Abstracts
Catheter ablation can be accomplished with radiofrequency current, cryoablation, direct current shock, and intravascular ethanol. The complications of catheter ablation are related to the target arrhythmia, the anatomy of the heart and vessels that are entered or traversed, the energy source used for ablation, and the size and number of catheters used. In addition, the anatomic approach to the heart is associated with specific complications, such as those unique to transpericardial access. This chapter reviews the common complications of catheter ablation and their management.
Keywords
atrial fibrillation, catheter ablation, complications, pericardial tamponade, ventricular tachycardia
Key Points
- •
Complications can occur in any aspect of catheter ablation procedures.
- •
Common and specific risks and complications of catheter ablation procedures are well established.
- •
New risks and complications associated with catheter ablation of new target cardiac tachyarrhythmias such as atrial fibrillation and epicardial ventricular tachycardias are still being recognized.
- •
Extracardiac complications can also occur as collateral damage during or after catheter ablation of atrial fibrillation and epicardial catheter ablation.
- •
The presence of structural heart disease, multiple ablation targets, and a low left ventricular ejection fraction may increase the risk of complications.
- •
Electrophysiologists who attempt catheter ablation should understand the potential complications associated with this technique, how to minimize their occurrence, and how to rapidly recognize and treat the complications they encounter.
- •
If these complications are managed appropriately, the outcome is usually excellent.
Introduction
Catheter ablation has played an increasingly important role in the management of cardiac tachyarrhythmias. As the mechanisms of cardiac tachyarrhythmias have become better understood, and new techniques and technology of catheter ablation have been developed, the targets of catheter ablation have increasingly extended to more types of cardiac tachyarrhythmias. As these techniques have developed, common and specific risks and complications of catheter ablation procedures treating cardiac tachyarrhythmias have become well established. In addition to these well recognized risks, novel complications of catheter ablation continue to be reported especially as ablation is applied to new target cardiac tachyarrhythmias. The risk of a specific complication from catheter ablation differs when applied to different cardiac tachyarrhythmias, and depends on the experience and skill of both the operator and the institution. The presence of structural heart disease, multiple ablation targets, and a low left ventricular ejection fraction may increase the risk of complications. As the techniques of catheter ablation become well established, and the knowledge and experience have accumulated, the incidence of the complications has decreased. However, as more centers begin to offer catheter ablation, the number of lower volume centers is likely to increase, and may be associated with a higher risk of complications. This chapter provides an update regarding the current understanding of the risks and complications that can occur during catheter ablation procedures, and discusses their prevention, recognition, and treatment.
Types and Classification of Complications
There is a variety of potential complications that may occur during or after a catheter ablation procedure. These may be classified as major and minor complications. Major complications are defined as those that result in permanent injury or death, require an interventional remedy, or prolong the duration of hospitalization. All other complications are generally referred to as minor . Periprocedural death associated with catheter ablation is very rare, but it can occur in the catheter ablation of any kind of cardiac tachyarrhythmia.
Complications can occur in any aspect of the catheter ablation procedure, including (1) placement of a peripheral intravenous catheter; (2) intravenous sedation and/or anesthesia; (3) radiation exposure from fluoroscopy; (4) vascular access; (5) intravascular and intracardiac catheter manipulation; (6) obtaining pericardial access; (7) intrapericardial catheter manipulation; (8) cardioversion; and (9) delivery of radiofrequency (RF) energy. Any specific complication that can occur in each aspect of the procedure should be well understood to prevent and immediately recognize its occurrence.
General Complications
Table 38.1 presents a list of general complications.
During Vascular Access and Catheter Manipulation |
|
|
|
|
|
|
|
|
During Energy Delivery |
|
|
|
|
Others |
|
|
Vascular Access Complications
The possible complications associated with vascular access include bleeding, hematomas, pseudoaneurysms, retroperitoneal bleeding, arteriovenous fistulae, venous thrombi, air emboli, and arterial dissections. A pneumothorax, hemothorax, and airway compression are additional complications associated with subclavian or internal jugular venous access. Among these vascular access complications, an arterial pseudoaneurysm, arteriovenous fistula, and retroperitoneal hematoma are most common with a reported incidence of 0.28% to 1%, 0.1% to 0.86%, and 0.2% to 0.5%, respectively. A study using a routine ultrasound assessment after a vascular access reported an even higher incidence (2.2%–2.9%) of these complications, suggesting that these risks may not always be detected by a routine physical examination. Vascular access complications can be minimized by proficiency and care during vascular access. A vessel puncture with ultrasound guidance may be recommended to prevent these complications. When heparin is administered for left-sided procedures, sheaths should be removed only after the activated clotting time (ACT) is less than around 170 seconds. Reversal of anticoagulation with protamine should be considered in most of these cases. Adequate hemostasis following sheath removal is essential and may be best accomplished by specialized nursing units. Low molecular weight (LMW) heparin, if used, should not be started for at least 4 to 6 hours following the sheath removal. Most hematomas can be managed conservatively. An arteriovenous fistula complicated with a 7 F or smaller sheath may close spontaneously within a year. Therefore smaller sheaths should be used to minimize the sequelae of this complication. However, arteriovenous fistulae usually cannot be closed by an ultrasound probe compression and often require a surgical repair. An arterial pseudoaneurysm is usually associated with a large hematoma and pain usually accompanied by a bruit on examination and diagnosed by an ultrasound assessment. In general, a pseudoaneurysm is the result of inadequate compression following a sheath removal, and most common when systemic anticoagulation has been used. Pseudoaneurysms can be managed by an ultrasound-guided manual compression of the neck of the pseudoaneurysm or thrombin injection into the extravascular space. The “neck” of the pseudoaneurysm is the narrow path of blood flow between the artery, through the arterial wall, and into the pseudoaneurysm cavity. The ultrasound probe can be pushed firmly against the patient’s skin to compress the neck of the pseudoaneurysm usually for about 20 minutes. During this time, the blood within the pseudoaneurysm clots. After the probe is removed, the pseudoaneurysm will usually remain clotted especially if anticoagulation has been stopped or reversed. The procedure may require intravenous analgesia if there is significant patient discomfort. Compression is less successful if the patient is obese, because there is more fatty tissue between the skin and the neck of the pseudoaneurysm. It is also less successful if the neck of the pseudoaneurysm is wider, because it is less likely to clot during the period of compression. Finally, it is also much less successful if the patient is taking aspirin, warfarin, or another anticoagulants because these agents prevent clotting of the blood within the pseudoaneurysm. Advantages of ultrasound guided compression are that this is the least invasive method of stopping arterial blood flow into a pseudoaneurysm. If this least invasive method is not successful, a covered arterial stent placement or surgical repair should be considered. Retroperitoneal bleeding often results in orthostatic hypotension and/or anemia caused by unknown bleeding. A definitive diagnosis of this complication is made by abdominal computed tomography (CT). Retroperitoneal bleeding can usually be managed by conservative treatment but may sometimes require an arterial embolization or surgical repair.
Pericardial Effusion and Cardiac Tamponade
A pericardial effusion, with or without a tamponade, is a well-documented complication even in the most experienced centers. Cardiac tamponade may complicate any ablation procedure, but the incidence is somewhat higher with atrial fibrillation (AF) ablation (0.8%–2.9%). This is because of the complexity of the procedure, the thin walled left atrium (LA), extensive ablation, and the high level of systemic anticoagulation. Because cardiac tamponade is potentially life threatening, prompt recognition and management is essential. In the vast majority of situations, if the complication is recognized and managed expeditiously, the outcome is excellent; however, a delay in the diagnosis and treatment can lead to catastrophic results. Thus all electrophysiologists who perform catheter ablation must be prepared to immediately diagnose and treat cardiac tamponade.
Mechanism of Cardiac Tamponade
The heart lies within the pericardium, a sac composed of two layers: the visceral pericardium formed by a single layer of cells which covers the epicardium, and the thicker fibrous parietal pericardium. The pericardial space between the two layers is normally filled with a small amount of fluid, which reduces the friction from the normal torsion during cardiac contraction and relaxation. Importantly, the normal pericardial space has a significantly lower pressure than any of the cardiac chambers. Thus any breach in the myocardial wall and visceral pericardium allows blood to leak into the lower pressure pericardial space and form an effusion. The accumulation of fluid elevates the pressure within the pericardial space and impedes ventricular filling. A small amount of pericardial fluid can cause a cardiac tamponade if the pericardium is less compliant, whereas a large amount of pericardial fluid is necessary to cause tamponade when the compliance of the pericardial space is greater.
Other factors may affect the hemodynamics including the rate and volume of pericardial fluid accumulation. The occurrence and time course of cardiac tamponade therefore will vary, with tamponade physiology occurring acutely during the procedure, particularly when the area of injury is large or when a high-pressure chamber is perforated. Alternatively, some cases of cardiac tamponade develop as a slower accumulation of fluid over time and are only recognizable several hours after the procedure.
Potential Risk of a Cardiac Perforation Relevant to the Cardiac Anatomy
Cardiac tamponade is most commonly caused by a cardiac perforation occurring during catheter manipulation. Therefore it is important to understand the potential risks of a cardiac perforation relevant to the cardiac anatomy to recognize and treat, or perhaps avoid, cardiac tamponade. With respect to standard electrophysiologic procedures, the right ventricular apex is a particularly thin area of the heart rendering it prone to perforation. When placing a catheter in the right ventricle (RV) for the purpose of an electrophysiology (EP) study, it may be preferable to position the catheter in the middle of the RV near the septum, thereby avoiding the apex. The RV outflow tract is also a relatively thin area, particularly in the free wall. Care should be taken when placing catheters in this region for mapping and ablation of outflow tract tachycardias. It may be advisable to use a 4-mm-tip, nonirrigated ablation catheter in this region rather than a larger tip or externally irrigated catheter where the risk of a steam pop during RF energy delivery is higher.
The transseptal puncture is one of the most common causes of tamponade. If the transseptal needle is inadvertently exposed in the superior vena cava or right atrium, perforation can occur. If the tip of the shaped transseptal sheath is directed anteriorly while pulling it from the superior vena cava down to the fossa ovalis, it may perforate the right atrial appendage. Once the transseptal sheath is well engaged in the fossa ovalis, the potential for peril still exists. A puncture with the needle directed too posteriorly can result in a direct perforation of the posterior LA, whereas directing the needle too anteriorly can result in injury to the base of the LA appendage or root of the aorta. The damage to the base of the LA appendage usually results in tearing of this structure and often requires prompt surgical intervention.
Manipulation of catheters within the LA can also give rise to a cardiac perforation. As the ablation technology has advanced, the sheath and catheter sizes have increased. This equipment is more rigid and less forgiving when manipulating it within the heart. If any catheter or sheath is located within the LA appendage and clockwise torque is applied, there is a risk of tearing the base of the LA appendage. Likewise, there is often an invagination in the roof of the atrium that can be subject to similar trauma with manipulation of equipment within the superior aspect of the LA ( Fig. 38.1 ).

RF ablation is another potential cause for a cardiac perforation. Lesions associated with overheating with the development of steam pops are often extensive and may lead to myocardial rupture. Because multiple factors influence lesion formation, no set of ablation parameters can be considered absolutely safe. Nevertheless, limiting the power to less than 30 W and avoiding higher power applications with 8-mm-tip electrodes or irrigated electrodes minimizes the risk of steam pops. Intracardiac echocardiography (ICE) may be used to recognize overheating with the development of steam bubbles during nonirrigated RF applications. However, the value of ICE monitoring during open-irrigation RF applications is more challenging because the irrigated saline may obscure steam bubble detection.
Detection of a Pericardial Effusion and Cardiac Tamponade
A pericardial effusion may be suspected to occur during the procedure if there is rapid unexpected movement of a catheter that can puncture or tear the heart or if a catheter travels to a location not felt to be endocardial. Often, however, a pericardial effusion is not suspected until it has progressed to cardiac tamponade and the patient experiences a decrease in the blood pressure. Decreases in the blood pressure are not uncommon in the EP laboratory and are most often attributable to the dosing of medications for sedation or induced arrhythmias. It is important for the electrophysiologist to maintain a dialogue with those tasked with administering sedation. In the absence of such communication, administration of drugs to support the blood pressure can lead to a delay in the diagnosis of a cardiac tamponade as the underlying etiology of hypotension. When cardiac tamponade presents later following the procedure, patients often complain of a diffuse chest discomfort and shortness of breath. The chest discomfort is often similar in character to the pericardial pain some patients feel with ablation, but it is of greater intensity. If these symptoms arise, a prompt evaluation is critical. Although in these patients the effusion collects more gradually, the end hemodynamic effect is the same. Sinus tachycardia is known to be a common clinical sign of a cardiac tamponade that gradually develops. On the other hand, sinus bradycardia may be caused by a vagal reflex if cardiac tamponade rapidly develops. The absence of tachycardia thus does not exclude cardiac tamponade. Likewise, patients with sinus node dysfunction or who are pacemaker-dependent will not manifest sinus tachycardia.
An early diagnosis is essential to a successful management of this condition and should be made, whenever possible, in the EP laboratory so that treatment can be delivered urgently. It is preferable to recognize a developing pericardial effusion before the progression to a cardiac tamponade. The symptoms of a tachycardia, tachypnea, and hypotension are findings after the tamponade physiology is present. There are a number of techniques to document a pericardial effusion and tamponade, and fortunately several are readily available in the EP laboratory. The first detectable sign of a significant pericardial effusion is the decrease in the motion of the left heart border in the left anterior oblique (LAO) projection ( ). This finding is because the thin layer of pericardial fluid and the negative pericardial pressure usually present in the pericardial space draws the parietal pericardium inward with the visceral pericardium during systole, leading to the normal fluoroscopic excursion. This surface tension effect is similar to two glass slides with a layer of water between them. When fluid accumulates and the intrapericardial pressure becomes positive, the parietal pericardium is no longer pulled in the same direction as the visceral pericardium during systole. The decreased excursion followed by the absence of excursion usually precedes a decrease in the arterial blood pressure. Thus the operator should always note the motion of the left heart border before placement of intracardiac catheters and examine this periodically throughout the procedure. Routine fluoroscopic monitoring of the left heart border allows for the detection of a pericardial effusion before it progresses to tamponade physiology. We have found it best to have a baseline LAO cine image at the onset of the procedure to serve as a reference for a comparison during the procedure. If an effusion is detected by this method, then immediate ultrasound imaging and preparation for a pericardial drainage in the EP laboratory should be initiated.
ICE is another useful tool for a rapid diagnosis of a pericardial effusion. When using ICE, it is preferable to obtain views from the right atrium and ventricle to evaluate the presence of a pericardial effusion at baseline. Any significant accumulation of fluid within the pericardial space during the procedure is abnormal and should lead to the reevaluation of the patient’s hemodynamic stability.
Transthoracic echocardiography is a very valuable tool for the diagnosis and management of cardiac tamponade. Reliance on transthoracic echocardiography should not, however, lead to an unnecessary delay in the diagnosis or therapy. Because the time delay to obtain a standard echocardiogram is often unacceptable, all EP laboratories should have a portable ultrasound machine immediately available to the operator. Right ventricular diastolic collapse is diagnostic of cardiac tamponade. Earlier signs of an increased intrapericardial pressure include a plethora of the inferior vena cava and right atrial collapse.
If there still exists a question as to whether an effusion is hemodynamically significant, then right heart catheterization remains the gold standard. This is often easily accomplished as venous access is typically already present in patients undergoing electrophysiologic procedures. Elevation and equalization of the central venous, right ventricular end diastolic and pulmonary capillary wedge pressures as well as an attenuation in the Y descent of the right atrial pressure waveform are the hallmark findings of tamponade.
Specific Considerations
If a perforation is a focal lesion in a low-pressure chamber such as the superior vena cava or right atrium during the transseptal puncture, then conservative management with termination of the procedure and avoidance or reversal of systemic anticoagulation may be all that is required. However, a tear in the heart or great vessels necessitates urgent surgical repair.
Patients with prior cardiac surgery that have previously had their pericardium opened are at a greatly reduced risk for cardiac tamponade. Once the pericardium has been opened, the layers of the pericardium tend to form dense adhesions and the potential space between the visceral and parietal pericardium is eliminated. Although this decreases the risk of a cardiac tamponade, it does not eliminate the potential for a perforation.
Management of Cardiac Tamponade
A flow diagram of the management of a cardiac tamponade is shown in Fig. 38.2 . The first step in the management of a cardiac tamponade is blood pressure support with volume and, if necessary, pressors. An intravenous bolus injection of atropine may help to improve the hemodynamics by blocking increased vagal tone. The accumulation of fluid within the pericardial space depends on the pressure gradient between the pericardial space and perforated structure. Rapid elevation in the blood pressure should be avoided, especially if the source of the bleeding is from the left ventricle (LV). Maintenance of a relatively low normal blood pressure is desirable to minimize this gradient. Anticoagulation should be reversed. If the patient has been administered heparin, then this can be treated with protamine (1.5 mg per 1000 units of heparin given, maximum dose 50 mg, typically after a 1 mg test dose). Likewise, if the international normalized ratio (INR) is elevated, this can be treated with vitamin K, fresh frozen plasma, and recombinant factor VII. Currently, for those patients treated with new oral anticoagulants, a specific reversal agent (idarucizumab) is available for dabigatran, a direct thrombin inhibitor, and usually results in prompt normalization of coagulation. The factor Xa inhibitors, such as apixaban, edoxaban, and rivaroxaban, can be reversed with andexanet, although this antidote has not received U.S. Food and Drug Administration approval at this time. The patient should be typed and crossmatched for blood as well. These interventions should take place simultaneously with preparation for pericardiocentesis.

The subxiphoid technique described by Sosa and colleagues is a preferred method of pericardial access. A pericardiocentesis kit should be located in the procedure room so that it is immediately available. When performing an urgent pericardiocentesis in this situation, because both the pericardial space and cardiac chambers are filled with blood, aspiration of the blood does not establish the location of the needle tip. The exact location of the pericardiocentesis needle may be easily confirmed by a contrast injection into the pericardial space on fluoroscopy and/or the presence of bubbles in the pericardial effusion by echocardiography. When passing the guidewire through the needle, its location within the pericardial space must be confirmed. Typically, this is done by observing the wire travel along the left heart border in the LAO projection. A pericardial drain with multiple side holes for aspiration of fluid should be placed over the guidewire.
In certain situations, such as an obese patient, a subxiphoid pericardiocentesis may be impossible. Another option that is available is an intercostal puncture. This is easiest to accomplish if there is a clear anterior effusion, which can be located with transthoracic echocardiography. The pericardiocentesis needle is directed between the ribs for the collection of fluid under echocardiogram guidance. Similar to a thoracentesis, the needle is inserted near the superior aspect of the rib to avoid damage to the intercostal nerve, artery, and vein, which travel along the inferior margin of the rib. If this condition does not exist and the patient is hemodynamically unstable, then transcardiac decompression may be performed. This is accomplished by deliberately perforating a cardiac chamber to gain access to the pericardial space.
Once access to the pericardial space is attained, the fluid should be evacuated. For low-volume effusions, this fluid can be discarded. If blood continues to accumulate in the pericardial space, the patient can be autotransfused. This is best done with an autologous blood recovery system, as a direct autotransfusion can result in a systemic inflammatory response that can result in disseminated intravascular coagulation. An allogenic blood transfusion may involve risks including transfusion reactions. A surgical repair will be required in the event the bleeding fails to stop.
Once fluid is evacuated from the pericardial space, the hypotension should resolve immediately. It is our practice to accurately measure the volume of blood aspirated every minute following initial evacuation of the effusion with pericardiocentesis. If the rate of bleeding does not decrease within the first 15 minutes, it is likely that a surgical repair will be required. If hypotension persists, other etiologies should be explored. Medications used to support the blood pressure can typically be discontinued. Patients may have a significant amount of chest discomfort because of inflammation and irritation of the pericardium. It is well treated with intrapericardial steroids (triamcinolone 20 mg). It may also be necessary to treat the patient with intravenous narcotics as well. The presence of pericardial pain is a useful indicator that the effusion has not recurred. If the patient is stable and bleeding seems to have stopped, the patient is monitored with a portable echocardiogram every 30 minutes for 2 hours and then every 4 hours. The pericardial drain is left in place for 12 to 24 hours after no further drainage is observed and removed if there is no sign of significant reaccumulation of the effusion.
For patients who continue to have active bleeding into the pericardial space, surgical management is required. Patients managed in this fashion, avoiding prolonged hypotension, have excellent long-term outcomes. If cardiac tamponade occurs during catheter ablation to treat AF, surgical repair can be combined with an epicardial MAZE procedure with or without exclusion of the LA appendage.
When a cardiac perforation occurs in the LV, the systemic blood pressure drops immediately, resulting in cardiogenic shock. In such a situation, percutaneous cardiopulmonary support should be deployed before a pericardiocentesis.
Thromboembolism
A thromboembolism is one of the most serious complications resulting from catheter ablation procedures, although its risk for most cardiac arrhythmias has been small. The risk of thromboembolism is overall 0.6%, but it is higher in left-sided procedures (1.8%–2.0%). Thrombi can form on intravascular sheaths, RF lesions can be intrinsically thrombogenic, and preexisting thrombi can be dislodged. Most events occur within 24 hours of ablation with a high-risk period extending to 2 weeks following ablation. Although cerebral thromboembolism is most commonly detected clinically, emboli can also involve the pulmonary artery, coronary artery, or abdominal or peripheral vascular circulation. A cerebral embolism can be managed conservatively or with thrombolytic therapy or percutaneous intervention. A thromboembolism associated with catheter ablation procedures can be prevented by appropriate anticoagulation, appropriate settings of RF energy delivery, the use of an external irrigation catheter, and frequent or continuous irrigation with heparinized saline through the sheaths. Anticoagulation should be mandatory during any left-sided procedure, and it may be recommended during right-sided procedures in cases with a patent foramen ovale. In general, intravenous heparin is administered at 60 units/kg as an initial dose and added with 12 units per kg per hour continuously or a bolus of 500 to 2000 units to maintain an ACT of 250 to 300 seconds throughout the procedure. The ACT should be maintained at more than 300 seconds when a basket catheter is used, and the cases are at high risk for a thromboembolism, and at 350 to 400 seconds during LA ablation procedures for AF and flutter.
Catheter ablation of AF and atrial flutter (AFL) may potentially be the most thromboembolic. Therefore the patients with AF and AFL should be anticoagulated throughout the periablation period. Most operators systemically anticoagulate patients for a period of at least 30 days before and 6 to 8 weeks after the procedure. Two approaches can be used in the periablation period: warfarin or new oral anticoagulants such as dabigatran, apixaban, and rivaroxaban, can be continued throughout this period without interruption; or warfarin and the new oral anticoagulants may be stopped 3 days before the procedure with subcutaneous LMW heparin (1 mg/kg) used as a bridge. With the latter approach, warfarin is reinitiated the night of the ablation, and LMW heparin is started 6 hours following the sheath removal (0.5 mg/kg) and continued for 2 to 3 days. With either approach, warfarin should be continued for at least 6 to 8 weeks following the ablation.
Currently, it may be considered more preferable in most experienced centers to continue oral anticoagulation throughout the periprocedural period and to perform catheter ablation with a target INR of 2.0 to 3.5 on the day of the procedure. If the INR is above 3.5 on the day of the procedure, vitamin K can be administered to reduce the INR to a target range. Such a strategy has been shown to be associated with a very low risk of systemic emboli and a lower risk of vascular access bleeding complications than with the use of LMW heparin bridging. In addition to the lower risk of groin complications, this strategy is less costly and more convenient for both the patient and the operator. Preferably all patients (with the possible exception of those with paroxysmal AF and a CHADS 2 score of 0) should undergo transesophageal echocardiography on the day of the procedure to exclude any LA thrombus.
A thrombus formation at the tip of the ablation catheter during the RF energy delivery is known to be related to a rise in the impedance. When an impedance rise of greater than 10 to 20 Ω is observed, the RF energy delivery should be terminated, and the tip of the ablation catheter should be inspected. Thrombus formation is also related to a rise in the temperature of the tip of the ablation catheter. Therefore an aggressive setting of the temperature and RF energy should be avoided. It may be helpful for preventing thromboembolism to observe for bubble formation during the RF energy delivery, which may suggest a risk of a thromboembolism, by the use of ICE.
Air Embolism
Air can be introduced into a long sheath through infusion lines or by suction when catheters are removed. A larger diameter sheath is associated with the higher risk of an air embolism. A cerebral air embolism can result in an altered mental status, seizures, or focal neurologic signs. Magnetic resonance imaging (MRI) or CT may reveal serpiginous hypodensities in the cerebral vasculature. A coronary air embolism typically involves the right coronary artery and can present as acute inferior ischemia, ventricular fibrillation, and/or heart block. The air in the coronary artery will usually disappear soon, but it can be flushed out by a contrast injection. The pooled air should be aspirated through the sheath if possible. A massive air embolism can cause shock, and treatment of the shock should be initiated first. It is especially important to suspect and recognize a cerebral air embolism and to initiate prompt treatment. The patient should be placed in the Trendelenburg position and be administered intravenous fluids and supplemental oxygen. Hyperbaric oxygen and heparin could also be considered. To prevent an air embolism, all infusion lines should be monitored for bubbles, all sheaths must have side-holes, and catheter withdrawal should be slow and always followed by aspiration of the sheath. It is especially important to aspirate and flush long sheaths in the LA on initial entry (i.e., after removal of needle and dilator) and before inserting a new catheter after one has been removed.
Atrioventricular Block
Inadvertent atrioventricular (AV) block can occur during catheter ablation of AV nodal reentrant tachycardia, septal accessory pathways, and even ventricular tachycardias arising from the ventricular septum with an incidence of 1% to 3%. The risk of AV block is reported to be the highest in cases with a midseptal accessory pathway.
To reduce the likelihood of inadvertent AV block, RF energy should not be applied near the compact AV node. The His bundle is less likely to be damaged than either the AV node or right bundle branch. His bundle and coronary sinus catheters are helpful as landmarks for understanding the Koch’s triangle. Looking at both the LAO and right anterior oblique (RAO) projections is important to understand the distance between the His bundle catheter and ablation catheter. Anatomically, the compact AV node is located inferior and slightly posterior to the His bundle. Therefore an RF energy application may be safer on the ventricular side as noted by a large ventricular and a very small atrial electrogram when it is delivered below the His bundle region. AV block has been reported even when the RF energy was delivered at the level of the coronary sinus. It is critical to monitor AV conduction during RF energy delivery. If AV block or PR interval prolongation occurs, RF energy application should be terminated immediately. If a junctional rhythm develops during an RF energy application, the ventriculoatrial (VA) conduction should be monitored. The development of VA block during the junctional rhythm may suggest damage to the fast AV nodal pathway and should prompt immediate termination of the application. When junctional rhythm develops during an RF energy application, deployment of rapid atrial pacing faster than the junctional rhythm may be an alternative to assure that the conduction is intact via the fast AV nodal pathway. When a patient has a hemi-bundle branch block at baseline, catheter ablation around the other bundle branch may result in AV block. Therefore in such a case, the risk of inadvertent AV block should be considered even when a peripheral catheter ablation away from the AV node and His bundle is performed. Back up ventricular pacing should be prepared in cases when a risk of inadvertent AV block is expected. The incidence of inadvertent AV block during catheter ablation of a cavotricuspid isthmus dependent AFL has been reported. This is usually the result of ablation more septal than is desired. Thus the cavotricuspid isthmus should be initially approached at its midpoint (6 o’clock in the LAO view) safely away from the normal conduction system. However, if too much clockwise torque is given to the ablation catheter to keep it on the septal side of the cavotricuspid isthmus, the ablation catheter may flip up to the site of the normal conduction system, resulting in inadvertent AV block. This potential risk should be understood during the AFL ablation. Late inadvertent AV block can occur, which likely results from expansion of the RF lesions, development of edema, and local ischemia. When transient inadvertent AV block is observed during catheter ablation, the patient should be monitored overnight. Patients with late AV block may develop syncope, acute heart failure, and fatigue.
Cryoablation may be an alternative to RF ablation during catheter ablation around the AV node and His bundle region. Ice mapping by cooling the tissue to mild temperatures (0 to –10°C) allows testing the results of a potential ablation site before a permanent lesion is created. Even if inadvertent AV block occurs during ice mapping, it should be reversible. A lesion expansion after the application is less likely to occur with cryoablation than with RF ablation. Cryoablation may more often be used in pediatric patients. Despite these potential safety advantages, cryoablation seems to produce less durable efficacy for AV nodal reentrant tachycardia or AFL than RF energy.
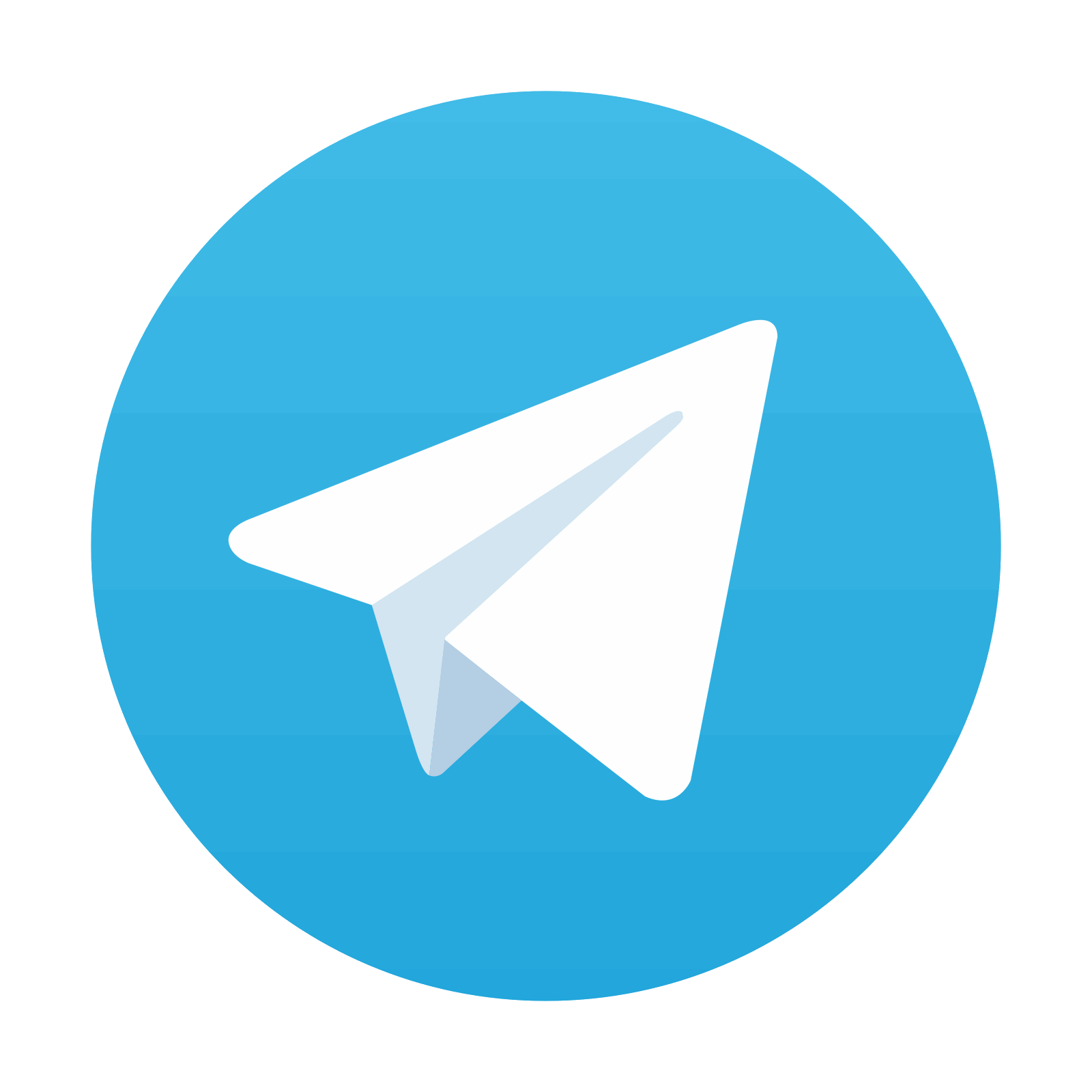
Stay updated, free articles. Join our Telegram channel

Full access? Get Clinical Tree
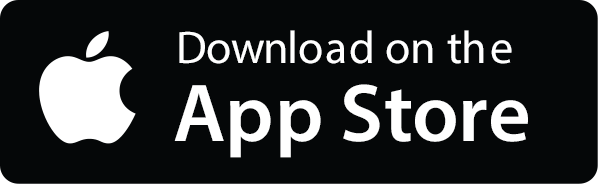
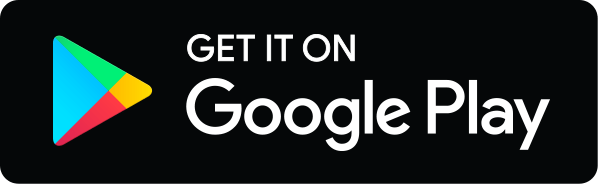