Background
Myocardial deformation parameters obtained by speckle-tracking echocardiography (STE) and color Doppler tissue imaging (CDTI) using two different ultrasound systems and three different software packages were compared.
Methods
Apical four-chamber, short-axis grayscale, and color Doppler tissue images were prospectively acquired using Vivid 7 and iE33 ultrasound systems in 34 children and then analyzed using EchoPAC and QLAB (STE) and SPEQLE (CDTI).
Results
Measurement of myocardial deformation was feasible for all three modalities. Longitudinal strain (ϵ) measurements showed the lowest intraobserver and interobserver variability (intraobserver and interobserver coefficients of variation, 9% and 8% for EchoPAC, 5% and 6% for QLAB, and 14% and 16% for SPEQLE). In addition, longitudinal ϵ had a small bias and narrow limits of agreement when comparing different techniques. The coefficients of variation of circumferential ϵ by EchoPAC and QLAB were 12% and 11% (intraobserver) and 9% and 13% (interobserver), respectively. Circumferential ϵ by STE had a small systematic bias but relatively narrow limits of agreement. The reproducibility of radial ϵ measurements using STE was low, while CDTI ϵ provided better performance (intraobserver and interobserver coefficients of variation for radial posterior ϵ, 12% and 24% for EchoPAC, 39% and 56% for QLAB, and 12% and 14% for SPEQLE). Radial ϵ was on average 50% lower using QLAB compared with EchoPAC and SPEQLE. Systolic strain rate values obtained by STE were lower compared with CDTI-derived values. The limits of agreement for strain rate values among the three modalities were wide, and intraobserver and interobserver variability was poor for all three modalities.
Conclusions
Some deformation measurements (e.g., longitudinal and circumferential ϵ) are comparable among different ultrasound machines and software packages, whereas others are significantly different (e.g., radial ϵ and strain rate). This study stresses the need for an industry standard for these techniques.
Different echocardiographic techniques are available to quantify myocardial deformation (strain [ϵ] and strain rate [SR]) as measures of global and regional myocardial function. Various clinical applications have been proposed in adult heart disease, especially for the quantification of global and regional left ventricular (LV) function and for the evaluation of dyssynchrony. In pediatric and congenital heart disease, these techniques could also be useful for the quantification of right ventricular function and for functional assessment of univentricular hearts. Some of the problems that currently limit the clinical implementation of deformation imaging are that (1) the technology for measuring deformation is constantly evolving, (2) competing vendors and research groups propose dissimilar solutions, and (3) there has been inadequate evaluation of the feasibility and reproducibility of these different techniques, especially in pediatrics.
Currently, two different methods are used to measure myocardial deformation. The first is based on color Doppler tissue imaging (CDTI), which uses myocardial tissue Doppler data to measure myocardial velocity gradients and SR. These SR curves are temporally integrated to calculate myocardial ϵ curves. The advantage of this approach is its high temporal resolution, which is potentially advantageous for pediatric applications because of higher heart rates in children. The major disadvantage of CDTI is its angle dependency, which limits the analysis to myocardial segments moving in parallel to the direction of the ultrasound beam. The assessment of radial deformation is therefore limited to the posterior wall, and the measurement of circumferential deformation, rotation, and torsion proves challenging by CDTI. The angle dependency in combination with the relatively low signal-to-noise ratio has been reported to influence the feasibility and reproducibility of the measurements. Competing vendors have their own software packages for tissue Doppler–derived ϵ calculations. For the present study, we decided to use Software Package for Echocardiographic Quantification Leuven (SPEQLE; University of Leuven, Leuven, Belgium) because it includes a semiautomated tracking algorithm that allows the tracking of the myocardial segment of interest throughout the cardiac cycle while correcting for translational motion. It is a well-validated research tool that has been used in a large number of studies.
A second approach to measure myocardial deformation is two-dimensional speckle-tracking echocardiography (STE). This technique is based on frame-by-frame tracking of natural acoustic markers (speckles) that are generated by B-mode images. In contrast with Doppler tissue imaging, it has been shown to be relatively angle independent in experimental settings and can measure cardiac deformation in opposing directions using the same two-dimensional image, making it a two-dimensional technique. Nearly every vendor has currently commercialized a different solution for STE, but there are very few validation data on most of the techniques in pediatrics.
The aim of the present study was to compare different techniques to quantify myocardial deformation and their reproducibility and feasibility using images obtained with two ultrasound systems (Vivid 7, GE Healthcare, Milwaukee, WI; and iE33, Philips Medical Systems, Andover, MA) and three different software packages in children.
Methods
Study Participants
Children with or without heart disease were prospectively enrolled. Participants were recruited in the outpatient clinic when scheduled to undergo clinically indicated echocardiography and from healthy volunteers. Excluded from participation were patients with functional single ventricular physiology, unstable hemodynamics, and cardiac arrhythmias. No children were excluded a priori on the basis of expected poor echocardiographic windows. The institutional research ethics board approved this study, and written informed consent was obtained from each participant and/or his or her parents prior to enrollment in this prospective study.
Echocardiographic Image Acquisition
Echocardiographic examinations were performed using Vivid 7 and iE33 ultrasound systems. For the GE system, we used either an S4 or an S5 curved-array transducer, and for the Philips system, we used an S5 curved-array probe. Image acquisition was performed by the same experienced sonographer (C.S.) within a 30-min time frame. Each participant first underwent an extensive initial assessment of anatomic and cardiac function with one ultrasound system, which included the acquisition of tissue Doppler data and grayscale images optimized for speckle-tracking analysis. Subsequently, a less extensive assessment with the other ultrasound system followed. The order of ultrasound machines in which the examinations were done was dependent on the indication for the echocardiographic request. For children with cardiomyopathy and those without heart disease, the first examination was performed on the GE system and the second on the Philips system. For patients who needed echocardiographic examinations for anatomic follow-up, the Philips system was used first, followed by the GE system. The baseline assessment also included M-mode recordings from the parasternal long and short axes to calculate ejection time, cardiac cycle length, LV dimensions, and shortening fraction.
Speckle-Tracking Image Acquisition
Grayscale images for offline speckle-tracking analysis were acquired at frame rates of 55 to 90 frames/sec. Parasternal short-axis images were acquired at the midventricular level (showing both papillary muscles) and from standard apical four-chamber views. At least two cardiac cycles were stored for offline analysis. Color Doppler tissue images were acquired with the Vivid 7 machine at a frame rate > 130 frames/sec. Only the GE images were analyzed, because Philips color Doppler tissue images cannot be imported into SPEQLE. Parasternal short-axis images were taken at the midventricular level. For CDTI in the apical four-chamber view, each individual wall was scanned separately with a narrow sector width to optimize frame rate. The ultrasound beam was aligned as best as possible with the motion of the wall of interest, allowing a maximal angle of insonation of 30°. At least three to five cardiac cycles for CDTI were stored as raw data for offline analysis.
Offline Analysis
STE
The grayscale images acquired on the Vivid 7 machine were analyzed using EchoPAC version 7.0 (GE Healthcare). Analysis was performed according to the vendor’s instructions and as previously described. Briefly, the endocardial border was manually traced at end-systole (starting at the mid septum for short-axis and the basal septum for apical four-chamber views). Tracking was automatically performed, and the analysis was accepted after visual inspection and when the software indicated adequate tracking. If tracking was suboptimal, the endocardial border was retraced. If satisfactory tracking was not accomplished within 5 min, the nontracking segments were excluded from analysis. Lagrangian radial ϵ and SR curves and circumferential ϵ curves from the short-axis view (six segments: anterior septum, anterior, lateral, posterior, inferior, and septum) and longitudinal ϵ curves from the apical four-chamber view (six segments: basal septum, mid septum, apical septum, apical lateral, mid lateral, and basal lateral) were obtained. Strain curves were visually inspected, and if the absolute ϵ value was equal to or less than an arbitrarily set value of 5% in a certain segment, the measurement for that segment was excluded from the analysis. The automated timing of aortic valve closure was used. End-systolic values for ϵ and peak systolic and early diastolic values for SR were measured. All offline measurements with EchoPAC were performed by a single observer (C.S.).
The images acquired on the iE33 machine were analyzed using QLAB version 7.0 (Philips Medical Systems). The automated tracking analysis was performed in the apical four-chamber view and the parasternal short-axis view according to the vendor’s instructions. After automated tracking analysis, visual inspection of the tracking was performed. If the tracking was not satisfactory, manual adjustments were made to the tracking points throughout the cardiac cycle. If satisfactory tracking was not accomplished within 5 min, the nontracking segments were excluded from analysis. Lagrangian ϵ and SR curves were provided by the software. Strain curves were visually inspected, and the same criteria for segment exclusion as for EchoPAC were used. Because aortic valve closure is not provided by the software, we used the electrocardiographic trace to estimate the timing of end-systole and early diastole. All offline measurements with QLAB were performed by a single observer (L.P.K.).
CDTI
The CDTI data sets obtained with the Vivid 7 ultrasound system were exported into SPEQLE version 4. The analysis was performed as previously described. SPEQLE allows the estimation of natural SR curves by measuring the spatial velocity gradient in a user-defined region of interest. The lengths of the regions of interest were 8 and 10 mm for radial and longitudinal deformation, respectively. For radial ϵ and SR, the region of interest was manually placed at the midmyocardial portion of the posterior wall of the short-axis image. For longitudinal ϵ, the region of interest was placed in the mid portion of the basal, mid, and apical segments of the intraventricular septum and LV free wall (similar segments as were used for STE). An M-mode algorithm provided by the software was applied to track the region of interest throughout the cardiac cycle. Blood-pool pulsed-wave tissue Doppler traces of mitral valve inflow and aortic valve outflow were used to time cardiac events. Natural ϵ curves were obtained by integrating the mean SR profile over time and averaged for three to five cardiac cycles. Strain curves were visually inspected, and the same criteria for segment exclusion as for EchoPAC were used. Natural ϵ values were mathematically converted into Lagrangian ϵ values as previously described. All offline measurements with SPEQLE were performed by a single observer (L.P.K.).
Reproducibility and Data Analysis
Interobserver and intraobserver variability in offline measurements was assessed in 15 randomly selected studies of children with and without heart disease. To assess intraobserver variability, the same observer who performed all the measurements for one modality remeasured the same segments ≥2 weeks later to avoid recall bias (EchoPAC by C.S. and QLAB and SPEQLE by L.P.K.). To assess interobserver variability, deformation parameters were measured for each modality by one independent observer, who was blinded to the results of the other observer. Interobserver variability for EchoPAC was performed by L.P.K., for QLAB by C.S., and for SPEQLE by W.H.
Global longitudinal ϵ was based on the apical four-chamber view only, while global radial and circumferential ϵ and SR were based on the short-axis view at the papillary muscle level only. Global ϵ was calculated as the average of the segments when at least three of six segments were measurable. Data are presented as mean ± SD, as medians with minimum and maximum values, or as frequencies as appropriate. Agreement between machines, software, techniques for continuous variables was calculated using Bland-Altman statistics, including the calculation of mean bias (average difference between measurements), the statistical significance of the mean bias on paired t tests (the null hypothesis was zero bias), and the lower and upper limits of agreement (95% limits of agreement of mean bias). The percentage differences (the difference between paired measurements divided by the average of the two measurements times 100) were calculated for all Bland-Altman plots. Furthermore, Pearson’s correlations and their statistical significance were calculated as measures of raw associations between measurements. Interobserver and intraobserver variability were calculated using the same methodology and by calculating the coefficient of variation (the standard deviation of the difference of paired samples divided by the average of the paired samples). To investigate whether the difference in deformation parameters between two methods was related to the absolute value of the deformation parameter, univariate linear regression models using maximum likelihood estimates for parameter determination were used. These models used the difference of a given deformation parameter between two different methods as the dependent variable and the average deformation value of two methods as the independent variable. All statistical analyses were performed using SAS version 9.1 (SAS Institute Inc., Cary NC).
Results
General Characteristics
Table 1 shows the general patient characteristics and a selection of conventional echocardiographic measurements of the study group. Of the three children with cardiomyopathies, two had dilated cardiomyopathy and one had hypertrophic cardiomyopathy. Diagnosis of congenital heart disease ( n = 12) included tetralogy of Fallot ( n = 4), ventricular septal defects ( n = 2), LV outflow tract obstructions ( n = 4), Ebstein’s anomaly ( n = 1), and transposition of the great arteries ( n = 1). Most of the patients had normal ejection fractions.
Variable | Mean ± SD or n (%) | Range |
---|---|---|
Age (years) | 11.6 ± 3.5 | 5 to 18 |
Male | 20 (59%) | |
Height (cm) | 147 ± 17 | 114 to 171 |
Weight (kg) | 43 ± 16 | 17 to 74 |
Body surface area (m 2 ) | 1.3 ± 0.3 | 0.7 to 1.8 |
Body mass index (kg/m 2 ) | 19 ± 4 | 12 to 30 |
Systolic blood pressure (mm Hg) | 100 ± 10 | 81 to 125 |
Diastolic blood pressure (mm Hg) | 57 ± 8 | 44 to 78 |
Diagnosis | ||
Normal heart | 12 (35%) | |
Heart transplant recipient | 4 (12%) | |
Systemic arterial hypertension | 2 (6%) | |
Cardiomyopathy | 3 (9%) | |
Congenital heart disease | 13 (47%) | |
Heart rate (beats/min) | 74 | 41 to 97 |
LV end-diastolic diameter Z score | 0.92 ± 1.63 | −1.69 to 6.9 |
LV end-systolic diameter Z score | 0.64 ± 1.60 | −2.44 to 4.07 |
Shortening fraction (%) | 37 ± 7 | 23 to 53 |
LV ejection time (msec) | 300 ± 48 | 201 to 458 |
Feasibility of the Different Deformation Analysis Techniques
For the analysis of the speckle-tracking techniques, the mean frame rates for the short-axis grayscale images were 73 ± 19 frames/sec for Vivid 7 images and 73 ± 17 frames/sec for iE33 images ( p = .77). For the apical four-chamber images, the mean frame rates were 65 ± 16 frames/sec for Vivid 7 images and 71 ± 14 for iE33 images ( p = .03). The short-axis and apical four-chamber images used for CDTI analysis both had a mean frame rate of 191 ± 28 frames/sec. To obtain a full analysis of all the included segments, the offline analysis time was approximately 15 min for EchoPAC, 20 min for QLAB, and 45 min for SPEQLE.
For radial deformation, we aimed to analyze six segments by STE and one segment by CDTI (the posterior wall segment from the short-axis view). Feasibility for STE-derived radial ϵ was between 97% (inferior wall) and 100% (posterior wall) for EchoPAC and between 74% (anterior septal wall) and 97% (posterior wall) for QLAB. Feasibility for CDTI-derived radial ϵ at the posterior wall was 97%.
The feasibility of circumferential ϵ (applicable only to STE) was between 91% (inferior wall) and 100% (anterior septal wall) for EchoPAC and between 94% (anterior septal wall) and 100% (septal wall) for QLAB.
For longitudinal ϵ analysis, we aimed to measure six segments from the apical four-chamber view using all three techniques. With the speckle-tracking techniques, global and regional longitudinal ϵ measurements were possible in the majority of patients (EchoPAC: global 97%, regional 91%; QLAB: global 100%, regional 97%). The feasibility of the longitudinal measurements was lower using the CDTI technique, at 88% for global ϵ measurements and 53% of the basal lateral wall segments. The main reasons for excluding measurements were as follows: for speckle tracking, inconsistent ϵ curves due to reverberation artifacts or poor echo windows, and for CDTI, reverberation artifacts, poor signal-to-noise ratio, and poor ultrasound beam alignment with the motion of the interrogated myocardial wall. There was no difference in exclusion rates between patients with normal hearts and those with heart disease (data not shown).
Reproducibility
Intraobserver and interobserver variability results are shown in Table 2 . Overall, the variability of radial ϵ measurements was higher compared with circumferential and longitudinal measurements. QLAB and EchoPAC showed poor reproducibility for radial ϵ measurements, while SPEQLE had better reproducibility for radial ϵ in the posterior wall, which is the only segment in which it can be reliably measured using this technique. Circumferential ϵ intraobserver and interobserver variability was similar for EchoPAC and QLAB and lower compared with radial ϵ measurements. Variability for longitudinal ϵ was overall lower and comparable between the two speckle-tracking modalities while being somewhat higher for SPEQLE. Overall, variability was higher when the different segments were analyzed separately compared to global deformation parameters (data shown only for radial ϵ and SR posterior wall short axis). SR measurements had higher variability compared with ϵ measurements, and diastolic SR measurements were more variable compared with systolic measurements.
EchoPAC | QLAB | SPEQLE | |||||||||||||
---|---|---|---|---|---|---|---|---|---|---|---|---|---|---|---|
Variable | Mean ± SD | Bias | LCL | UCL | COV | Mean ± SD | Bias | LCL | UCL | COV | Mean ± SD | Bias | LCL | UCL | COV |
Intraobserver variability | |||||||||||||||
Radial ϵ posterior wall (%) | 57 ± 19 | −4.7 | −18 | 9 | 11.9 | 31 ± 11 | 1.3 | −22 | 25 | 38.6 | 48 ± 10 | 2.0 | −9 | 13 | 12.0 |
Radial S-SR posterior wall (sec −1 ) | 1.9 ± 0.4 | −0.0 | −0.6 | 0.6 | 15.7 | 1.7 ± 0.9 | 0.2 | −1.1 | 1.5 | 39.5 | 2.8 ± 0.6 | 0.1 | −1.0 | 1.2 | 20.4 |
Radial ED-SR posterior wall (sec −1 ) | −2.3 ± 0.7 | 0.1 | −1.4 | 1.5 | 31.9 | −4.4 ± 1.6 | 0.7 | −2.2 | 3.4 | 30.0 | −4.0 ± 1.3 | −0.1 | −2.1 | 2.0 | 25.9 |
Global radial ϵ (%) | 48 ± 13 | −5.0 | −13 | 5 | 9.7 | 23 ± 7 | −0.2 | −12 | 12 | 25.8 | NA | NA | NA | NA | NA |
Global radial S-SR (%) | 1.8 ± 0.3 | 0.0 | −0.4 | 0.4 | 12.8 | 1.6 ± 0.6 | −0.0 | −1.1 | 1.1 | 33.4 | NA | NA | NA | NA | NA |
Global radial ED-SR (%) | −2.1 ± 0.6 | −0.0 | −0.9 | 0.8 | 20.5 | −3.0 ± 0.7 | 0.1 | −1.1 | 1.2 | 19.3 | NA | NA | NA | NA | NA |
Global circumferential ϵ (%) | −18 ± 2 | −0.4 | −4.8 | 3.4 | 11.6 | −21 ± 3 | −0.2 | −4.0 | 3.5 | 9.0 | NA | NA | NA | NA | NA |
Global longitudinal ϵ (%) | −20 ± 2 | −0.2 | −3.7 | 3.3 | 8.9 | −20 ± 2 | 0.7 | −2.8 | 1.4 | 5.2 | −21 ± 4 | 0.9 | −5.0 | 6.7 | 14.0 |
Interobserver variability | |||||||||||||||
Radial ϵ posterior wall (%) | 59 ± 19 | −1.3 | −29 | 26 | 23.8 | 29 ± 11 | −2.7 | −34 | 29 | 55.6 | 46 ± 10 | −1.6 | −14 | 11 | 14.0 |
Radial S-SR posterior wall (sec −1 ) | 2.1 ± 0.4 | 0.3 | −0.4 | 1.0 | 17.9 | 1.6 ± 0.9 | 0.1 | −1.0 | 1.2 | 34.5 | 2.7 ± 0.6 | −0.2 | −1.2 | 0.9 | 20.3 |
Radial ED-SR posterior wall (sec −1 ) | −2.5 ± 0.7 | −0.3 | −1.9 | 1.4 | 33.5 | −4.5 ± 2.0 | 0.8 | −4.0 | 5.7 | 57.0 | −3.8 ± 1.3 | 0.2 | −1.5 | 1.9 | 22.6 |
Global radial ϵ (%) | 49 ± 13 | −0.8 | −17 | 16 | 17.3 | 23 ± 5 | −1 | −14 | 12 | 26.7 | NA | NA | NA | NA | NA |
Global radial S-SR (%) | 1.9 ± 0.4 | 0.2 | −0.3 | 0.8 | 15.8 | 1.7 ± 0.5 | −0.0 | −1.3 | 1.3 | 38.1 | NA | NA | NA | NA | NA |
Global radial ED-SR (%) | −2.2 ± 0.7 | −0.3 | −1.4 | 0.8 | 25.7 | −3.0 ± 0.6 | −0.1 | −2.1 | 2.0 | 30.3 | NA | NA | NA | NA | NA |
Global circumferential ϵ (%) | −19 ± 3 | −1.9 | −6.0 | 2.2 | 11.0 | −21 ± 3 | −0.2 | −5.6 | 5.3 | 13.2 | NA | NA | NA | NA | NA |
Global longitudinal ϵ (%) | −20 ± 2 | −0.7 | −3.9 | 2.4 | 8.0 | −20 ± 2 | 0.7 | −2.9 | 1.6 | 5.6 | −22 ± 5 | 0.3 | −6.4 | 7.0 | 15.7 |
Comparison of Myocardial Deformation Parameters Using EchoPAC, QLAB, and SPEQLE
Tables 3 and 4 summarize the findings when comparing radial, circumferential, and longitudinal ϵ and radial SR values between different modalities.
Variable | n , EchoPac | Mean ± SD, EchoPAC | n , QLAB | Mean ± SD, QLAB | n , SPEQLE | Mean ± SD, SPEQLE | Bias, EchoPAC-QLAB (LOA) | R , EchoPAC-QLAB | Bias, SPEQLE-EchoPAC (LOA) | R , SPEQLE- EchoPAC | Bias, SPEQLE-QLAB (LOA) | R , SPEQLE-QLAB |
---|---|---|---|---|---|---|---|---|---|---|---|---|
Radial ϵ posterior wall (%) | 34 | 55 ± 21 | 33 | 29 ± −13 | 33 | 60 ± 15 | 27 (−11 to 65) ∗ | 0.40 † | 4 (−44 to 52) | 0.06 | 31 (−5 to 68) | 0.11 |
Radial S-SR post. wall (sec −1 ) | 34 | 1.8 ± 0.4 | 33 | 1.5 ± 0.7 | 32 | 3.3 ± 1.0 | 0.3 (−1.0 to 1.6) ‡ | 0.45 ‡ | 1.5 (−0.2 to 3.2) ∗ | 0.48 ‡ | 1.8 (−0.2 to 3.9) ∗ | 0.48 ‡ |
Radial ED-SR posterior wall (sec −1 ) | 34 | −2.3 ± 0.8 | 33 | −4.8 ± 2.3 | 32 | −5.5 ± 2.4 | 2.5 (−1.7 to 6.6) ∗ | 0.40 † | −3.3 (−7.8 to 1.2) ∗ | 0.28 | −0.7 (−7.1 to 5.6) | 0.28 |
Global radial ϵ (%) | 34 | 47 ± 15 | 34 | 23 ± 6 | NA | NA | 24 (−6 to 54) ∗ | 0.19 | NA | NA | NA | NA |
Global radial S-SR (sec −1 ) | 34 | 1.7 ± 0.3 | 34 | 1.5 ± 0.6 | NA | NA | 0.2 (−0.9 to 1.3) § | 0.26 | NA | NA | NA | NA |
Global radial ED-SR (sec −1 ) | 34 | −2.1 ± 0.7 | 34 | −3.0 ± 0.8 | NA | NA | 0.9 (−0.7 to 2.5) ∗ | 0.40 † | NA | NA | NA | NA |
Global circumferential ϵ (sec −1 ) | 34 | −18 ± 2 | 34 | −21 ± 3 | NA | NA | 2 (−4 to 9) ∗ | 0.42 ‡ | NA | NA | NA | NA |
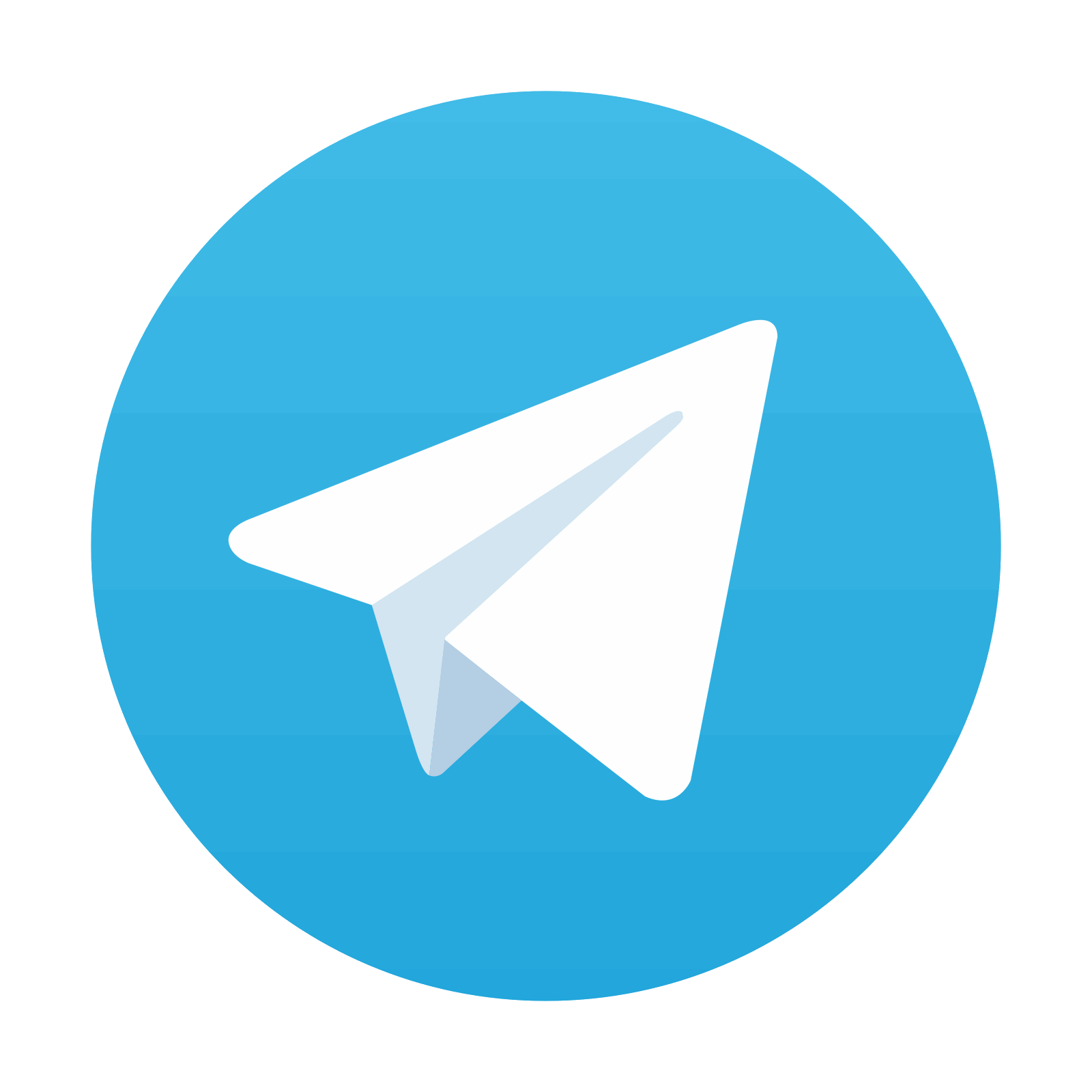
Stay updated, free articles. Join our Telegram channel

Full access? Get Clinical Tree
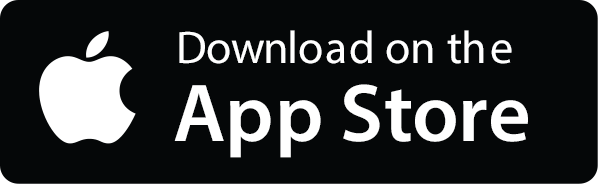
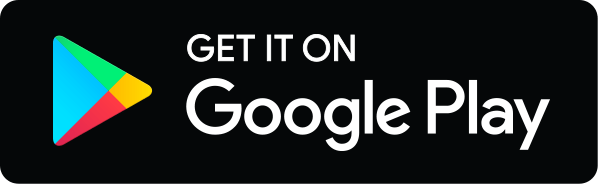
