60 ± 0.21 | 0.27 ± 0.07 | 0.47 ± 0.19 | 0.21 ± 0.09 † | 0.18 ± 0.12 † | |||
PDA ( n = 15) | 0.37 ± 0.11 | 0.70 ± 0.16 | 0.27 ± 0.08 | 0.50 ± 0.14 | 0.33 ± 0.05 ∗ | 0.23 ± 0.06 ∗ | |||
Group 3 (DS, 76%–100%) | |||||||||
mdLAD ( n = 15) | 0.32 ± 0.12 | 0.52 ± 0.34 | 0.26 ± 0.09 | 0.42 ± 0.27 | 0.20 ± 0.23 ∗ | 0.16 ± 0.18 ( P = .003) | 1.50 ± 0.69 | 1.51 ± 0.78 | −0.01 ± 0.09 † |
CxMb ( n = 3) | 0.45 ± 0.10 | 0.62 ± 0.35 | 0.33 ± 0.11 | 0.46 ± 0.32 | 0.17 ± 0.25 † | 0.13 ± 0.21 † | |||
PDA ( n = 19) | 0.37 ± 0.13 | 0.52 ± 0.26 | 0.31 ± 0.12 | 0.36 ± 0.21 | 0.15 ± 0.13 ( P = .003) | 0.06 ± 0.09 † |
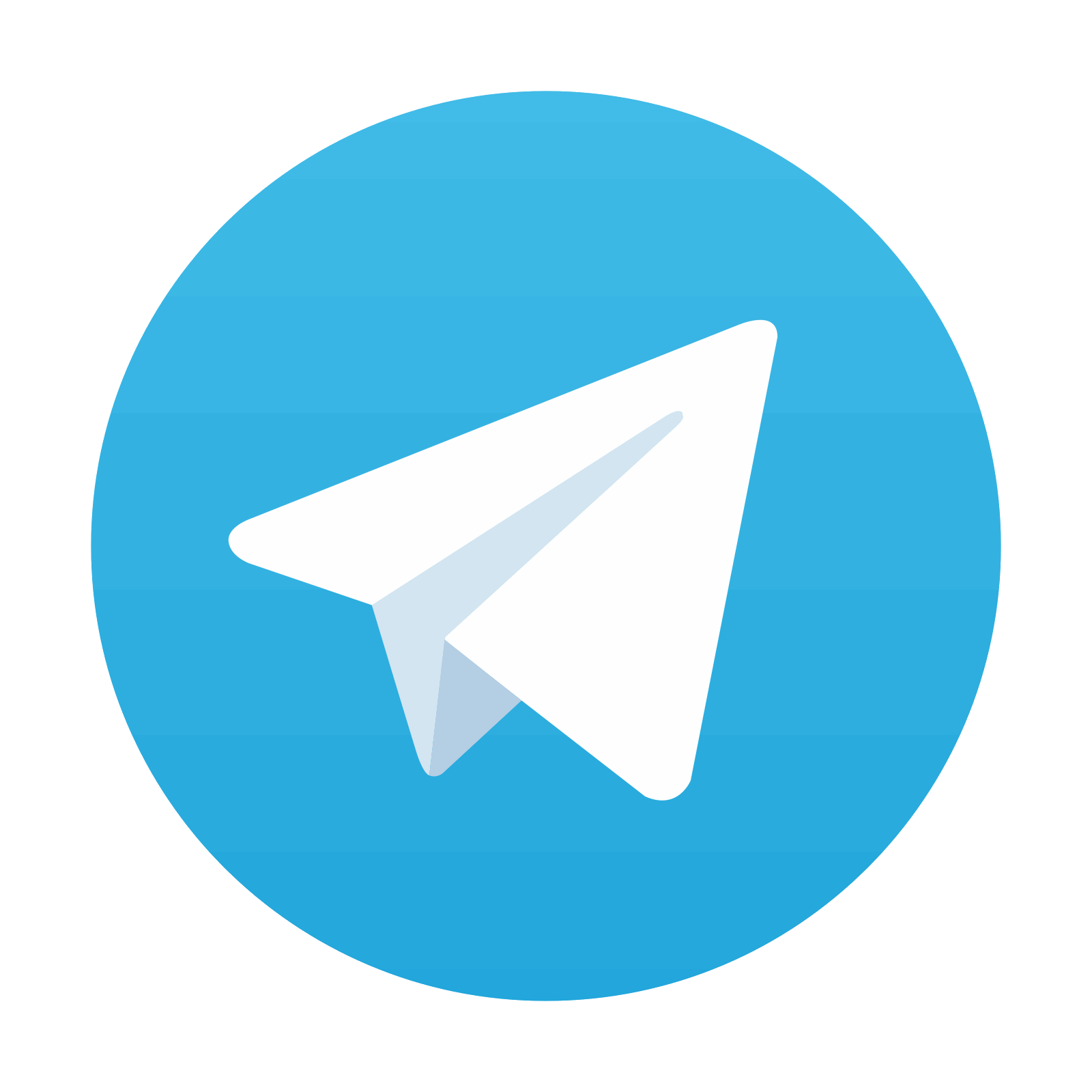
Stay updated, free articles. Join our Telegram channel

Full access? Get Clinical Tree
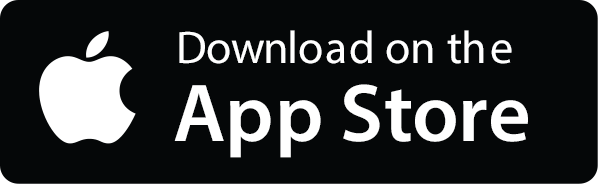
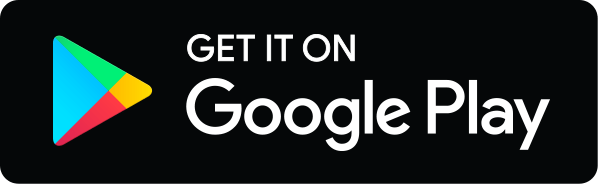
