Noninvasive identification of nonculprit lesions could improve preventive strategies for acute myocardial infarction (AMI). We assessed the morphology, composition, and spatial distribution of nonculprit coronary plaques in patients with AMI using computed tomographic angiography (CTA). A total of 64 patients with AMI underwent 64-slice CTA within 2 weeks after admission, and 162 symptomatic patients with stable angina pectoris (SAP) underwent CTA and stress myocardial perfusion imaging (MPI). Of these 226 patients, 16 were excluded from the analysis because of image artifacts. The mean number of nonculprit plaques per patient was 5.0 ± 2.6 in the AMI group (n = 60), 4.2 ± 2.6 in the SAP group with abnormal MPI findings (n = 67), and 1.1 ± 1.3 in the SAP group with normal MPI findings (n = 83; p <0.01). Positive remodeling and low-attenuation plaques (<30 Hounsfield units) were more frequently observed in the AMI group (1.9 ± 1.8) than in the SAP groups (0.6 ± 0.9 with abnormal MPI findings and 0.2 ± 0.4 with normal MPI findings; p <0.01). Within the AMI group, positive remodeling and low-attenuation plaques were present significantly more frequently in patients with metabolic syndrome than in those without (2.6 ± 2.2 vs 1.4 ± 1.4; p = 0.03) and was significantly more frequently distributed in the proximal segments of the left anterior descending artery (p <0.01). In conclusion, 64-slice CTA could provide promising information for preventive strategies by identifying nonculprit plaque morphology and zones at high risk of future events.
The recent advent of multidetector computed tomographic angiography (CTA) has greatly improved image quality and might allow more precise evaluation of coronary stenosis and plaque morphology. Previous studies of CTA introduced the concept of noninvasive detection and characterization of coronary atherosclerotic lesions in patients with acute myocardial infarction (AMI) and stable angina pectoris (SAP) and identified differences in lesion morphology and plaque composition between culprit lesions in patients with acute coronary syndrome and stable lesions in patients with acute coronary syndrome or SAP. Recently, Motoyama et al demonstrated that the computed tomographic characteristics of the culprit lesions in acute coronary syndrome included positive remodeling and low-attenuation plaques (LAPs) and that patients with these 2 plaque characteristics found using CTA were at a greater risk of acute coronary syndrome developing during follow-up compared to patients having lesions without these characteristics. We therefore hypothesized that positively remodeled plaques with LAPs in nonculprit lesions, favoring a propensity to rapidly progress to a culprit lesion or rupture, might be more frequently observed in patients with AMI within the proximal 1/3 of the major epicardial coronary arteries. To investigate this hypothesis, we examined whether 64-slice CTA can be used to noninvasively assess the spatial distribution, morphology, and composition of nonculprit lesions in patients with AMI compared to stable lesions in patients with SAP.
Methods
We studied a prospective, but nonconsecutive, series of 226 patients who had undergone 64-slice CTA from September 2006 to December 2007. The study population consisted of 64 patients with AMI and 162 symptomatic patients with suspected coronary artery disease who underwent stress myocardial perfusion imaging (MPI). In these 162 patients, the stress MPI findings were positive in 72 patients and negative in 90 patients. AMI was defined as follows: (1) chest pain >30 minutes in duration with presentation within 12 hours after the onset of symptoms; (2) ST-segment elevation >0.1 mV within 2 contiguous electrocardiograph leads; and (3) elevated creatine kinase-MB isoenzymes within 12 hours of chest pain. The patients with AMI underwent 64-slice CTA within 2 weeks after successful primary stenting. SAP was defined as no change in frequency, duration, or intensity of chest pain within 4 weeks in patients in sinus heart rhythm and without previous myocardial infarction, previous percutaneous coronary intervention or coronary bypass surgery, and unstable angina.
Of the 226 patients, 16 (4 in the AMI group, 5 in the SAP group with abnormal MPI findings, and 7 in the SAP group with normal MPI findings) were excluded from the present analysis because of image artifacts that made assessment of the entire coronary anatomy impossible on the computed tomographic angiographic images. The remaining 210 patients (AMI group, n = 60; SAP with abnormal MPI group, n = 67; and SAP with normal MPI group, n = 83) represent the population of the present study. All patients provided written informed consent, and our institutional review board approved the study protocol.
Scanning was performed using a 64-slice computed tomographic scanner (Aquilion 64; Toshiba Medical Systems, Otawara, Japan). According to a previous published protocol, the images were acquired with 64 × 0.5-mm slice collimation, gantry rotation speed of 400 ms/rotation, table feed of 6.4 mm/gantry rotation, tube energy of 120 kV, and an effective tube current of 400 mA. The computed tomographic dose index volume and dose–length product of this scan protocol was 75.2 mGy and 1.10 Gy × cm, respectively, and corresponded to an approximate mean radiation dose of 15 mSv. A 60-ml volume of contrast agent (iopamidol 370, 370 mgI/ml, Schering AG, Berlin, Germany) was injected intravenously at a rate of 4 ml/s. The patients were given oral metoprolol 20 to 40 mg 1 hour before the scheduled scan if their heart rate was >65 beats/min, and all patients were given sublingual nitroglycerin 0.3 mg 5 minutes before the scan.
The analysis of the scans was performed using a ZIOSTATION workstation (ZIOSOFT, Tokyo, Japan). The images were initially reconstructed at 75% of the cardiac cycle, with a slice thickness of 0.5 mm at an increment of 0.3 mm. In the case of motion artifacts, additional reconstructions were made at different points of the RR interval. Each scan was analyzed independently by 2 experienced readers who were unaware of the results of the stress thallium-201 single photon emission computed tomography.
The image display settings were modified on an individual basis to accommodate differences in contrast enhancement or image noise in some patients. The individually optimized window width (600 to 900 Hounsfield units) and level (100 and 250 Hounsfield units) settings were maintained for each of the measurements. Coronary atherosclerotic plaques were visually classified as follows. Noncalcified plaque was defined as any discernible structure that could be assigned to the coronary artery wall, with computed tomographic attenuation less than that of the contrast-enhanced coronary lumen but greater than that of the surrounding connective tissue/epicardial fat. Calcified plaque was defined as any structure with computed tomographic attenuation of >130 Hounsfield units that could be visualized separately from the contrast-enhanced coronary lumen (either because it was embedded within noncalcified plaques or because its density was greater than that of the contrast-enhanced lumen). Spotty calcification was defined when <3 mm in size on curved multiplanar reformation and one-sided on cross-sectional images. Mixed plaque was defined as plaque with noncalcified and calcified elements present. LAP was defined as plaque with low computed tomographic density (<30 Hounsfield units). The remodeling index was calculated by dividing the lesion diameter at the plaque site of maximum luminal narrowing by the reference diameter (proximal to the lesion in a normal-appearing vessel segment). Positive remodeling was defined as a remodeling index of >1.10. We used the coronary map modified from the Bypass Angioplasty Revascularization Investigation (BARI). Proximal segments of coronary plaque location included 1, proximal right coronary artery; 12, proximal left anterior descending artery; and 18, proximal left circumflex artery. Middle segments included 2, mid-right coronary artery; 13, mid-left anterior descending artery; 15, diagonal 1 branches; 19, mid-left circumflex artery; and 20, obtuse marginal 1. Distal segments included 3, distal right coronary artery; 4, posterior descending artery; 6, posterior lateral segment; 14, distal left anterior descending artery; 16, diagonal 2 branches; 19a, distal left circumflex coronary artery; and 21, obtuse marginal 2.
Thallium-201 single photon emission computed tomography was performed within 1 week of the CTA. β Blockers, calcium channel blockers, and nitrates were discontinued for 24 to 48 hours before the test. Adenosine was administered intravenously to all patients at 0.14 mg/kg/min for 6 minutes, and a dose of 111 MBq of thallium-201 was injected 3 minutes into the infusion. The electrocardiogram and blood pressure were monitored before, throughout, and after the infusion. The initial image was obtained in the supine position at 5 minutes after the thallium-201 injection, and a delayed image was obtained 4 hours later.
Single photon emission computed tomography was performed using a double-detector system (Picker Prism 2000 XP, Shimadzu, Kyoto, Japan) equipped with a low-energy, high-resolution collimator according to a previous published protocol. The single photon emission computed tomographic images were assessed using a 17-segment model. Stress and delayed images were independently analyzed by 2 nuclear medicine physicians who were unaware of the data from CTA. Visual grading was defined as normal (no perfusion defects) or abnormal (stress perfusion defects with redistribution).
Hypertension was defined as blood pressure at rest ≥140/90 mm Hg or the use of antihypertensive medication. Hyperlipidemia was defined as a total fasting serum cholesterol level of ≥200 mg/dl or serum triglycerides of ≥150 mg/dl or use of antihyperlipidemic medication. The revised National Cholesterol Education Program criteria have defined metabolic syndrome as the presence of any 3 of the following 5 traits: (1) the cutpoint of waist circumference was modified for the Japanese as ≥90 cm in men and ≥80 cm in women, according to the recommendation by the International Diabetes Federation; (2) blood pressure ≥130/85 mm Hg or drug treatment for elevated blood pressure; (3) serum triglycerides of ≥150 mg/dl or drug treatment for elevated triglycerides; (4) high-density lipoprotein cholesterol <40 mg/dl in men and <50 mg/dl in women or drug treatment for low high-density lipoprotein cholesterol; and (5) fasting glucose of ≥100 mg/dl or drug treatment for elevated blood glucose levels.
All data are expressed as the mean ± SD or the median and interquartile range. Comparisons of categorical variables between groups were performed using the chi-square test or Fisher’s exact test. Comparisons of continuous variables were analyzed using one-way analysis of variance or the Kruskal-Wallis test. All probability values were considered significant at p <0.05.
Results
The baseline clinical characteristics of the patients are summarized in Table 1 , and the characteristics of the nonculprit lesions in AMI are presented in Figure 1 . Of the 300 plaques identified in the AMI group, 153 plaques (51%) were classified as noncalcified, 72 (24%) as mixed, and 75 (25%) as calcified. Of the 281 plaques identified in the SAP group with abnormal MPI findings, 74 (26%) were classified as noncalcified, 60 (22%) as mixed, and 147 (52%) as calcified. In the SAP group with normal MPI findings, of the 91 plaques identified, 50 (55%) were classified as noncalcified, 17 (18%) as mixed, and 25 (27%) as calcified. The mean number of coronary plaques per patient was 5.0 ± 2.6 in the AMI group, 4.2 ± 2.6 in the SAP group with abnormal MPI findings, and 1.1 ± 1.3 in the SAP group with normal MPI findings (p <0.01; Figure 2 ). The mean number of positive remodeling and LAPs per patient was 1.9 ± 1.8 in the AMI group, 0.6 ± 0.9 in the SAP group with abnormal MPI findings, and 0.2 ± 0.4 in the SAP group with normal MPI findings (p <0.01). Of these positive remodeling and LAPs, spotty calcification was observed significantly more frequently in the AMI group (0.8 ± 1.2) than in the SAP group with abnormal MPI findings (0.4 ± 0.7) and with normal MPI findings (0.03 ± 0.2; p <0.01). In the AMI group, the number of positive remodeling and LAPs was significantly greater in patients with metabolic syndrome than in those without; however, no difference was found in the total number of plaques between these 2 groups ( Figure 3 ).
Variable | AMI (n = 60) | SAP With Abnormal MPI (n = 67) | SAP With Normal MPI (n = 83) | p Value |
---|---|---|---|---|
Age (years) | 64 ± 10 | 67 ± 12 | 66 ± 10 | 0.35 |
Men/women | 49/11 | 40/12 | 49/34 | <0.01 |
Hypertension | 33 (55%) | 37 (56%) | 43 (52%) | 0.85 |
Hyperlipidemia | 30 (50%) | 36 (54%) | 40 (48%) | 0.83 |
Diabetes mellitus | 25 (42%) | 30 (45%) | 27 (33%) | 0.41 |
Smoker | 35 (58%) | 34 (51%) | 35 (43%) | 0.23 |
Body mass index (kg/m 2 ) | 24.7 (22.0–26.3) | 24.4 (22.3–26.7) | 23.7 (20.7–26.2) | 0.50 |
Culprit coronary artery | 0.69 | |||
Right | 20 | 21 | — | |
Left anterior descending | 36 | 43 | ||
Left circumflex | 4 | 3 |
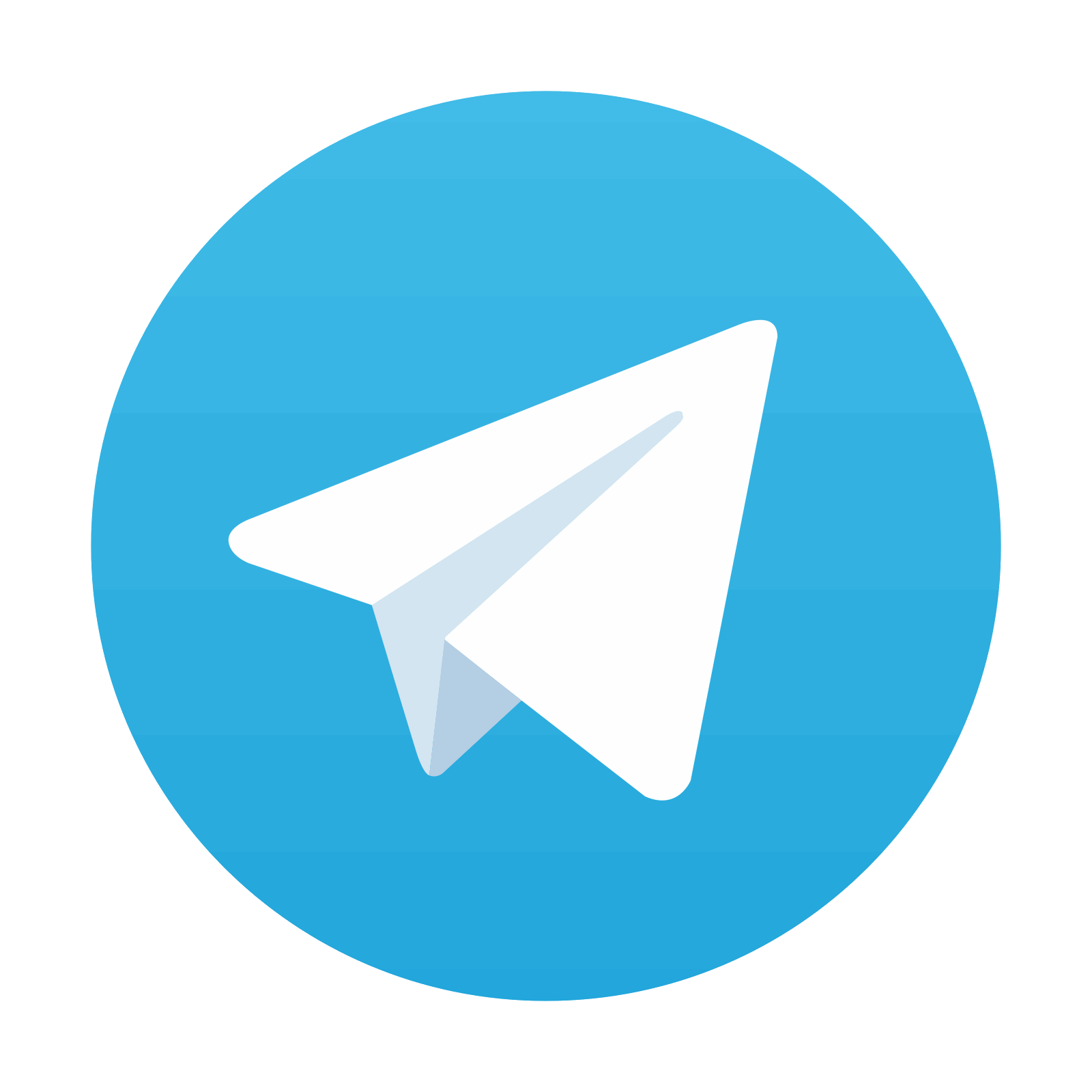
Stay updated, free articles. Join our Telegram channel

Full access? Get Clinical Tree
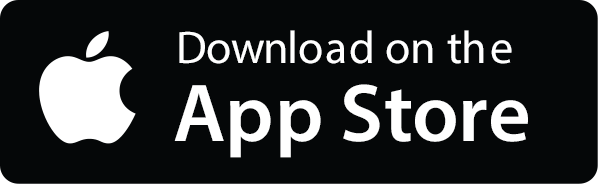
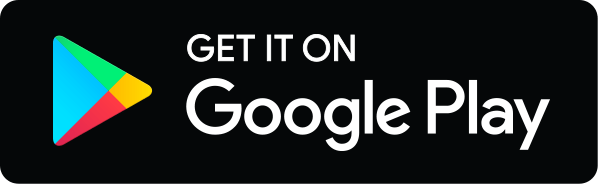
