Background
Identification of mitral regurgitation (MR) mechanism and pathology are crucial for surgical repair. The aim of the present investigation was to evaluate the comparative accuracy of real-time three-dimensional (3D) transesophageal echocardiography (TEE) and transthoracic echocardiography (TTE) with two-dimensional (2D) TEE and TTE in diagnosing the mechanism of MR compared with the surgical standard.
Methods
Forty patients referred for surgical mitral valve repair were studied; 2D and 3D echocardiography with both TTE and TEE were performed preoperatively. Two independent observers reviewed the studies for MR pathology, functional or organic. In organic disease, the presence and localization of leaflet prolapse and/or flail were noted. Surgical findings served as the gold standard.
Results
There was 100% agreement in identifying functional versus organic MR among all four modalities. Overall, 2D TTE, 2D TEE, and 3D TEE performed similarly in identifying a prolapse or a flail leaflet; 3D TEE had the best agreement in identifying anterior leaflet prolapse, and it also showed an advantage for segmental analysis. Three-dimensional TTE was less sensitive and less accurate in identifying flail segments.
Conclusion
All modalities were equally reliable in identifying functional MR. Both 2D TEE and 3D TEE were comparable in diagnosing MR mechanism, while 3D TEE had the advantage of better localizing the disease. With current technology, 3D TTE was the least reliable in identifying valve pathology.
Significant mitral regurgitation (MR) is a common disease for which valve repair is the preferred operation. Identification of the mechanism of regurgitation and localization of the pathology of the valve are crucial for planning surgical management. Echocardiography is at present the first-line modality for the assessment of the etiology and severity of MR. Traditionally, two-dimensional (2D) transthoracic echocardiography (TTE) was the main modality for this evaluation. Localization of the involved leaflet or scallop has been difficult and was enhanced with the introduction of multiplane 2D transesophageal echocardiography (TEE). Although 2D TEE has high resolution, segmental analysis aiming to localize disease remains challenging. With the advent of real-time three-dimensional (3D) echocardiography (initially TTE and more recently TEE), volumetric imaging is now possible, providing 3D images from the atrial and ventricular side as well as unlimited 2D tomographic planes. Therefore, 3D imaging has the potential of better disease localization and segmental analysis. Previous studies have shown an advantage of 3D echocardiography in evaluating the mitral valve, particularly with the transesophageal approach. Whether 3D TTE offers a similar advantage to 3D-TEE or may be limited by image resolution despite the versatility of imaging views and planes is not known. To date, there has been no head-to-head comparison of all four available modalities (2D TTE and TEE, live 3D TTE and TEE) in the accuracy of diagnosing mitral valve pathology. Accordingly, the present study was undertaken to evaluate the comparative accuracy of the four echocardiographic approaches in diagnosing and localizing disease of the mitral valve compared to the surgical standard in patients with significant MR.
Methods
Patient Population
Consecutive patients with significant MR who were referred for mitral valve repair were prospectively approached and enrolled in the present study. The presence of atrial fibrillation or a poor acoustic window was not a contraindication for this study. All patients provided written informed consent, and the study was approved by the local human research review board.
Two-Dimensional and 3D Imaging of the Mitral Valve
The study protocol included a total of four echocardiographic studies for each patient: 2D TTE and TEE and 3D TTE and TEE. Standard 2D TTE was performed while the patient was alert (usually 1 day before the operation), using standard parasternal and apical views along with color Doppler and pulsed-wave and continuous-wave Doppler for evaluation of the mitral valve. After 2D TTE, a standardized 3D transthoracic echocardiographic imaging protocol was performed. The 3D images included parasternal and apical views, real-time zoom, full-volume, and color acquisitions aiming to visualize the mitral valve. The full-volume acquisition involved stitching four to seven consecutive beats, with avoidance of stitching artifacts. In the operating room, under general anesthesia and before the procedure was performed, patients underwent routine 2D TEE according to the intraoperative transesophageal echocardiographic guidelines of the American Society of Echocardiography using a multiplane probe. Attention again was focused on the mitral valve in addition to the other structures. This was followed by 3D TEE. The standardized protocol for 3D TEE included midesophageal and transgastric images, real-time zoom, full-volume, and color Doppler acquisition focusing on the mitral valve. In general, the 3D acquisition protocol was performed by the same 2D operator. Aiming to minimize bias, the operators were asked to adhere to each study protocol with imaging from all views described above. The imaging was performed using an iE33 ultrasound system (Philips Medical Systems, Andover, MA). The S5-1 probe was used for 2D TTE and the X3-1 probe for 3D TTE. Two-dimensional and 3D TEE was performed using the X7-2t probe. The 2D and 3D images were digitally stored and were analyzed at a later time.
Data Analysis
Two independent observers, blinded to the surgical findings and all other data, reviewed the 2D and 3D transthoracic and transesophageal echocardiographic studies. Both observers are experts in the field. One read the 2D studies (S.F.N.) and the other the 3D studies (S.H.L.); the latter had >6 years experience with 3D techniques and analysis. The transthoracic and transesophageal echocardiographic studies were read at random and separated in time by >2 months. The reason we chose to have the same reader for 2D studies and for 3D studies was to decrease the influence of interobserver variability on the results while spacing the reads far enough apart and in a random fashion for the reader not to be influenced by the previous findings of the same study.
The 2D studies were read using an offline station (Digisonics Inc., Houston, TX). The 3D studies were read using QLAB version 7 (Philips Medical Systems). Left ventricular ejection fraction was calculated using the method of disks. The severity of MR was assessed using color Doppler; MR volume and fraction were calculated as recommended by the guidelines of the American Society of Echocardiography. The 2D observer had all the images of the study for evaluation. The 3D observer had access to all 3D images (live zoom, full-volume, or color). Although he had the freedom to choose any acquisition, there was a preference to use live zoom images. The 3D data were reviewed in volumetric format from the left atrial view (en face view) and in multiple 2D planes derived from the volumetric data. The en face view is achieved by positioning the left atrium at the top of the volumetric data; subsequently, the aortic valve is identified and localized anteriorly to create the surgical view. The volumetric data can also be presented with multiple 2D planes, allowing 3D orientation and thus facilitating the localization of anatomic structures. Identification of the mitral leaflets segments was based on both the 3D en face view and the multiple 2D planes. In addition, the readers were asked to identify the mechanism of MR, organic of functional. Functional MR was defined as secondary MR: poor coaptation of the leaflets without significant calcifications, prolapse or flail, an enlarged annulus, a restricted leaflet, and/or a dilated left ventricle. For patients with organic MR, the presence of prolapsed or flail segments was noted. Localizing the segmental disease (scallop) was reported on 2D TEE, 3D TTE, and 3D TEE but could not be performed reliably on 2D TTE. A prolapsed scallop was identified as the presence of a billowing segment (>2 mm) toward the left atrium, whereas a flail segment was noted as a protruding, highly mobile segment with torn chordae. The localization of the pathology was performed according to the Carpentier nomenclature.
All patients were operated by the same surgeon (G.M.L.), who was aware of both preliminary 2D and 3D transesophageal echocardiographic findings. Surgical findings served as the gold standard; leaflet mobility was assessed by pulling the free edge of each leaflet toward the left atrium, with forceful saline injection through the valve with a bulb syringe simulating LV systolic pressure and its effects on producing MR. A prolapsed leaflet was defined if the free edge of each leaflet was overriding the plane of the annulus. Evaluation of one or more ruptured chordae was performed by direct inspection, and the involved leaflet scallop was identified. As part of the protocol, the surgeon reported his diagnosis by the end of the operation.
Statistical Analysis
Continuous variables are expressed as mean ± SD and discrete variables as percentages. Contingency tables were constructed to calculate sensitivity, specificity, and accuracy for 2D and 3D echocardiographic findings compared with those at surgery. These were constructed for the following diagnoses: any diagnosis of prolapse, prolapse located at the anterior leaflet, prolapse located at the posterior leaflet, any diagnosis of flail leaflet, flail leaflet located at the anterior leaflet, and leaflet located at the posterior leaflet. This was also repeated for segmental diagnosis (A1, A2, and A3 and P1, P2, and P3). McNemar’s test was used to compare sensitivity and specificity by the various modalities. Paired t tests were used to compare the accuracy of 2D and 3D echocardiography with that at surgery. Kappa coefficients were calculated to assess agreement between any modality (2D TTE, 2D TEE, 3D TTE, and 3D TEE) and the surgical diagnosis. P values < .05 were considered significant. The statistical analysis was done using SPSS version 15 (SPSS, Inc., Chicago, IL).
Results
Patient Population
The patient population included 40 patients (27 men; mean age, 59.4 ± 10.4 years) with significant MR who ultimately underwent mitral valve repair after the echocardiographic evaluation with the four modalities. Table 1 summarizes the occurrence of the different types of mitral valve pathology encountered at surgery. No patient was excluded because of suboptimal image quality. Ejection fraction was estimated at 60 ± 12% (range, 35%–70%); the regurgitation volume and fraction measured 72.1 ± 26.8 mL and 50.3 ± 9.4%, respectively.
Mitral valve pathology | n |
---|---|
Organic MR | 34 |
Functional MR | 6 |
Any leaflet prolapse | 31 |
Anterior leaflet prolapse | 16 |
Posterior leaflet prolapse | 28 |
Any flail leaflet | 22 |
Flail anterior leaflet | 1 |
Flail posterior leaflet | 21 |
P1 prolapse | 15 |
P2 prolapse | 28 |
P3 prolapse | 14 |
A1 prolapse | 14 |
A2 prolapse | 16 |
A3 prolapse | 13 |
P2 flail | 21 |
A2 flail | 1 |
General Identification of Prolapse or Flail
There were 34 patients with organic MR and six patients with functional MR.
One hundred percent agreement was observed in the ability to identify mitral valve pathology (functional vs organic MR) among the four modalities. For patients with organic MR, Figure 1 summarizes the sensitivity, specificity, accuracy, and κ coefficient of each of the four modalities in identifying any prolapsed segment, anterior or posterior leaflet prolapse, any flail segment, and anterior or posterior flail leaflet. There was only one anterior leaflet flail (localized at A2) that was not identified by any modality. Compared with 2D TEE and 3D TEE, 3D TTE was significantly less sensitive and less accurate in identifying flail segments and in localizing them to the posterior leaflet. The use of 2D TTE allowed the identification of flail segments and localizing them in the posterior leaflet better than 3D TTE. Overall, 2D TTE, 2D TEE, and 3D TEE performed similarly in identifying a prolapse or a flail leaflet, without further localization. Three-dimensional TEE had the best agreement in identifying anterior leaflet prolapse ( Figure 1 D).
Localization of Specific Mitral Valve Scallop Pathology
In the 40 patients, 101 of the 240 mitral valve scallops were identified with prolapse by the surgeon. The sensitivity, specificity, and accuracy of 2D TEE, 3D TTE, and 3D TEE in identifying the precise scallop prolapse are shown in Figure 2 . Three-dimensional TTE had the lowest sensitivity, with good specificity. The highest sensitivity was with 2D TEE but with the lowest specificity for localizing the prolapsed scallop. Three-dimensional TEE had intermediate sensitivity and the best specificity and overall accuracy.
A similar analysis was performed for the localization of flail segments (22 of 240; Figure 2 ). Three-dimensional TTE again had the lowest sensitivity. Specificity was similar for all three modalities. Accuracy was similar for 2D and 3D TEE and was higher than that of 3D TTE.
The sensitivity, specificity, accuracy, and κ coefficients of 2D TEE, 3D TTE, and 3D TEE in identifying the specific scallop pathology at each location are shown in Figure 3 , with examples in Figures 4 to 6 . More heterogeneity in detection and accurate sublocalization of the valve pathology was noted among the imaging modalities. Although 2D TEE was very sensitive in detecting P1 and P3 prolapse, it was poorly specific. Three-dimensional TTE presented high specificity and overall low sensitivity, with the exception of identifying P2 prolapse. In general, 3D TEE provided better accuracy in localizing the scallop pathology; this was statistically significant for diagnosing flail P2 segments. Overall, 3D TEE presented higher and statistically significant level of agreement with surgical finding for segmental analysis.
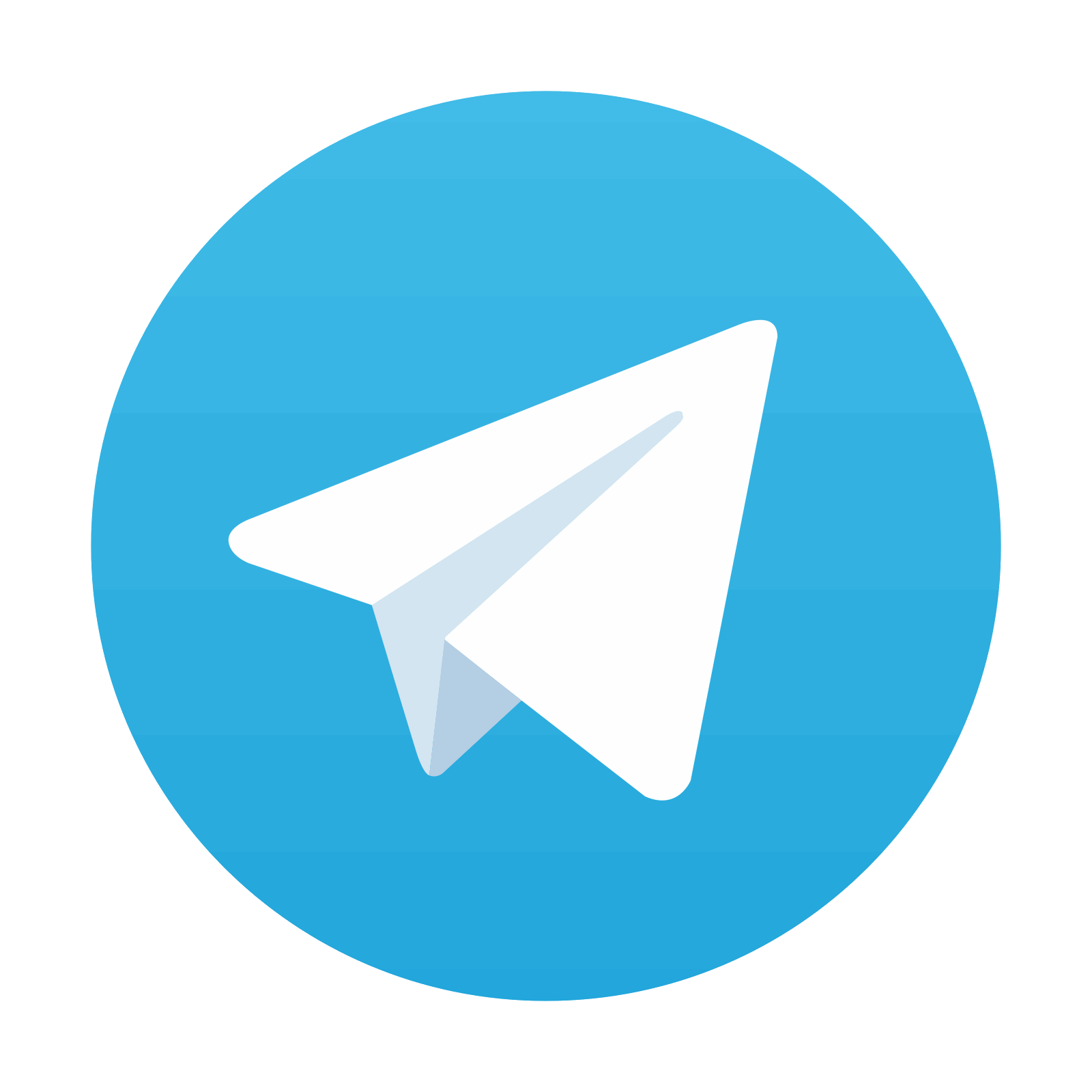
Stay updated, free articles. Join our Telegram channel

Full access? Get Clinical Tree
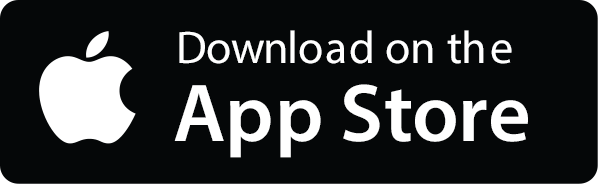
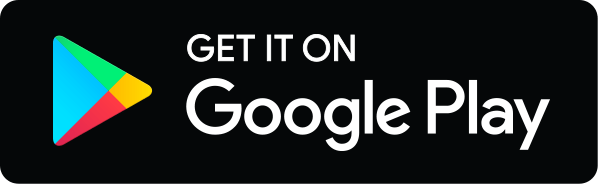
