Outcome is better in patients with idiopathic dilated cardiomyopathy (IDC) than in ischemic heart failure (HF), but morbidity and mortality are nevertheless presumed to be substantial. Most data on the prognosis in IDC stem from research performed before the widespread use of current evidence-based treatment, including implantable devices. We report outcome data from a cohort of patients with IDC treated according to current HF guidelines and compare our results with previous figures: 102 consecutive patients referred to our tertiary care hospital with idiopathic IDC and a left ventricular ejection fraction <40% were included in a prospective cohort study. After extensive baseline work-up, follow-up was performed after 6 and 13 months. Vital status and heart transplantation were recorded. Over the first year of follow-up, the patients were on optimal pharmacological treatment, and 24 patients received implantable devices. Left ventricular ejection fraction increased from 26 ± 10% to 41 ± 11%, peak oxygen consumption increased from 19.5 ± 7.1 to 23.4 ± 7.8 ml/kg/min, and functional class improved substantially (all p values <0.001). After a median follow-up of 3.6 years, 4 patients were dead, and heart transplantation had been performed in 9 patients. According to our literature search, survival in patients with IDC has improved substantially over the last decades. In conclusion, patients with IDC have a better outcome than previously reported when treated according to current guidelines.
Approximately 50% of people diagnosed with heart failure (HF) die within 5 years. The outcome is strongly related to left ventricular (LV) function. Approximately 10% of HF cases are due to idiopathic dilated cardiomyopathy (IDC). Its prognosis is generally better than in patients with ischemic HF. The 5-year mortality is substantial, however, ranging from >50% in early accounts to approximately 25% in more recent reports. Reported survival rate varies because of different inclusion criteria in different trials. In addition, there has probably been a real improvement in outcome as treatment for HF has advanced over the past 3 decades. A number of studies have demonstrated that LV function often improves considerably in patients recently diagnosed with IDC. The reason for this improvement, which is beyond what is observed after the initiation of treatment in patients with HF of ischemic cause, is poorly understood. Also, this improvement is by no means uniform and can be difficult to predict. We present outcome data from a contemporary cohort of patients with IDC treated according to current guidelines. We hypothesized that in a meticulously assessed and well-treated contemporary cohort of patients with IDC, LV reverse remodeling and functional improvement would be substantial and that the long-term prognosis would compare favorably with what has been reported previously.
Methods
This is a prospective cohort study performed at our tertiary care university hospital. The trial complies with the Declaration of Helsinki and was approved by the Regional Committee for Medical and Health Research Ethics South East. The results are reported according to the STrengthening the Reporting of OBservational studies in Epidemiology (STROBE) guidelines. All patients provided written informed consent.
Patients aged ≥18 years, admitted to our cardiology department with suspected IDC, were eligible for participation. Inclusion criteria were a dilated left ventricle (end-diastolic internal diameter ≥6.5 cm or an indexed value of >3.2 cm/m 2 ), and a left ventricular ejection fraction (LVEF) ≤40%. Ischemic, hypertensive, and primary valvular heart disease were excluded by patient history, clinical examination, echocardiography, and coronary angiography (n = 101) or computer tomography angiography (n = 1) before inclusion. Other exclusion criteria were a known or suspected cause of cardiomyopathy, including acute or previous myocarditis; inotropic or mechanical support at admittance; an implantable cardiac device (pacemaker, intracardiac defibrillator, and/or biventricular pacemaker); and significant concomitant disease such as chronic infections, connective tissue disease, severe pulmonary disease, cancer, serious psychiatric disease, life-threatening ventricular arrhythmias, severe kidney failure, or severe hepatic failure. As we are the only center in Norway to perform orthotopic heart transplantation, patients referred specifically for heart transplant evaluation were excluded to avoid outcome selection bias. Patients who were referred for diagnostic workup, and later found to be in need of heart transplantation, were not excluded. Atrial fibrillation was not an exclusion criterion, provided the ventricular rate had been well controlled (i.e., an average resting heart rate <100 beats/min) over the past 4 weeks. As we are a tertiary care hospital, all patients had been admitted to their local hospitals initially, and unless the patients were in need of immediate mechanical support or urgent evaluation for heart transplantation, we usually awaited the effect of pharmacologic intervention before the patients were transferred to our center.
At baseline, the study participants underwent physical examination; blood tests including screening for known monogenic causes of IDC; echocardiography; cardiac magnetic resonance imaging (CMR); exercise testing with measurement of peak oxygen uptake; 24-hour electrocardiogram (ECG); and right-sided cardiac catheterization with endomyocardial biopsy. Follow-up was performed after 6 months and 1 year. Patients were later followed through the Norwegian National Population Register and our national heart transplant database for mortality and heart transplantation, respectively. Patient records were acquired from local hospitals for adjudication of events.
Echocardiography was performed with Vivid 7 or E9 ultrasound scanners (GE Vingmed Ultrasound, Horten, Norway), using phased-array transducers. Three heart beats were recorded for each registration. Cine loops were digitally stored and later analyzed offline using Echo-Pac (GE Vingmed Ultrasound). Individual examinations were deidentified and randomized, and image analysis was performed blinded to patient characteristics and to whether the examination was performed at baseline or follow-up. Two-dimensional parameters and conventional Doppler parameters were measured according to current recommendations. LVEF was measured by Simpson biplane method.
Exercise testing was performed on a bicycle ergometer using an individualized, stepwise protocol, with the load incrementally increased every minute. On the basis of the patient’s age, gender, size, physical shape, and symptoms, an expected maximum load was estimated for each patient, and the test was designed to reach this load after approximately 10 minutes. Simultaneous hemodynamic monitoring and gas exchange analysis were performed (Cardiovit CS-200; Schiller, Baar, Switzerland, and Ganshorn PowerCube; Ganshorn, Niederlauer, Germany). We calculated the peak oxygen consumption per kilogram per minute and expressed the result as a percentage of the age- and-gender adjusted reference values as recommended by Wasserman et al.
Values are presented as mean ± standard deviation, median (interquartile range), or number (%) depending on the distribution. Differences across subgroups were assessed by Student t tests for normally distributed values, Mann–Whitney U tests for skewed parameters and chi-square tests for categorical data. Changes in values from baseline to follow-up were calculated by paired Student t tests for normally distributed values or by Wilcoxon signed rank tests for continuous, skewed parameters. We used Friedman test to assess changes in New York Heart Association (NYHA) functional class. Post hoc Wilcoxon signed ranks tests were performed.
Associations between baseline parameters and (1) the LVEF and (2) the age- and gender-adjusted peak oxygen consumption at 1-year follow-up were assessed by univariate and multivariate linear regression. Skewed parameters were log-transformed before regression analyses. To avoid overfitting, rather than feeding a large number of potential risk factors into an automated statistical algorithm, we a priori selected risk factors which have previously been shown to be associated with outcome in HF (age, symptom severity, duration of symptoms, heart rate and rhythm, blood pressure, LV volume and ejection fraction, peak oxygen consumption, QRS width, pulmonary capillary wedge pressure, and N-terminal-pro-B-type natriuretic peptide [NT-proBNP]). Associations between baseline values and the time to death due to HF or heart transplantation were assessed by Cox regression. p-values <0.05 were considered significant. All statistical analyses were performed in SPSS version 21.0.
We performed a PubMed search using the search string ([dilated cardiomyopathy] and [mortality or survival] and [cohort studies or cohort or longitudinal or follow-up or “follow up” or prospective or retrospective]) and restricted our search to reports in English concerning adult, human patients. In addition, we used “snowballing” to identify additional reports pertaining to mortality in IDC.
Results
Patient recruitment and follow-up are summarized in Figure 1 . From October 13, 2008, to November 16, 2012, a total of 265 consecutive patients were evaluated for participation. One hundred fifty-five patients did not fulfill the inclusion criteria, 3 patients were unwilling to participate, and 5 patients were not included for administrative reasons. One hundred two patients were thus included in the present study. Their baseline data are presented in Table 1 .
Variable | Value |
---|---|
Age (years) | 51 ± 14 |
Male | 74 (73 %) |
Body mass index (kg/m 2 ) | 28 ± 5 |
Systolic blood pressure (mm Hg) | 116 ± 20 |
Diastolic blood pressure (mm Hg) | 71 ± 12 |
Heart rate (beats/minute) | 75 ± 16 |
Atrial fibrillation | 18 (18 %) |
NYHA class (I/II/III/IV) | 15/61/20/6 |
Smokers | 24 (24 %) |
Hypertension (by history) | 18 (18 %) |
Diabetes mellitus | 4 (4 %) |
Duration of symptoms (months) | 7 (3 – 16) |
NYHA class at peak symptom severity (I/II/III/IV) | 4/15/25/58 |
QRS-duration (ms) | 110 (99 – 134) |
Left bundle branch block | 21 (21 %) |
Hemoglobin (g/dl) | 14.4 ± 1.5 |
Creatinine (mmol/l) [mg/dl] | 86 ± 21 [0.97 ± 0.24] |
N-terminal pro-B-type natriuretic peptide (pg/ml) | 1332 (584 – 2903) |
Echocardiogram | |
Left ventricular ejection fraction (%) | 26 ± 10 |
Left ventricular end diastolic diameter (cm) | 7.1 ± 0.8 |
Left ventricular end diastolic internal volume (ml) | 267 (216 – 328) |
Cardiac output (l/min) | 4.9 ± 1.4 |
Cardiac magnetic resonance imaging (88 patients) | |
Left ventricular ejection fraction (%) | 28 ± 11 |
Left ventricular end diastolic internal volume (ml) | 273 (214 – 356) |
Left ventricular myocardial volume (ml) | 212 ± 62 |
Gadolinium late enhancement | 29 (35 %) |
Exercise testing (96 patients) | |
Maximum work load (Watts) | 133 ± 56 |
Peak heart rate (beats per minute) | 151 ± 25 |
Peak oxygen uptake (ml/kg/min) | 19.7 ± 7 |
Peak oxygen uptake as percentage of expected value | 69 ± 22 |
Cardiac catheterization (97 patients) | |
Right atrial pressure (mmHg) | 6 (4-10) |
Mean pulmonary artery pressure (mmHg) | 24 ± 10 |
Pulmonary capillary wedge pressure (mmHg) | 15 ± 8 |
Cardiac output (l/min) | 4.9 ± 1.5 |
24-hour electrocardiogram (89 patients) | |
Average heart rate (beats per minute) | 76 ± 13 |
Minimum heart rate (beats per minute) | 49 ± 10 |
Maximum heart rate (beats per minute) | 142 ± 28 |
Ventricular tachycardia | 25 (28 %) |
By design, all patients were diagnosed with IDC before inclusion. Extensive workup led to a definitive etiologic diagnosis in 14 patients: in 9 patients, a probable disease-causing mutation was discovered; 2 patients had inflammatory myocardial disease as diagnosed by CMR and/or endomyocardial biopsy; 1 patient had transthyretin amyloidosis as determined by endomyocardial biopsy; and 2 patients had noncompaction cardiomyopathy detected by CMR. Pharmacologic treatment and device implantations are presented in Table 2 . By design, no patients had implantable devices at inclusion.
Variable | Baseline | Six months | 13 months |
---|---|---|---|
Medication | |||
Angiotensin converting enzyme inhibitor and/or angiotensin II receptor blocker | 99 (97 %) | 95 (98 %) | 93 (98 %) |
Angiotensin converting enzyme inhibitor and/or angiotensin II receptor blocker (% of optimal daily dose) | 74 | 90 | 92 |
Beta blocker | 95 (93 %) | 96 (99 %) | 94 (99 %) |
Beta blocker (% of optimal daily dose) | 47 | 72 | 74 |
Aldosterone antagonist | 22 (22 %) | 30 (29 %) | 31 (33 %) |
Diuretic | 72 (71 %) | 61 (63 %) | 52 (55 %) |
Digoxin or digitoxin | 30 (29 %) | 15 (15 %) | 13 (14 %) |
Implantable devices and heart transplantations | |||
Cardioverter defibrillator | 0 | 19 (20 %) | 20 (21 %) |
Cardiac resynchronization therapy | 0 | 9 (9 %) | 9 (9 %) |
Cardiac resynchronization therapy defibrillator | 0 | 5 (5 %) | 5 (5 %) |
Left ventricular assist device ∗ | 0 | 1 (1 %) | 0 |
Heart transplantation | 0 | 2 (2 %) | 3 (3 %) |
∗ Left ventricular assist devices were used as bridge to transplantation.
At 6-month follow-up, 97 patients were reexamined. One patient was on an LV assist device awaiting heart transplantation, 2 patients had received cardiac allografts, and 2 patients did not appear for their scheduled appointments. At 13 (12 to 16) months, 95 patients were reexamined: in addition to the 3 heart transplant recipients, 4 patients did not appear for their appointments. One of these patients did not show up for either of the 2 scheduled follow-up appointments and was thus lost to short-term follow-up ( Figure 1 ).
Before treatment initiation, the large majority of patients were severely symptomatic. During follow-up, there was a highly significant change in NYHA classification overall (chi-square [3] = 200.7; p <0.001), most of which occurred shortly after treatment initiation ( Figure 2 ).
A substantial increase in LVEF was observed in transplant-free survivors throughout the first year of follow-up ( Table 3 and Figure 3 ). From baseline to 13 months, 69 patients experienced an increase in LVEF by ≥5 percentage points, 7 patients had a decrease in LVEF by ≥5 percentage points, and in 19 patients, there was no change (between −5 and +5 percentage points change). After 13 months, 22 patients (22%) had achieved complete LV recovery, defined as an LVEF >50%, 11 of whom also had a normal NT-proBNP value at this time point. Most of the improvement in LVEF took place shortly after presentation, and after 6 months, it had increased to 37 ± 9%. On univariate analyses, the duration of symptoms before inclusion, QRS width, and LV end-diastolic volume on presentation were associated with LVEF 1 year later. LVEF at baseline was not associated with LVEF at follow-up. Only LV end-diastolic volume and the duration of symptoms on presentation remained independent predictors of 1-year LVEF in the multivariate model ( Table 4 ). The duration of symptoms in patients who recovered by >10 LVEF points was 5 (2 to 8) months versus 12 (7 to 35) months in patients who did not recover (p <0.001). Of note, viral persistence as examined by polymerase chain reaction in myocardial biopsies (p = 0.63), a monogenic etiology (p = 0.44), or gadolinium late enhancement (p = 0.82) at baseline was not associated with LVEF at follow-up.
Variable | Baseline | 13 months | Mean change ∗ | p-value |
---|---|---|---|---|
Systolic blood pressure (mm Hg) | 116 ± 20 | 122 ± 18 | 6 (2 – 10) | 0.002 |
Diastolic blood pressure (mm Hg) | 71 ± 12 | 70 ± 11 | 0 (-2 – 3) | 0.70 |
Resting heart rate (beats/minute) | 75 ± 16 | 66 ± 14 | -9 (-12 – -5) | <0.001 |
New York Heart Association class I/II/III/IV | 15/61/20/6 | 51/37/6/1 | – | <0.001 |
Left ventricular end diastolic volume (ml) | 267 (216 – 328) | 186 (154 – 244) | -81 (-96 – -65) | <0.001 |
Left ventricular ejection fraction (ml) | 26 ± 10 | 40 ± 11 | 13 (11 – 16) | <0.001 |
Cardiac output at rest (l/min) | 4.9 ± 1.4 | 4.9 ± 1.0 | 0 (-0.3 – 0.3) | 0.97 |
Laboratory values | ||||
Creatinine (mmol/l) | 86 ± 21 | 86 ± 29 | 0 (-6 – 5) | 0.97 |
C-reactive protein (mg/l) | 3.0 (1.1 – 7.6) | 2.1 (0.9 – 4.4) | -4.8 (-8.8 – -1.2) † | 0.01 |
N-terminal pro-B-type natriuretic peptide (pg/dl) | 1332 (583 – 2903) | 330 (127 – 706) | -1182 (-1756 – -611) † | <0.001 |
Exercise testing | ||||
Peak heart rate (beats per minute) | 151 ± 25 | 150 ± 25 | -3 (-2 – 8) | 0.29 |
Peak load (Watts) | 133 ± 56 | 153 ± 59 | 19 (10 – 28) | <0.001 |
Peak oxygen consumption (ml/kg/min) | 19.5 ± 7.1 | 23.4 ± 7.8 | 3.7 (2.5 – 4.9) | <0.001 |
Peak oxygen consumption (% of expected value) | 69 ± 22 | 84 ± 23 | 14 (10 – 18) | <0.001 |
∗ This column contains the population average change and the 95% confidence interval of this mean.
† These confidence intervals were estimated by bootstrapping.
Variable | Univariate r | p-value | Multivariate β | p-value |
---|---|---|---|---|
Age | -0.07 | 0.48 | 0.04 | 0.75 |
Duration of symptoms ∗ | -0.46 | <0.001 | -0.55 | <0.001 |
New York Heart Association class II | – | 0.09 † | 0.23 | 0.11 |
New York Heart Association class III | 0.08 | 0.61 | ||
New York Heart Association class IV | -0.11 | 0.38 | ||
Atrial fibrillation | – | 0.11 † | -0.06 | 0.59 |
Systolic blood pressure | 0.14 | 0.18 | 0.14 | 0.23 |
Left ventricular ejection fraction | 0.18 | 0.19 | -0.10 | 0.52 |
Left ventricular end diastolic volume | -0.29 | 0.004 | -0.41 | 0.006 |
Peak oxygen consumption (% of expected) | 0.15 | 0.15 | -0.05 | 0.73 |
Pulmonary capillary wedge pressure | -0.08 | 0.43 | 0.04 | 0.73 |
QRS width | -0.22 | 0.03 | -0.16 | 0.21 |
N-terminal pro-B-type natriuretic peptide ∗ | -0.12 | 0.23 | 0.04 | 0.77 |
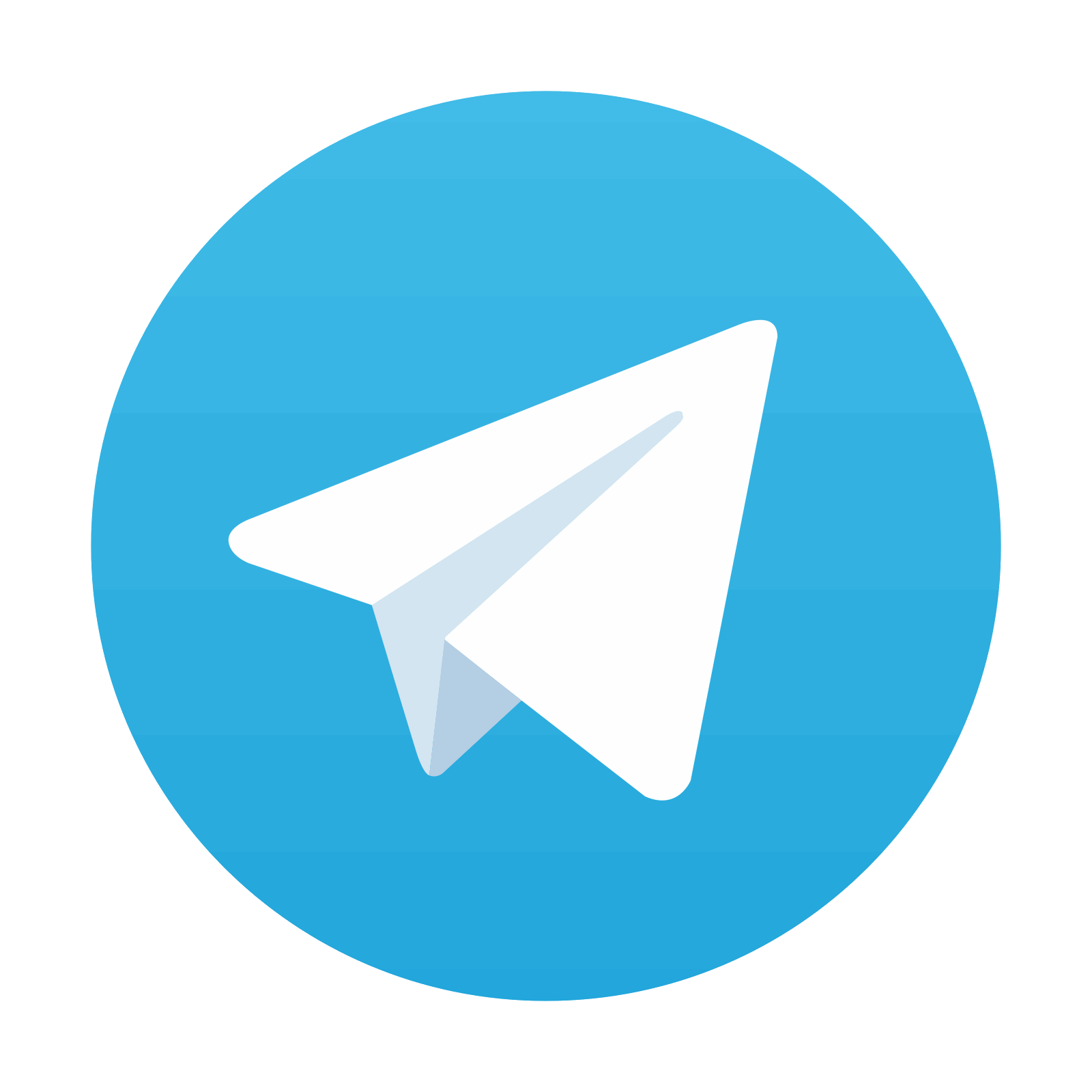
Stay updated, free articles. Join our Telegram channel

Full access? Get Clinical Tree
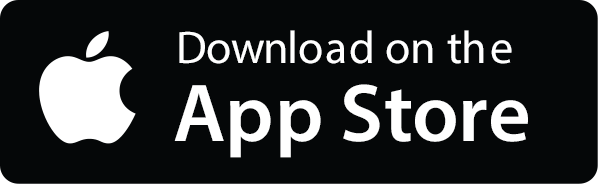
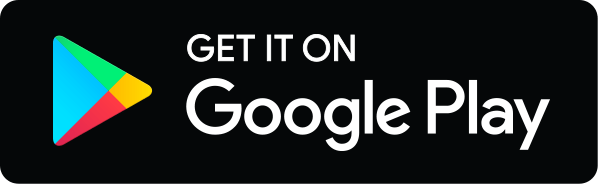
