Concept Review
Action Potential—Myocardial Cell
The different phases of the action potential relate directly to the waveforms, intervals, and segments that constitute a cardiac cycle on the ECG. Each phase is distinguished by an alteration in cell membrane permeability to sodium, potassium, and calcium ions. A basic understanding of these phases and their major ion currents will help in learning the ECG features associated with conduction abnormalities, drug toxicities, and electrolyte disturbances.
The action potential of the myocardial cell is divided into five phases (phases 0-4). The first phase, phase 0, represents ventricular depolarization. Rapid depolarization depends on initial sodium entry that triggers the explosive influx of more sodium through fast-gated sodium channels. This phase takes the myocardial cell from its resting potential of −90 mV to a positive potential of 20 mV. The summation of this phase across the ventricular myocardium is represented on the ECG as the QRS wave.
![]() FIGURE 1.1 Action potential of a myocardial cell and phases of the action potential as they relate to ECG waves and segments.1 The action potential also represents the cell membrane in this figure, with the area under the action potential representing intracellular space and the area above the action potential representing extracellular space. |
Ventricular repolarization occurs during phases 1, 2, and 3. By phase 1, the fast sodium channels are closed (and inactivated), and the cell returns to a neutral potential by the opening of potassium channels that allow for the outward movement of potassium.
During phase 2, the neutral potential is maintained by balancing potassium efflux with the sustained entry of calcium ions. Calcium entry during this phase initiates the release of intracellular calcium stores necessary for sarcomere shortening and ventricular contraction. The ST segment corresponds to phase 2.
Phase 3 represents rapid repolarization to the negative resting potential by potassium ion efflux and the closure of calcium channels on the cell membrane. This phase corresponds to the T wave.
After the membrane returns to the resting potential, this potential is maintained during phase 4 by continued potassium efflux and the Na+/K+ ATPase pump (red oval). The ventricular myocardium is at its resting potential (phase 4) between the end of the T wave and the beginning of the next QRS wave (T-Q segment).
Action Potential—Pacemaker Cell
Cardiac pacemaker cells are specialized to spontaneously depolarize and initiate action potentials. The action potential of pacemaker cells is divided into three phases (phases 0, 3, and 4). Spontaneous phase 4 depolarization (represented by an upsloping phase 4 in the action potential) distinguishes pacemaker cells from myocardial cells. During this phase, slow inward sodium current results in the gradual rise of the membrane potential toward its threshold potential. The current responsible for phase 4 depolarization is also known as the pacemaker current or funny current (If).
Once threshold potential is attained, calcium channels open to depolarize the cell during phase 0.
Repolarization occurs in phase 3 with the opening of potassium channels and closure of calcium channels. When the membrane potential returns to its resting potential, sodium channels immediately open again.
![]() FIGURE 1.2 Action potential of a pacemaker cell. Lines of the action potential also represent the cell membrane of the pacemaker cell. |
The slope of phase 4 is directly affected by sympathetic and parasympathetic tone. Sympathetic stimulation results in a steeper phase 4 and consequently a faster heart rate. Norepinephrine (NE) released from sympathetic nerves increases the membrane’s permeability to sodium. Vagal tone decreases the slope of phase 4 and, consequently, the heart rate. Acetylcholine (ACh) released by the vagus nerve increases the cell membrane’s permeability to potassium while decreasing its permeability to sodium.2
Refractory Periods
Once myocardial cells have entered phase 0 depolarization, the cells become refractory to the conduction of incoming impulses. This allows cells to recover from depolarization. The relative differences in refractory states depend on the state of the fast sodium channels. Refractory states are defined by the strength of impulse required for a myocardial cell to generate and propagate an action potential. Figure 1.4 shows how these different refractory periods relate to phases of the action potential.2 Refractory periods are especially relevant to ECG rhythms resulting from a reentry circuit and to antiarrhythmic drugs that prolong the duration of the action potential.
Effective Refractory Period
During phases 0 to 2 and part of phase 3, the myocardial cell is unable to propagate an action potential in response to a stimulus, regardless of the strength of that stimulus.
Relative Refractory Period
Before the cell has returned to its resting membrane potential of −90 mV, it may respond to a stronger than normal stimulus. This can occur during phase 3 of repolarization. The response to this stimulus is slower than normal.
Supranormal Period
A smaller than normal impulse can elicit a normal action potential.
Reentry Circuits
A reentrant circuit consists of two separate pathways that differ in refractory period duration. An impulse may simultaneously encounter a group of refractory cells and a group of cells ready to conduct. Conduction preferentially occurs down the pathway out of its refractory period.
Drugs that prolong the action potential and effective refractory period are used to terminate reentrant circuits.
Class IA Antiarrhythmics
Quinidine, procainamide, and disopyramide prolong the effective refractory period by blocking the sodium channels responsible for rapid depolarization, thereby prolonging phase 0. This is represented on the ECG by QRS widening.
Class III Antiarrhythmics
Amiodarone, dofetilide, and ibutilide prolong the action potential duration by blocking the outward flow of potassium during phase 3. This increases the effective refractory period of cardiac myocytes. On the ECG, this is represented by QT prolongation.
Conduction Anatomy
Normal conduction is essential to coordinated and efficient ventricular contraction. Impulses from the sinus node activate the atrial myocardium and travel down internodal tracts to the AV node. After a brief delay at the AV node, simultaneous conduction down the right and left bundle branches allows for synchronous contraction of the right and left ventricles. Simultaneous conduction down the left anterior and posterior fascicles of the left bundle branch allows for coordinated contraction of the left ventricular free wall.
Retrograde Conduction Patterns
Basic teaching of conduction anatomy addresses anterograde conduction down normal pathways from the sinus node to the His-Purkinje system. Conduction can also occur in retrograde fashion (in a ventricular to atrial direction) through normal and bypass conduction pathways.
From Atrium to Sinus Node
A premature atrial impulse travels to both the AV node and sinus node (A). This impulse can depolarize the sinus node and effectively reset the pacemaker while it travels through the AV node and His-Purkinje system.
From AV Node to Atrium
Retrograde conduction can also occur from a focus or reentrant circuit within the AV node (B). Retrograde conduction from the AV node to atria can result in atrial depolarization. When not buried by a QRS wave, this can result in the appearance of a retrograde P wave on the ECG.
Concealed Retrograde Conduction
Retrograde conduction may reach the AV node but fail to penetrate and depolarize atrial tissue (C). By reaching the AV node, however, a retrograde impulse can render the AV node refractory to the next incoming atrial impulse. The next sinus P wave will be blocked and appear without an associated QRS complex.
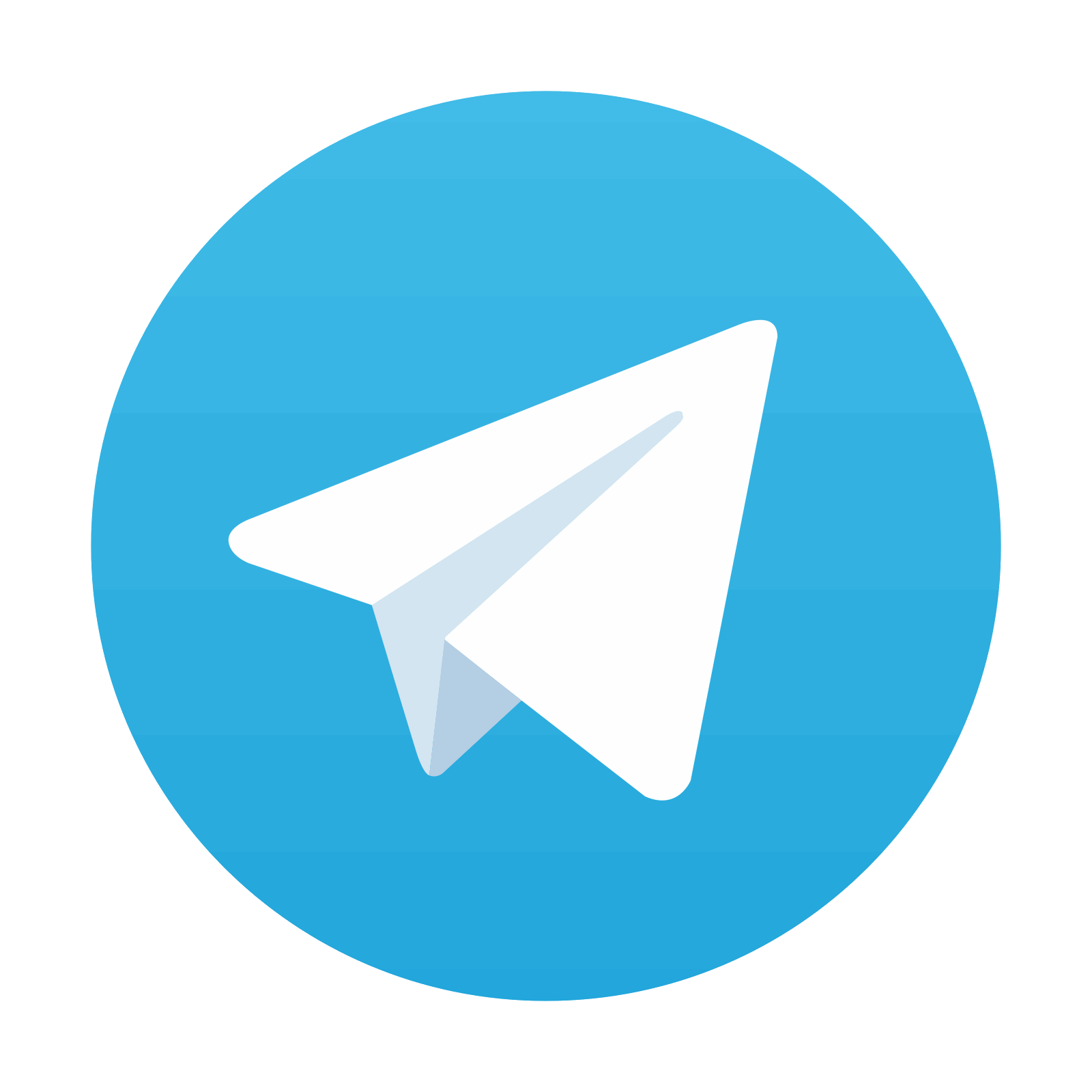
Stay updated, free articles. Join our Telegram channel

Full access? Get Clinical Tree
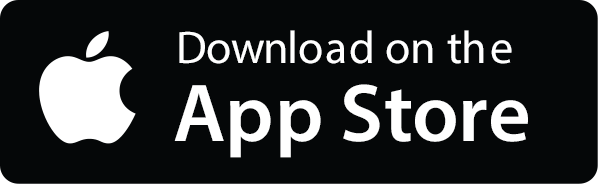
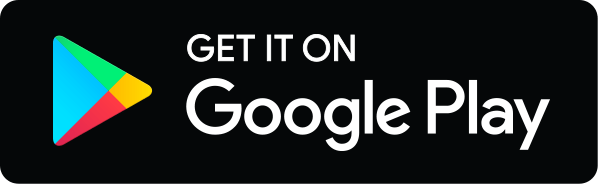