We sought to compare the diagnostic accuracy and feasibility of coronary flow velocity reserve (CFVR) measurement using transthoracic Doppler echocardiography (TTDE) and 320-row multidetector computed tomographic coronary angiography (CTCA) for predicting in-stent restenosis (ISR). We enrolled 126 consecutive patients with 309 implanted coronary stents in the 3 major coronary arteries. TTDE and CTCA were performed within the 2-week period before follow-up invasive coronary angiography. Binary ISR was defined as percent diameter stenosis ≥50% on invasive coronary angiogram. A CFVR <2.0 using TTDE and a narrowing of ≥50% measured with CTCA were the thresholds indicating the presence of binary ISR. Presence of ISR using invasive coronary angiography was observed in 26 (8%) stents and 26 (14%) vessels. Feasibilities of CFVR measurement and CTCA for predicting ISR in the 3 major vessels were 94% and 91%, respectively. A CFVR <2.0 revealed a 95% diagnostic accuracy with sensitivity of 87%, specificity of 96%, positive predictive value of 77%, and negative predictive value of 98%. Diagnostic accuracy of CTCA was comparable to that of CFVR measurement; however, CTC angiographic results were confounded by metal artifacts in the assessment of small-diameter stents. In conclusion, noninvasive CFVR measurement has high feasibility and accuracy for predicting ISR and is comparable to 320-row CTCA.
Coronary flow velocity reserve (CFVR) measurement by transthoracic Doppler echocardiography (TTDE) and computed tomographic coronary angiography (CTCA) have the potential to provide accurate information for the diagnosis of in-stent restenosis (ISR). CFVR has recently been shown to be an accurate method of measuring significant ISR in the 3 major coronary arteries using TTDE. In contrast, CTCA has advanced in recent years as the most accurate tool for noninvasive coronary angiography. The ability of 320-slice technology to incorporate 16-cm anatomic coverage allows imaging of the entire heart in a single gantry rotation, and this improves image quality, decreases artifacts, and enables better visualization of stented coronary segments. Despite emerging evidence supporting the use of these 2 noninvasive methods in the assessment of ISR, there has been no objective comparison of the diagnostic utility of noninvasive CFVR measurement and 320-row CTCA. Therefore, we compared the diagnostic accuracy and feasibility of CFVR measurement using TTDE and 320-row CTCA for predicting ISR.
Methods
The study group began with 131 consecutive patients referred to the Yokkaichi Social Insurance Hospital from January 2009 through December 2010 for follow-up invasive coronary angiography after successful coronary stent implantation with residual diameter stenosis <50%. After excluding 4 patients with renal insufficiency (serum creatinine >2.0 mg/dl) and 1 patient with severe respiratory insufficiency, 126 patients underwent TTDE and CTCA within 2 weeks before follow-up invasive coronary angiography 9 ± 3 months after stent implantation. All patients were evaluated for the presence of coexisting traditional risk factors for coronary artery disease. Dyslipidemia was defined as low-density lipoprotein cholesterol ≥140 mg/dl, triglyceride ≥150 mg/dl, and/or high-density lipoprotein cholesterol <40 mg/dl according to Japanese Atherosclerosis Society criteria or use of antidyslipidemic medications. All medications including oral β blockers were not discontinued before TTDE and CTCA. Written informed consent was obtained from all subjects, and the protocol was approved for use by the human studies subcommittee of the Yokkaichi Social Insurance Hospital.
All patients underwent a complete routine transthoracic echocardiography with CFVR measurement using a Sonos 7500 system (Philips Medical Systems, Inc., Andover, Massachusetts). Left ventricular ejection fraction and wall thickness were measured by 2-dimensional echocardiography. Patients were defined as having left ventricular hypertrophy if interventricular septal or posterior wall thickness was ≥12 mm. A high-frequency 8.0-MHz broadband transducer for the left anterior descending coronary artery (LAD) and a 4.0-MHz broadband transducer for the left circumflex and right coronary arteries were equipped for CFVR measurement (Doppler frequency 5.0 or 3.5 MHz). Using color Doppler flow mapping, the velocity range was set at ±10.0 to ±20.0 cm/s. To measure LAD flow, the left ventricle was imaged in the low parasternal long-axis cross section, and coronary blood flow in the distal portion of the LAD was identified in the anterior interventricular sulcus using Doppler color flow mapping. Long-axis sections were then carefully adjusted to minimize the angle between the Doppler beam and the LAD flow. With a sample volume (length 1.5 mm) positioned on the color signal in the LAD in diastole, pulsed Doppler signal tracings of flow velocity were recorded. To measure left circumflex coronary artery flow, we searched Doppler flow signals at the basal to the midportion of the left ventricular lateral region in the apical 4-chamber view. Doppler spectral tracings of left circumflex coronary artery flow velocities were then recorded with a sample volume (length 1.5 mm). To measure right coronary artery flow, we selected the posterior descending coronary artery. In the apical 4-chamber view, the transducer was rotated in a counterclockwise manner until the linear color signal was clearly visualized in the posterior interventricular sulcus using Doppler color flow mapping. A sample volume (length 1.5 to 2.0 mm) was positioned on the color signal, and then CFV was recorded.
After baseline spectral Doppler signals in the 3 major coronary arteries were recorded in >5 cardiac cycles at end-expiration, adenosine triphosphate was administered intravenously (0.14 mg/kg/min) to obtain the peak flow response induced by coronary microvessel dilation. All patients underwent continuous 12-lead electrocardiographic monitoring and blood pressure recording at baseline, every minute during adenosine triphosphate infusion, and at recovery. Two experienced and blinded investigators measured CFVR by tracing the contour of the spectral Doppler signal using the computer incorporated in the ultrasound system. CFVR was defined as the ratio of mean diastolic velocity at peak flow response to mean diastolic velocity at baseline ( Figure 1 ), and a CFVR <2.0 was defined as abnormal flow reserve equivalent to having binary ISR. All measurements for calculating CFVR were averaged over 3 cardiac cycles. The mean time required to complete a CFV measurement including CFVR calculation was around 10 minutes in each coronary artery.
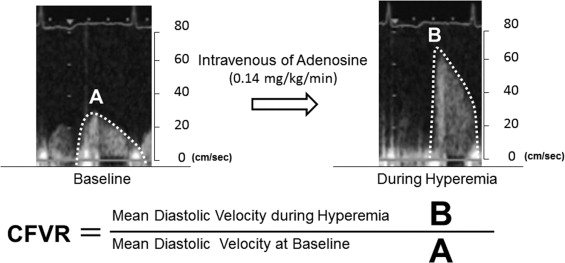
All patients received nitroglycerin 0.3 mg sublingually just before CTC angiographic scanning to increase coronary artery diameters and facilitate accurate evaluation. An additional oral β blocker (atenolol 25 to 50 mg) was given 1 hour before CTC angiographic scanning in 76 patients because their heart rates were ≥65 beats/min. Imaging was performed with a whole-heart scan on a 320-row CTC angiography with 0.5-mm detector elements, 350 ms of gantry rotation time, and up to 16 cm of coverage in the Z direction (Aquilion ONE, Toshiba Medical Systems, Otawara, Japan). During the scan, electrocardiogram was registered simultaneously for prospective triggering of data. Tube voltage was 120 kV and maximal tube current was 400 to 580 mA. Depending on a patient’s body weight and height, iodine 6 to 11 ml (iodine 350 mg/ml; Omnipaque 350, Daiichi-Sankyo, Tokyo, Japan) was injected at a 3- to 5.5-ml/s flow rate as a test bolus for determining the arrival time in the ascending aorta, followed by a 20-ml saline chaser at the same flow rate. All images were acquired during an inspiratory breath-hold of ≥5seconds. An initial dataset was reconstructed at 75% to 80% of the RR interval, with a slice thickness of 0.50 mm and a reconstruction interval of 0.25 mm. If multiple phases were obtained, additional reconstructions were explored in an effort to obtain images with the fewest motion artifacts. Two blinded and experienced investigators assessed ISR on a commercially available workstation (ZIO STATION System 1000, Amin/ZIOSOFT, Tokyo, Japan). Significant ISR was defined as a darker eccentric or concentric rim between the stent and the enhanced vessel lumen, with a lumen decrease ≥50% or the presence of significant stent edge stenosis (significant stenosis <5 mm from stent edge). In the present study, vessel-based analysis was performed for head-to-head comparisons of the utility of CFVR measurement for the diagnosis of ISR. Therefore, the entire vessel was considered to have ISR if ≥1 of multiple stents was defined as ISR, and the entire vessel was considered misdiagnosed or not assessable if ≥1 of multiple stents was not accurately diagnosed or no diagnostic image was produced.
Selective invasive coronary angiography was performed using standard techniques after right and left intracoronary administration of isosorbide dinitrate 2.5 to 5 mg. Quantitative analysis of invasive coronary angiogram (QAngio XA 7.1.14.0, Medis Medical Imaging Systems, Leiden, the Netherlands) was performed and interpreted independently by another 2 readers unaware of CFVR and CTC angiographic results. At least 2 orthogonal projections were evaluated; measurement of percent diameter stenosis was performed in the projection showing the greatest degree of narrowing. All stented segments including the 5-mm segments proximal and distal to stent edges were evaluated and binary ISR was defined as percent diameter stenosis ≥50%.
Continuous variables are presented as mean ± SD. Categorical variables are presented as percent frequency. Sensitivity, specificity, positive predictive value, and negative predictive value of detecting ISR ≥50% by CTCA and of detecting CFVR <2.0 by TTDE were compared to invasive coronary angiography using a 2 × 2 cross-tabulation model. The diagnostic performance between CTC angiographic and CFVR measurement was compared with pairwise McNemar test. Confidence intervals were calculated according to the percentile method. A p value <0.05 was accepted as statistically significant. Analyses were performed using SPSS 18 for Windows (SPSS, Inc., Chicago, Illinois).
Results
Patient characteristics are listed in Table 1 . Of 126 patients, 9 patients had chest symptoms. In total 188 vessels were treated with 309 stents. Stent characteristics are listed in Table 2 . Of the 309 stents, 90 were partially overlapping and 2 were entirely overlapping owing to stent implantation inside another stent after previous ISR. Stent diameter ranged from 2.5 to 4.0 mm, and 75% of stents were ≤3.0 mm. Binary ISR was observed on invasive coronary angiogram in 26 (8%) stents and 26 (14%) vessels (10 in the LAD, 7 in the left circumflex coronary artery, and 9 in the right coronary artery). Only 2 of 9 symptomatic patients had ISR. None of the ISR lesions were at the site of overlapping. All 26 stents with ISR were drug-eluting stents, and their mean stent diameter was 2.93 ± 0.30 mm and mean stent length was 21.5 ± 6.5 mm.
Variable | Value |
---|---|
Age (years) | 68 ± 6 |
Men | 90 (71%) |
Height (cm) | 160 ± 11 |
Weight (kg) | 62 ± 20 |
Body mass index >30 kg/m 2 | 4 (3%) |
Systolic blood pressure (mm Hg) | 137 ± 21 |
Diastolic blood pressure (mm Hg) | 78 ± 13 |
Heart rate (beats/min) | 78 ± 15 |
Hypertension | 105 (83%) |
Diabetes mellitus | 69 (55%) |
Dyslipidemia | 84 (67%) |
Current smoker | 59 (47%) |
Previous myocardial infarction | 5 (4%) |
Left ventricular ejection fraction | 63 ± 11 |
Left ventricular hypertrophy | 29 (23%) |
Number of stents | 309 |
Cell shape | |
Closed cell | 260 (84%) |
Open cell | 43 (14%) |
Semiopen cell | 6 (2%) |
Strut thickness | |
Thick strut ≥0.005 inch | 284 (92%) |
Thick strut <0.005 inch | 25 (8%) |
Stent diameter (mm) | 2.98 ± 0.40 |
2.5 | 96 (31%) |
2.75 | 3 (1%) |
3.0 | 133 (43%) |
3.5 | 68 (22%) |
4.0 | 9 (3%) |
Stent length (mm) | 21.4 ± 6.5 (8.0–33.0) |
Drug-eluting stent | 278 (90%) |
Bare-metal stent | 31 (10%) |
Diagnostic performance of CFVR measurement for prediction of significant ISR is presented in Table 3 . Inadequate spectral Doppler recordings of coronary flow were observed in 12 vessels, 3 of which vessels were diagnosed as having ISR on invasive coronary angiogram. There were 15 drug-eluting stents and 3 bare-metal stents in these 12 nonassessable vessels by CFVR measurements, and 7 of 18 stents were ≤3.0 mm in diameter. There were 150 vessels represented as CFVR ≥2.0 and 26 vessels represented as CFVR <2.0, and 20 vessels with significant ISR were correctly identified on CFVR measurements. Incidence of false-positive diagnoses by CFVR measurements was observed in 6 patients and 6 vessels. These 6 patients had coexistent hypertension, and 5 patients had multiple co-morbidities including diabetes mellitus, dyslipidemia, smoking, obesity, and left ventricular hypertrophy, which contribute to coronary microcirculatory dysfunction. Baseline heart rate was similar between patients with and those without false-positive CFVR results (64 ± 15 vs 65 ± 10 beats/min, p = NS) and had only a weak correlation with CFVR (r = −0.16, p <0.05) in all patients. Incidence of false-negative diagnoses by CFVR measurements was observed in 3 patients and 3 vessels, and these vessels had 50% to 75% stenosis quantified by invasive coronary angiography. Multivariate linear regression analysis was performed to identify clinical and angiographic variables (age, gender, baseline heart rate, coexisting risk factors for coronary artery disease, medication uses, stent characteristics, and percent diameter stenosis measured by quantitative invasive coronary angiography) that might predict CFVR with a stepwise method. Results indicated that only percent diameter stenosis was an independent determinant of CFVR with standardized coefficients of −0.47.
CFVR | CTCA | |||||||||
---|---|---|---|---|---|---|---|---|---|---|
Nonassessable Vessels | Assessable Vessels | Nonassessable Vessels | Assessable Vessels | |||||||
ISR | No ISR | TP | FP | FN | ISR | No ISR | TP | FP | FN | |
Left anterior descending coronary artery (n = 87) | 1 | 1 | 8 | 1 | 1 | 0 | 8 | 9 | 1 | 1 |
Left circumflex coronary artery (n = 39) | 2 | 3 | 4 | 2 | 1 | 3 | 1 | 3 | 4 | 1 |
Right coronary artery (n = 62) | 0 | 5 | 8 | 3 | 1 | 1 | 4 | 7 | 1 | 1 |
Inadequate CTC angiographic images were observed in 34 stents including 32 drug-eluting stents and 2 bare-metal stents and 17 vessels. In the 34 nonassessable stents, 28 stents were 2.5 mm and 6 stents were 3.0 mm in diameter, and all inadequate CTC angiographic images were due to metal artifacts. In 17 vessels nonassessable by CTCA, 4 vessels were diagnosed as having ISR on invasive coronary angiogram ( Table 3 ). There were 146 vessels represented as <50% stenosis and 25 vessels represented as ≥50% stenosis by CTCA, and 19 vessels with significant ISR were correctly identified on CTC angiogram. Incidence of false-positive diagnoses by CTCA was observed in 6 patients and 6 vessels. These stents were drug-eluting stents ≤3.0 mm in diameter. Incidence of false-negative diagnoses by CTCA was observed in 3 patients and 3 vessels.
Figures 2 to 4 show examples of invasive coronary angiogram, CTC angiogram, and CFVR in patients after stent implantation in the LAD, left circumflex coronary artery, and right coronary artery, respectively. In Figure 2 , CTCA and CFVR measured using TTDE indicated significant ISR in overlapping 3.0- × 18-mm and 3.0- × 12-mm drug-eluting stents in the proximal LAD, which were consistent with invasive coronary angiographic data. In Figure 3 , TTD echocardiogram clearly shows that CFVR was >2.0, indicating no ISR in the left circumflex coronary artery, which was consistent with invasive coronary angiographic data. However, assessment of ISR of a single drug-eluting stent with a 3.0- × 18-mm stent was not possible by CTCA because of metal artifacts. In Figure 4 , CTCA and CFVR measured using TTDE indicated no ISR in overlapping 3.5- × 33-mm and 3.0- × 33-mm drug-eluting stents in the right coronary artery, which were consistent with invasive coronary angiographic data. Diagnostic accuracy of CFVR measurement was similar to those of CTCA in all 3 major coronary arteries and in individual coronary arteries ( Table 4 ).
