Gary D. Webb, Jeffrey F. Smallhorn, Judith Therrien, Andrew N. Redington
Congenital Heart Disease
This chapter has been written for practicing cardiologists and is compatible with the existing expert management recommendations1–3 for the care of adult patients with congenital cardiac defects. Although concentrating on the issues of late adolescence and adulthood, such issues can be appreciated only in the setting of a comprehensive understanding of the anatomy, physiology, and events during childhood, and these issues are discussed in each section. Additional information can be found in other sources.4,5 Congenital cardiovascular disease is defined as an abnormality in cardiocirculatory structure or function that is present at birth, even if discovered much later. Congenital cardiovascular malformations usually result from altered embryonic development of a normal structure or failure of such a structure to progress beyond an early stage of embryonic or fetal development. The aberrant patterns of flow created by an anatomic defect may, in turn, significantly influence the structural and functional development of the remainder of the circulation. For example, the presence in utero of mitral atresia may prohibit normal development of the left ventricle, aortic valve, and ascending aorta. Similarly, constriction of the fetal ductus arteriosus may result in right ventricular dilation and tricuspid regurgitation in the fetus and newborn, contribute importantly to the development of pulmonary arterial aneurysms in the presence of a ventricular septal defect (VSD) and absent pulmonary valve, or result in an alteration in the number and caliber of fetal and newborn pulmonary vascular resistance vessels.
Postnatal events can markedly influence the clinical features of a specific “isolated” malformation. Infants with Ebstein malformation of the tricuspid valve may improve dramatically as the magnitude of tricuspid regurgitation diminishes with the normal fall in pulmonary vascular resistance after birth, and infants with pulmonary atresia or severe stenosis may not become cyanotic until normal spontaneous closure of a patent ductus arteriosus (PDA) takes place. Ductal constriction many days after birth may also be a central factor in some infants in the development of coarctation of the aorta. Still later in life, patients with a VSD may experience spontaneous closure and the development of right ventricular outflow tract obstruction and/or aortic regurgitation with increasing duration of follow-up. These selected examples serve to emphasize that anatomic and physiologic changes in the heart and circulation can continue to evolve from the prenatal period to late adult life.
Anatomy
Normal Cardiac Anatomy
The key to understanding CHD is having an appreciation of the segmental approach to the diagnosis of both simple and complex lesions.
Cardiac Situs
This refers to the status of the atrial appendages. The normal left atrial appendage is a finger-like structure with a narrow base and no guarding crista. On the other hand, the right atrial appendage is broad based and has a guarding crista and pectinate muscles. Situs solitus or inversus refers to hearts with both a morphologic left and right atrium. Situs ambiguus refers to hearts with two morphologic left or right atrial appendages. These conditions are dealt with in the section on isomerism and have implications with regard to associated intracardiac and extracardiac abnormalities.
Atrioventricular Connections
This refers to the connections between the atria and ventricles. The AV connections are said to be concordant if the morphologic left atrium is connected to the morphologic left ventricle via the mitral valve, with the morphologic right atrium connecting to the morphologic right ventricle via a tricuspid valve. They are said to be discordant in other circumstances, such as in congenitally corrected TGA (cc-TGA), and to be univentricular when both atria connect predominantly to one of the ventricles.
Ventriculoarterial Connections
This refers to the connections between the semilunar valves and ventricles. Ventriculoarterial concordance occurs when the morphologic left ventricle is connected to the aorta and the morphologic right ventricle is connected to the pulmonary artery. Ventriculoarterial discordance occurs when the morphologic left ventricle is connected to the pulmonary artery and the aorta is connected to the morphologic right ventricle. Double-outlet right ventricle occurs when more than 50% of both great arteries is connected to the morphologic right ventricle. A single-outlet heart has only one great artery connected to the heart.
Atria
Assignment of either a morphologic left or right atrium is determined by the morphology of the atrial appendages and not by the status of the systemic or pulmonary venous drainage. The right atrial appendage is broad and triangular, whereas the left one is smaller and finger-like. The internal architecture is the key feature to an accurate diagnosis, with the right having extensive pectinate muscles that run around the vestibule of the atrium, unlike its left counterpart. Although the pulmonary veins usually drain to a morphologic left atrium and the systemic veins drain into a morphologic right atrium, such is not always the case.
Atrioventricular Valves
The morphologic mitral valve is a bileaflet valve with the anterior or aortic leaflet in fibrous continuity with the noncoronary cusp of the aortic valve. The mitral valve leaflets are supported by two papillary muscle groups located in the anterolateral and posteromedial positions. Each papillary muscle supports the adjacent part of both valve leaflets, with considerable variation in morphology of the papillary muscles.
The tricuspid valve is a trileaflet valve, although it can frequently be difficult to identify all three leaflets because of variability in the anteroposterior commissure. With close inspection, the commissural chordae that arise from the papillary muscles may permit identification of the three leaflets. The three leaflets occupy a septal anterior, superior, and inferior position. The commissures between the leaflets are the anterior septal, anterior inferior, and inferior commissures. The papillary muscles supporting the valve leaflets arise mostly from the trabeculoseptomarginalis and its apical ramifications.
Morphologic Right Ventricle
The morphologic right ventricle is a triangular-shaped structure with inlet, trabecular, and outlet components. The inlet component of the right ventricle has attachments from the septal leaflet of the tricuspid valve. Inferior to this is the moderator band, which arises at the base of the trabeculoseptomarginalis, with extensive trabeculations toward the apex of the right ventricle. The outlet component of the right ventricle consists of a fusion of three structures (i.e., the infundibular septum separating the aortic from the pulmonary valve, the ventriculoinfundibular fold separating the tricuspid valve from the pulmonary valve, and finally the anterior and posterior limbs of the trabeculoseptomarginalis).
Morphologic Left Ventricle
The morphologic left ventricle is an elliptical-shaped structure with a fine trabecular pattern and absent septal attachments of the mitral valve in a normal heart. It consists of an inlet portion containing the mitral valve and a tension apparatus, an apical trabecular zone that is characterized by fine trabeculations, and an outlet zone that supports the aortic valve.
Semilunar Valves
The aortic valve is a trileaflet valve with the left and right cusps giving rise to the left and right coronary arteries, respectively, and the noncoronary cusp lacking a coronary artery connection. Of note, the noncoronary cusp is in fibrous continuity with the anterior leaflet of the mitral valve. The aortic valve has a semilunar attachment to the junction of the ventricular outlet and its great arteries. The aortic cusps have a main core of fibrous tissue with endocardial linings on each surface. The cusps are thickened at the midpoint to form a nodule. The characteristics of the pulmonary valve are similar to those of its aortic counterpart, but with no coronary ostia arising at the superior portion of the sinuses.
Aortic Arch and Pulmonary Arteries
In a normal heart the aortic arch usually points to the left, with the first branch, the innominate artery, giving rise to the right carotid and subclavian arteries. In general, the left carotid and left subclavian arteries arise separately from the aortic arch. By definition, the ascending aorta is proximal to the origin of the innominate artery, with the transverse aortic arch extending from the innominate artery to the origin of the left subclavian artery. The aortic isthmus is the area between the left subclavian artery and a PDA or ligamentum arteriosum.
Systemic Venous Connections
In a normal heart the left and right innominate veins form the superior caval vein, which connects to the roof of the right atrium. The inferior caval vein connects to the inferior portion of the morphologic right atrium, and the hepatic veins join the inferior caval vein before its insertion into the atrium. The coronary veins drain into the flow of the coronary sinus, with the latter running in the posterior AV groove and terminating in the right atrium. The inferior caval vein is guarded by the eustachian valve and may vary in size.
Pulmonary Venous Drainage in a Normal Heart
The pulmonary veins drain to the left-sided atrium. Usually, three pulmonary veins arise from the trilobed right lung and two pulmonary veins from the bilobed left lung. The pulmonary veins drain into the left atrium in superior and inferior locations. There is a short segment of extraparenchymal pulmonary vein before disappearing into the adjacent hila of the lungs.
Pathologic Consequences of Congenital Cardiac Lesions
Congestive Heart Failure (see Chapters 21 to 29)
Although the basic mechanisms of cardiac failure are similar for all ages, the common causes, time of onset, and frequently the approach to treatment vary with age (see Chapters 24 to 27). Fetal echocardiography now allows the diagnosis of intrauterine cardiac failure. The cardinal findings of fetal heart failure are scalp edema, ascites, pericardial effusion, and decreased fetal movements. In preterm infants, especially those less than 1500 g in birth weight, persistent patency of the ductus arteriosus is the most common cause of cardiac decompensation, and other forms of structural heart disease are rare. In full-term newborns the earliest important causes of heart failure are hypoplastic left heart and aortic coarctation syndromes, sustained tachyarrhythmia, cerebral or hepatic arteriovenous fistula, and myocarditis. Among the lesions commonly producing heart failure beyond 1 to 2 weeks of age, when diminished pulmonary vascular resistance allows substantial left-to-right shunting, are VSDs and AV septal defects, TGA, truncus arteriosus, and total anomalous pulmonary venous connection. Infants younger than 1 year with cardiac malformations account for 80% to 90% of pediatric patients in whom congestive heart failure develops. In older children, heart failure is often due to acquired disease or is a complication of open heart surgical procedures. In the acquired category are rheumatic and endomyocardial diseases, infective endocarditis, hematologic and nutritional disorders, and severe cardiac arrhythmias.
The distinction between left- and right-sided heart failure is less obvious in infants than in older children or adults. Conversely, augmented filling or elevated pressure of the right ventricle in infants reduces left ventricular compliance disproportionately when compared with older children or adults and gives rise to signs of both systemic and pulmonary venous congestion.
Care of infants with heart failure must include careful consideration of the underlying structural or functional disturbance. The general aims of treatment are to achieve an increase in cardiac performance, augment peripheral perfusion, and decrease pulmonary and systemic venous congestion. In many conditions, medical management cannot control the effects of the abnormal loads imposed by a host of congenital cardiac lesions. In these circumstances, interventional catheterization or surgery (see Chapter 28) may be urgently required.
Congestive heart failure is not common in adult CHD practice, although prevention of myocardial dysfunction is a common concern. Heart failure may develop in adult patients with CHD in the presence of a substrate (e.g., myocardial dysfunction, valvular regurgitation) and a precipitant (e.g., sustained arrhythmia, pregnancy, or hyperthyroidism). Patients prone to the development of congestive failure include those with longstanding volume loads (e.g., valvular regurgitation and left-to-right shunts) and those with primary depression of myocardial function (e.g., systemic right ventricles, ventricles damaged during surgery or because of late treatment of ventricular pressure or volume overload). Treatment depends on a clear understanding of the elements contributing to the decompensation and on addressing each of the treatable components. Standard palliative adult heart failure regimens are frequently used and may include ACE inhibitors, angiotensin receptor blockers, beta blockers, diuretics, resynchronization pacing, transplantation, and other novel therapies. Evidence of the effectiveness of these strategies is relatively lacking, and many of the proven therapies used for heart failure in patients with acquired heart disease have failed to show a demonstrable benefit in treating CHD.8
CHD accounts for 40% of pediatric heart transplants but only 2% of adult heart transplants.9 Adults receiving heart transplants because of CHD have a mean survival of 11 years, similar to that of patients with other forms of heart disease. Patients who have undergone Fontan surgery tend to have worse outcomes, presumably because they have multiorgan disease.10 Approximately a third of heart-lung transplants are done for CHD. The 3-year survival rate is approximately 50%, but it is better in patients with Eisenmenger syndrome.
Cyanosis
Definition
Central cyanosis refers to arterial oxygen desaturation resulting from shunting or mixing of systemic venous blood into the arterial circulation. The magnitude of shunting or mixing and the amount of pulmonary blood flow determine the severity of desaturation.
Morphology
Cardiac defects that result in central cyanosis can be divided into two categories: (1) those with increased pulmonary blood flow and (2) those with decreased pulmonary blood flow (Table 62-4).
TABLE 62-4
Cardiac Defects Causing Central Cyanosis
Transposition of the great arteries | Ebstein anomaly |
Tetralogy of Fallot | Eisenmenger physiology |
Tricuspid atresia | Critical pulmonary stenosis or atresia |
Truncus arteriosus | Functionally single ventricle |
Total anomalous pulmonary venous return |
Note the five T’s and two E’s.
Pathophysiology
Hypoxemia increases renal production of erythropoietin, which in turn stimulates the bone marrow to produce circulating red blood cells, thereby enhancing oxygen-carrying capacity. Secondary erythrocytosis should be present in all cyanotic patients because it is a physiologic response to tissue hypoxia. The improved tissue oxygenation that results from this adaptation may be sufficient to reach a new equilibrium at a higher hematocrit. However, adaptive failure can occur if the increased whole blood viscosity rises so much that oxygen delivery is impaired.11
Clinical Features
Hyperviscosity Syndrome
Although erythrocytosis is now rare because of the diminishing prevalence of untreated cyanotic CHD, it may cause symptoms of hyperviscosity, including headaches, faintness, dizziness, fatigue, altered mentation, visual disturbances, paresthesias, tinnitus, and myalgias. Iron deficiency, a common finding in cyanotic adult patients if repeated phlebotomies or excessive bleeding occurs, must be treated because it can increase the risk for complications.
Hematologic
Hemostatic abnormalities have been documented in cyanotic patients with erythrocytosis and can occur in up to 20% of patients. The bleeding tendency can be mild and superficial and lead to easy bruising, skin petechiae, and mucosal bleeding, or it can be moderate or life-threatening with hemoptysis or intracranial, gastrointestinal, or postoperative bleeding. An elevated prothrombin and partial thromboplastin time; decreased levels of factors V, VII, VIII, and IX; qualitative and quantitative platelet disorders; increased fibrinolysis; and systemic endothelial dysfunction from increased shear stress have all been implicated.
Central Nervous System
Neurologic complications, including cerebral hemorrhage, can arise secondary to hemostatic defects and can occur in patients taking anticoagulants. Patients with right-to-left shunts may be at risk for paradoxical cerebral emboli, especially if they are iron deficient. A brain abscess should be suspected in a cyanotic patient with a new or different headache or new neurologic symptoms. Air filters should be used in peripheral and central venous lines in cyanotic patients to avoid paradoxical emboli through a right-to-left shunt.
Renal
Renal dysfunction in patients with cyanotic CHD can be manifested as proteinuria, hyperuricemia, or renal failure. Pathologic studies at the level of the glomeruli show evidence of vascular abnormalities, as well as increased cellularity and fibrosis. Hyperuricemia is common and is thought to be due mainly to the decreased reabsorption of uric acid rather than to overproduction associated with erythrocytosis. Urate nephropathy, uric acid nephrolithiasis, and gouty arthritis may occur.
Arthritic
Rheumatologic complications of cyanotic CHD include gout and, especially, hypertrophic osteoarthropathy, which is thought to be responsible for the arthralgias and bone pain affecting up to a third of patients. In patients with right-to-left shunting, megakaryocytes released from bone marrow can bypass the lung. Entrapment of megakaryocytes in the systemic arterioles and capillaries induces the release of platelet-derived growth factor and thus promotes local cell proliferation. New osseous formation with periostitis ensues and gives rise to arthralgia and bone pain.
Coronary Arteries
Patients with central cyanosis display dilated coronaries, and atherosclerotic narrowing is rare. Their level of total cholesterol is also lower than that in the general population.
Interventional Options and Outcomes
Complete Repair
Physiologic or anatomic repair results in total or near-total separation of the pulmonary and systemic circulations in complex cyanotic lesions, which leads to the relief of cyanosis and shunting. Such procedures should be performed whenever feasible. Complete repairs are rarely without long-term sequelae despite the inference in the name, and both physicians and patients should be made aware of the need for regular follow-up in almost all cases.
Palliative Surgical Intervention
Palliative surgical interventions can be performed in patients with cyanotic lesions to increase pulmonary blood flow while allowing the cyanosis to persist. Palliative surgical shunts are summarized in Table 62-5. Blalock-Taussig-Thomas, central, and Glenn (also called cavopulmonary) shunts are still in use today. Blalock-Taussig-Thomas shunts seldom caused pulmonary hypertension when compared with central shunts and were less prone to causing pulmonary artery distortion. Glenn shunts have the advantage of increasing pulmonary flow without imposing a volume load on the systemic ventricle. Glenn shunts require low pulmonary artery pressures to work, and they may be associated with the development over time of pulmonary arteriovenous fistulas, which can worsen the cyanosis.
TABLE 62-5
Palliative Systemic-to-Pulmonary Shunts
Arterial |
Venous |
LPA = left PA; PA = pulmonary artery; RPA = right PA; SVC = superior vena cava.
Transplantation (see Chapter 28)
Transplantation of the heart, transplantation of one or both lungs with surgical cardiac repair, and heart-lung transplantation have been performed in cyanotic patients with or without palliation who were no longer candidates for other forms of intervention. Pulmonary vascular obstructive disease precludes isolated heart transplantation. An increasing number of CHD patients with previous palliation and ventricular failure are successfully undergoing cardiac transplantation.9 The timing of transplantation in these patients remains difficult to determine, however.
Other Management of Cyanosis
Phlebotomy
The goal of phlebotomy is control of symptoms. When patients have troubling symptoms of hyperviscosity, are iron replete (normal mean corpuscular volume, hematocrit >65%), and are not dehydrated, removal of 250 to 500 mL of blood over a 30- to 45-minute period should be performed with concomitant quantitative volume replacement. The procedure may be repeated every 24 hours until symptomatic improvement occurs or the hemoglobin level has fallen below 18 to 19 g/dL. Phlebotomy is not indicated for asymptomatic patients.
Iron Replacement
If iron deficiency anemia is found, iron supplements should be prescribed.12 Cyanotic patients should avoid iron deficiency, which can cause functional deterioration and is associated with an increased risk for stroke and adverse cardiovascular outcome.
Bleeding Diathesis
Platelet transfusions, fresh frozen plasma, vitamin K, cryoprecipitate, and desmopressin can be used to treat severe bleeding. Given the inherent tendency of cyanotic patients to bleed, aspirin, heparin, and warfarin should be avoided unless the risks associated with treatment are outweighed by the risks inherent in nontreatment. Similarly, nonsteroidal anti-inflammatory drugs should be avoided to prevent gastrointestinal bleeding.
Gouty Arthritis
Symptomatic hyperuricemia and gouty arthritis can be treated as needed with colchicine, probenecid, or allopurinol.
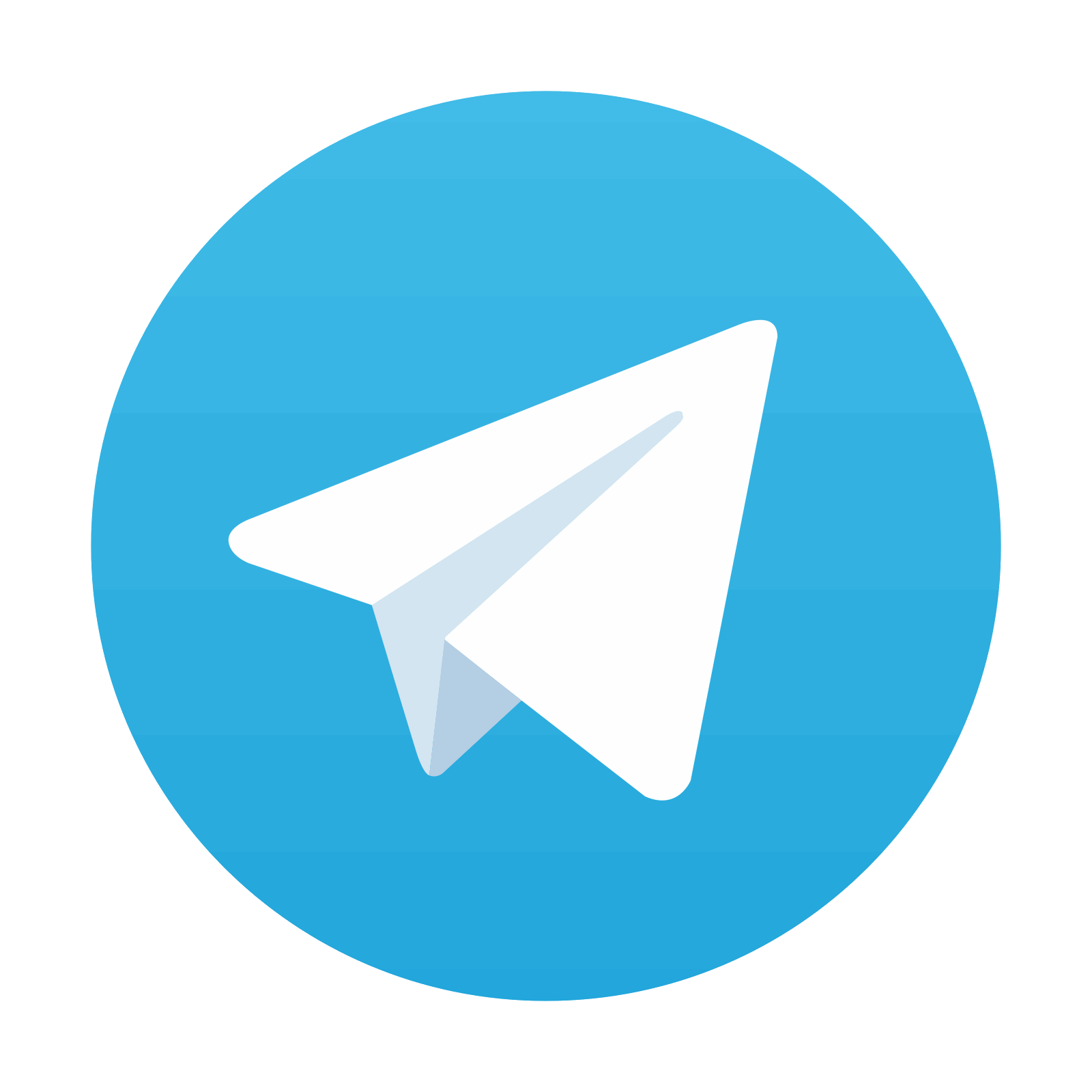
Stay updated, free articles. Join our Telegram channel

Full access? Get Clinical Tree
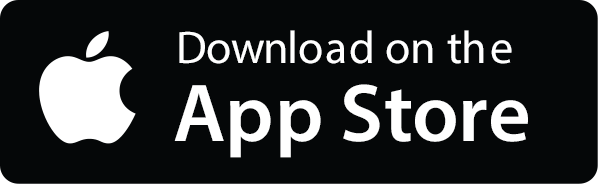
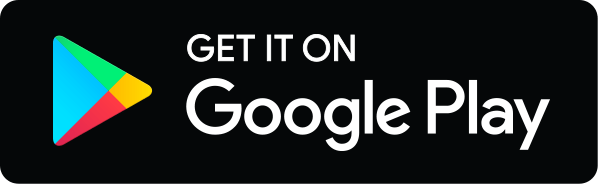