The specific mechanisms by which diabetes may affect the myocardial tissue response to ischemia are unclear. Our objective was to prospectively quantify the degree of myocardial edema in diabetics versus nondiabetics with ST elevation myocardial infarction using cardiac magnetic resonance. Fifty-two patients (16 diabetics and 36 nondiabetics) were enrolled after primary percutaneous coronary intervention and underwent cardiac magnetic resonance on a 1.5-T scanner at 48 hours and 6 months. Myocardial edema was quantified using a T2 mapping technique, and infarct size and microvascular obstruction size were assessed by way of a contrast-enhanced T1-weighted inversion recovery gradient-echo sequence. The infarct segment T2 was elevated in diabetics compared with nondiabetics (59.0 ± 8.0 vs 50.8 ± 3.1 ms, p <0.001) at 48 hours. Multivariate analysis demonstrated that diabetes (p <0.001) and symptom-to-balloon time (p = 0.04) were independent predictors of the degree of acute myocardial edema. Infarct size was nonsignificantly higher in the diabetic group at 48 hours (26.9 ± 9.4% vs 20.1 ± 10.1% of myocardium, p = 0.07) and 6 months (17.1 ± 6.3% vs 13.4 ± 6.1% of myocardium, p = 0.09). Microvascular obstruction size was equivalent in both groups, and there was a trend toward lower myocardial salvage index in diabetics (34.2 ± 11.8 vs 49.6 ± 13.4, p = 0.08). In conclusion, diabetes is associated with increased myocardial edema in the acute phase after primary percutaneous coronary intervention. Our results offer insight into the complex processes that characterize myocardial tissue response to injury in diabetic patients.
Edema constitutes one of the many components of myocardial tissue response to injury. Immediately after a coronary occlusion, the ischemic area at risk is characterized by substantial interstitial and intracellular edema, which may be further increased by reperfusion. The presence of myocardial edema after a non–ST-segment elevation acute coronary syndrome has been recently shown to be associated with a greater hazard of cardiovascular event or death within 6 months. Furthermore, quantification of myocardial edema using cardiac magnetic resonance (CMR) has been validated in animal models and humans using T2-based imaging techniques. The objective of our study was to quantitatively evaluate the relation between diabetes and myocardial edema using CMR in patients with ST elevation myocardial infarction (STEMI) after primary percutaneous coronary intervention (PCI).
Methods
Patients presenting to Sunnybrook Health Sciences Centre in Toronto, with STEMI from July 2009 to October 2011 were screened and prospectively enrolled. The main inclusion criterion was that patients should meet the standard diagnostic criteria for STEMI. All patients had undergone primary PCI as part of a regional program that triages patients with STEMI to our center for early revascularization. Exclusion criteria included hemodynamic instability (defined as systolic blood pressure <90 mm Hg and use of inotropic agents or an intra-aortic balloon pump), significant arrhythmias, significant renal dysfunction (estimated glomerular filtration rate <30 ml/min), and typical contraindications to CMR (e.g., pacemaker). We obtained written consent from all patients. The study was approved by the ethics review board of Sunnybrook Health Sciences Centre.
Patients were divided into 2 groups: diabetic versus nondiabetic. Diabetic patients were identified based on a confirmed preexisting diagnosis or a new diagnosis that fulfills current guidelines’ criteria including (1) hemoglobin A 1c ≥6.5% or (2) fasting plasma glucose ≥7.0 mmol/L.
All patients were pretreated before revascularization with aspirin 162 mg and clopidogrel 600 mg. Choice of anticoagulant (intravenous heparin or bivalirudin) and optional use of glycoprotein IIb/IIIa inhibitor were left to operator’s discretion. Thrombus aspiration was performed with an Export device (Medtronic Inc., Minneapolis, Minnesota). Subsequently patients received aspirin, clopidogrel, β blockers, angiotensin-converting enzyme inhibitors, angiotensin receptor blockers, and statins as per standard of care. Thrombolysis In Myocardial Infarction flow grade, myocardial blush grade, thrombus score, and ST-segment resolution were recorded by 2 blinded observers. Patients with a preexisting diagnosis of hypertension (>130/80 mm Hg for diabetics, >140/90 mm Hg for nondiabetics) or dyslipidemia (low-density lipoprotein >2.0 mmol/L) were classified as such.
Each patient underwent imaging within 48 hours after STEMI and at 6 months. Studies were performed on a 1.5-T clinical scanner (Signa TwinSpeed HDx; GE Healthcare, Waukesha, Wisconsin) with commercially available CMR software, electrocardiographic triggering, 8-channel receive coil and breath holds at end-expiration. After performing a localizer scan, short-axis cine CMR (10 to 12 slices) was performed with a balanced steady-state free-precession sequence (FIESTA, GE Healthcare) as described previously. Myocardial edema was assessed by T2 quantification using a previously validated free-breathing cardiac-gated spiral imaging sequence with T2 preparation, contiguous slices from base to apex, and the following typical parameters: echo times = 2.9, 24.3, 88.2, and 184.2 ms, 12 spiral interleaves with 4,096 points each (16.4-ms duration), slice thickness = 6 mm, and bandwidth = 125 kHz. Myocardial hemorrhage was assessed by T2* quantification using a breath hold, cardiac-gated, spoiled gradient-echo sequence with multiple echoes as described previosuly. A breath hold, contrast-enhanced, T1-weighted inversion recovery gradient-echo sequence was used to depict the presence, location, and extent of myocardial infarction and microvascular obstruction (MVO) as described previously.
All cine CMR images were analyzed using QMass MR software (Medis, Leiden, Netherlands), and the results were the consensus of 2 experienced blinded observers. Endocardial and epicardial borders were delineated on the end-diastolic and end-systolic phases of short-axis slices for quantification of left ventricular volumes, function, mass, and infarct segment systolic wall thickening. Measurements were indexed for body surface area. End-diastolic wall thickness was measured in the infarct segment as an indicator of myocardial edema in the acute phase and wall thinning in the chronic phase. Myocardial T2 and T2* maps were constructed by fitting signal intensities at each pixel with an exponential model for the given echo times. Hemorrhage was identified as an area of hypointensity on T2* images within the infarcted segment. Infarct size was quantified in a contrast-enhanced T1-weighted image using the full-width half-maximum technique as previously described, and MVO was identified as a region of hypoenhancement within the region of the hyperenhanced infarct, 10 minutes after contrast administration. Infarct, hemorrhage, and MVO sizes are expressed as a percentage of total myocardium. Myocardial salvage index (MSI) was quantified as the difference between the volume of increased T2 signal (area at risk) and infarct size as previously described. Data fitting for T2 and T2* maps and size computation for infarct, hemorrhage, and MVO were performed with custom-written scripts developed in MATLAB (MathWorks, Natick, Massachusetts). MVO was also recorded as a binary variable of being present or absent, based on the contrast-enhanced images.
We excluded patients with hemorrhage from our primary analysis given that T2 quantification is hindered by the competing effects of edema and hemorrhage in these patients. Study end points were the degree of myocardial edema (T2 value and end-diastolic wall thickness measurements), infarct size, and MVO size 48 hours and 6 months after STEMI in patients with and without diabetes.
We also performed a sensitivity analysis comprising all patients, including the ones with hemorrhage, and assessed their infarct, hemorrhage, and MVO sizes. Comparison of edema was not done in this analysis given the confounding susceptibility effects of hemorrhage.
Statistical analyses were carried out using SAS, version 9.0 (SAS Institute, Cary, North Carolina). Continuous data are expressed as mean ± SD and were analyzed by the Student t test. Categorical variables are reported as frequencies and percentages and were analyzed by Fisher’s exact test, as appropriate. Univariate linear regression analysis was utilized to determine the association of variables with degree of myocardial edema (infarct segment T2 measurement) at 48 hours. Variables with a p value <0.25 were then entered into a multivariate model. Multivariate regression was performed using a Cox proportional hazards analysis. For the multiple regression predictions, continuous variables were log transformed to control dispersion. All tests were 2-tailed and statistical significance was accepted at p <0.05.
Results
Ninety-nine patients with STEMI were screened, 31 patients were excluded, and 68 patients underwent CMR. Reasons for exclusion included severe renal insufficiency, claustrophobia, hemodynamic instability, and presence of permanent pacemaker. Patients were stratified based on diabetes status. Sixteen patients had a hypointense core within the infarcted segment on T2* imaging indicative of hemorrhage and were therefore excluded from the primary analysis. Fifty-two patients (16 diabetics and 36 nondiabetics) remained in the primary analysis and their clinical characteristics are listed in Table 1 . Of the 16 diabetic patients, 15 (94%) had type 2 diabetes, 4 (25%) used insulin as part of their medical regimen, whereas 12 patients (75%) were being treated with oral hypoglycemic agents only. Two of the 16 diabetic patients had newly diagnosed diabetes. Periprocedural characteristics for both groups are listed in Table 2 . The median symptom-to-balloon time was 260 minutes (interquartile range 140 to 464) in diabetic patients and 268 minutes (interquartile range 134 to 480) in nondiabetic patients (p = 0.79). The median door-to-balloon time was 72 minutes (interquartile range 52 to 94) in diabetic patients and 68 minutes (interquartile range 51 to 100) in nondiabetic patients (p = 0.71). The median time from primary PCI to CMR was 35 hours (interquartile range 30 to 44) in diabetic patients and 36 hours (interquartile range 29 to 46) in nondiabetic patients (p = 0.42).
Variable | Diabetes Mellitus | |
---|---|---|
Yes (n = 16) | No (n = 36) | |
Age (yrs) | 59.4 ± 7.1 | 59.7 ± 10.6 |
Men | 14 (88) | 32 (89) |
Hypertension | 7 (44) | 16 (44) |
Dyslipidemia | 5 (31) | 8 (22) |
Smoker | 5 (31) | 10 (28) |
Previous myocardial infarction | 1 (6) | 1 (3) |
Previous PCI | 1 (6) | 3 (8) |
Mean admission fasting glucose level (mmol/L) | 9.1 ± 1.9 | 5.5 ± 1.7 |
Mean hemoglobin A 1c level (%) | 8.2 ± 1.5 | 5.1 ± 1.1 |
Mean creatinine level (μmol/L) | 82.1 ± 16.8 | 83.9 ± 21.3 |
Variable | Diabetic (n = 16) | Nondiabetic (n = 36) |
---|---|---|
Infarct-related coronary artery | ||
Left anterior descending | 7 (44) | 14 (39) |
Left circumflex | 4 (25) | 5 (14) |
Right | 5 (31) | 17 (47) |
Multivessel coronary disease | 6 (38) | 15 (42) |
Thrombus score | ||
2 | 5 (31) | 14 (39) |
3 | 7 (44) | 13 (36) |
4 | 1 (6) | 2 (6) |
Adjunctive pharmacologic therapy | ||
Heparin + glycoprotein IIb/IIIa inhibitor | 7 (44) | 18 (50) |
Bivalirudin | 9 (56) | 18 (50) |
Thrombus aspiration | 13 (81) | 30 (83) |
Stent type | ||
Bare metal | 10 (62) | 25 (69) |
Drug eluting | 6 (38) | 11 (31) |
Mean peak creatine kinase (IU/L) | 2,691 ± 1,109 ∗ | 2,011 ± 1,353 ∗ |
ST-segment resolution | 10 (63) | 24 (67) |
Post-PCI Thrombolysis In Myocardial Infarction flow grade ≥2 | 15 (94) | 35 (97) |
Post-PCI myocardial blush grade ≥2 | 11 (69) | 26 (72) |
CMR outcome measures at 48 hours and 6 months are presented in Table 3 . At 48 hours, both measures of myocardial edema, that is, infarct segment T2 (p <0.001) and infarct segment end-diastolic wall thickness, were significantly higher in the diabetic group (p = 0.01). Remote segment T2 measurements were also higher in the diabetic group, although the difference was not statistically significant (p = 0.07). Figure 1 demonstrates the myocardial T2 maps along with late gadolinium enhancement images in short-axis views from representative patients in the diabetic and nondiabetic groups. The frequency and spatial extent of MVO was equivalent in both groups. There was a trend toward a greater infarct size at 48 hours ( Table 3 ) and a lower MSI in diabetic patients versus nondiabetics (34.2 ± 11.8 vs 49.6 ± 13.4, p = 0.08).
Variable | Scan 1 (48 h) | Scan 2 (6 mo) | ||||
---|---|---|---|---|---|---|
Diabetic | Nondiabetic | p Value | Diabetic | Nondiabetic | p Value | |
Infarct segment T2 (ms) | 59.0 ± 8.0 | 50.8 ± 3.1 | <0.001 | 39.9 ± 2.9 | 40.1 ± 2.8 | 0.83 |
Remote segment T2 (ms) | 43.9 ± 1.9 | 39.2 ± 2.4 | 0.07 | 40.4 ± 2.4 | 39.3 ± 2.7 | 0.78 |
Infarct segment end-diastolic wall thickness (mm) | 11.4 ± 2.2 | 9.2 ± 1.6 | 0.01 | 4.1 ± 1.1 | 5.5 ± 1.0 | 0.02 |
Remote segment end-diastolic wall thickness (mm) | 6.8 ± 1.4 | 7.1 ± 1.3 | 0.71 | 8.3 ± 1.4 | 8.2 ± 1.2 | 0.91 |
MVO | 5 (31) | 12 (33) | 0.74 | — | — | — |
MVO (% of myocardium) | 2.2 ± 1.2 | 3.1 ± 2.1 | 0.66 | — | — | — |
Infarct size (% of myocardium) | 26.9 ± 9.4 | 20.1 ± 10.1 | 0.07 | 17.1 ± 6.3 | 13.4 ± 6.1 | 0.09 |
Left ventricular end-diastolic volume index (ml/mm 2 ) | 67.4 ± 17.3 | 69.4 ± 10.9 | 0.82 | 84.4 ± 19.4 | 76.4 ± 19.8 | 0.32 |
Left ventricular end-systolic volume index (ml/mm 2 ) | 37.2 ± 12.7 | 37.1 ± 9.3 | 0.89 | 39.9 ± 15.8 | 37.3 ± 13.4 | 0.74 |
Left ventricular stroke volume index (ml/mm 2 ) | 30.6 ± 9.1 | 32.4 ± 4.1 | 0.72 | 44.4 ± 8.7 | 39.4 ± 9.6 | 0.55 |
Left ventricular ejection fraction (%) | 44.5 ± 8.0 | 46.2 ± 5.8 | 0.61 | 47.5 ± 9.4 | 51.2 ± 9.9 | 0.26 |
Infarct segment systolic wall thickening (%) | 1.8 ± 23.8 | 2.8 ± 16.3 | 0.68 | 25.8 ± 19.8 | 60.8 ± 35.3 | 0.03 |
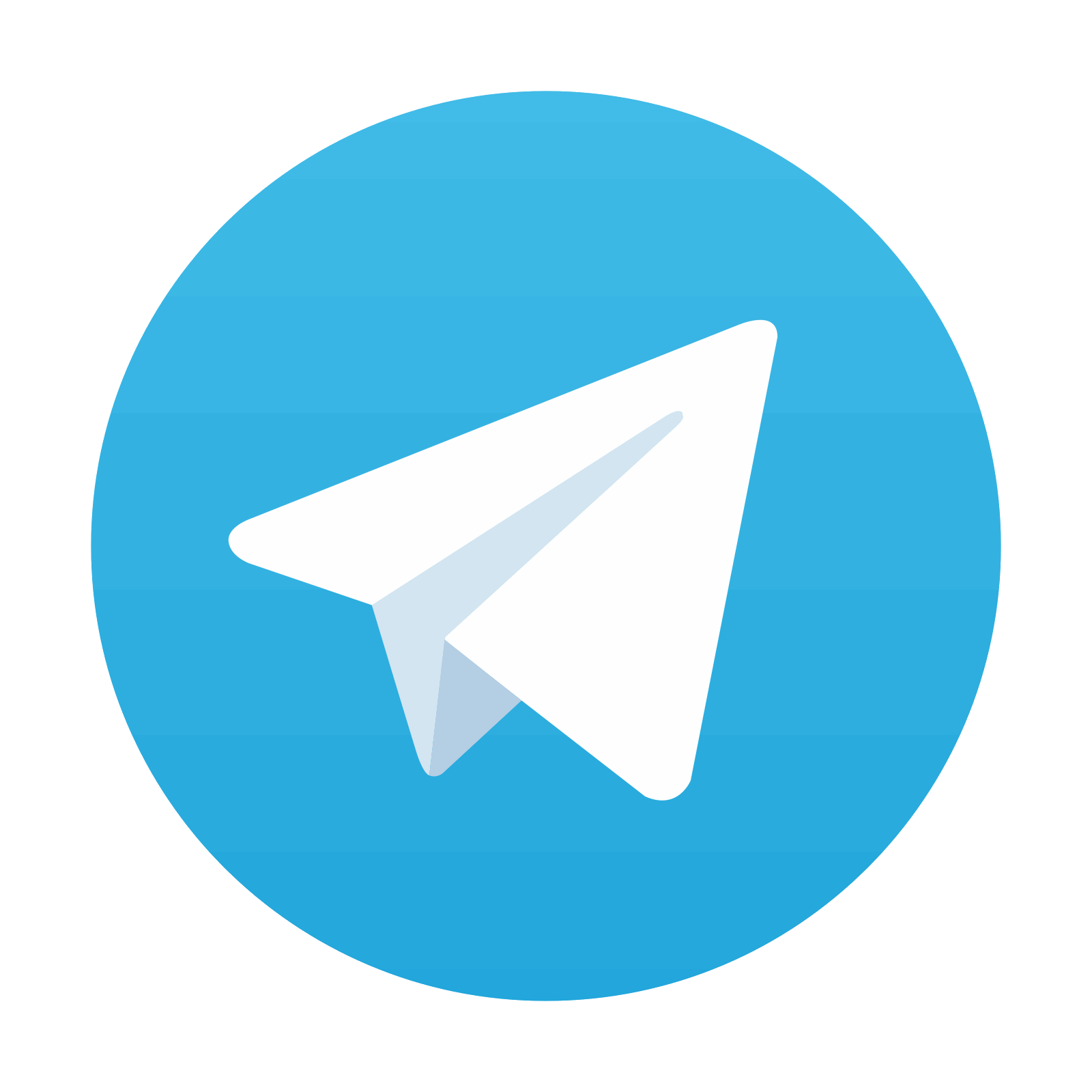
Stay updated, free articles. Join our Telegram channel

Full access? Get Clinical Tree
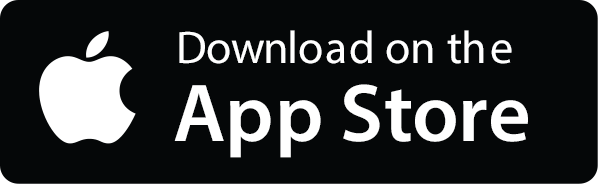
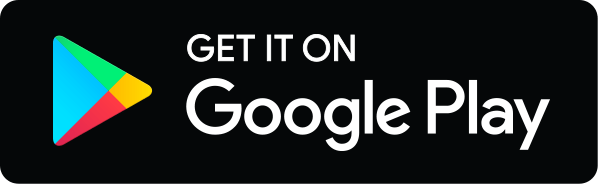
