Background
The objectives of this study were to test the utility of right ventricular (RV) speckle-tracking strain as an assessment tool for RV function in patients with pulmonary hypertension (PH) compared with conventional echocardiographic parameters and to investigate the relationship of the findings obtained with RV speckle-tracking strain with the hemodynamic parameters of RV performance.
Methods
Forty-five prospective consecutive patients with PH were studied. RV free wall longitudinal speckle-tracking strain (RV-free) and RV septal wall longitudinal speckle-tracking strain (RV-septal) were calculated by averaging each of three regional peak systolic strains along the entire right ventricle. The conventional echocardiographic parameters—RV fractional area change, RV myocardial performance index, tricuspid annular plane systolic excursion, and tricuspid annular peak systolic velocity—were also studied. For comparison, 22 age-matched volunteers with normal ejection fractions were studied.
Results
RV-free in patients with PH was significantly lower than that in normal controls, but RV-septal in the two groups was similar. Importantly, multivariate analysis revealed that RV-free was an independent echocardiographic predictor of hemodynamic RV performance items, including mean pulmonary artery pressure (β = −0.844, P = .001) and pulmonary vascular resistance (β = −0.045, P < .001). RV-free was also correlated with RV ejection fraction and RV end-systolic volume measured by cardiac magnetic resonance imaging and with 6-min walking distance ( r = 0.60, r = 0.56, and r = 0.49, respectively, P < .05). Furthermore, the improvement in RV-free 5 ± 3 months after adding medical treatment was significantly correlated with that in 6-min walking distance ( r = 0.68, P < .0001).
Conclusions
RV-free has the potential to allow for noninvasive follow-up of patients with PH.
The development of right ventricular (RV) dysfunction in patients with pulmonary hypertension (PH) has been associated with adverse outcomes regardless of the underlying clinical entity. Thus, the assessment of RV function has become increasingly important in the management of patients with PH. Most standard techniques for assessing RV hemodynamics, such as mean pulmonary artery pressure (mPAP) or pulmonary vascular resistance (PVR) are invasive and time consuming. In clinical practice, therefore, a visual assessment of RV function is performed most often. The current conventional echocardiographic techniques can be used to estimate RV performance, but the quantification of RV function remains a challenge because of the complex geometry of the chamber. A more recent development has been speckle-tracking echocardiography, which analyzes routine grayscale speckle patterns from frame to frame to derive quantitative regional functional data. This technique has been introduced recently for the evaluation of regional RV as well as left ventricular (LV) function. Accordingly, the objectives of this study were to test the utility of two-dimensional (2D) RV speckle-tracking strain compared with that of conventional echocardiographic parameters as a tool for RV functional assessment in patients with PH and to investigate the relationship of the findings obtained with 2D RV strain with the hemodynamic parameters of RV performance. Furthermore, we evaluated the utility of 2D RV strain for the effects of medical treatment in a subset of patients with PH.
Methods
Study Population
The study group consisted of 49 prospective consecutive patients with PH, defined as mPAP > 25 mm Hg at rest by right-heart cardiac catheterization. Patients with lung disease, left-sided heart failure, and more than mild aortic and/or mitral valvular heart disease were excluded from the study. This protocol was approved by the institutional review board on biomedical research, and all patients gave informed consent consistent with this protocol. Four patients (8%) with suboptimal images from poor echocardiographic windows were excluded from all subsequent analysis. Accordingly, the patient study group consisted of 45 patients, 36 women and 9 men ( Table 1 ). The mean age was 62 ± 14 years (range, 28–82 years), and the mean LV ejection fraction (LVEF) was 66 ± 6%. With regard to the etiology of PH (also listed in Table 1 ), only one patient (2%) was diagnosed with atrial fibrillation. Normal controls were randomly taken from our database to have a similar age distribution (range, 30–83 years). Thus, the normal control group consisted of 22 comparably aged volunteers with normal LVEFs (mean age, 57 ± 15 years; 72% women) with no histories of cardiovascular disease and completely normal results on electrocardiography and 2D and Doppler echocardiography (mean LVEF, 64 ± 4%). Indications for echocardiography were as follows: 15 normal volunteers (68%), five patients with chest pain (23%), and two patients with dyspnea (9%).
Variable | Patients with PH ( n = 45) | Normal controls ( n = 22) | P |
---|---|---|---|
Age (y) | 62 ± 14 | 57 ± 15 | .199 |
Men/women | 9/36 | 6/16 | .510 |
World Health Organization functional class (I/II/III/IV) | |||
I | 1 | ||
II | 31 | ||
III | 12 | ||
IV | 1 | ||
Etiology | |||
Idiopathic pulmonary arterial hypertension | 5 (11%) | ||
Collagen disease | 16 (36%) | ||
Other | 2 (4%) | ||
Congenital heart disease | 3 (7%) | ||
Chronic thromboembolic PH | 19 (42%) | ||
Heart rate (beats/min) | 70 ± 11 | 62 ± 6 | .012 |
Rhythm | .543 | ||
Sinus | 44 | 22 | |
Atrial fibrillation | 1 | 0 | |
Systolic blood pressure (mm Hg) | 112 ± 15 | 116 ± 11 | .317 |
Diastolic blood pressure (mm Hg) | 65 ± 8 | 62 ± 10 | .365 |
Medication | |||
Warfarin | 28 (62%) | ||
Bosentan | 17 (38%) | ||
Sildenafil | 16 (36%) | ||
Beraprost | 16 (36%) | ||
Diuretics | 12 (27%) | ||
Calcium blockers | 12 (27%) | ||
LVEF (%) | 66 ± 6 | 64 ± 4 | .102 |
Pulmonary artery systolic pressure by echocardiography (mm Hg) | 67 ± 23 | 20 ± 10 | <.0001 |
Echocardiography
All echocardiographic studies were performed with commercially available echocardiography systems equipped with a 3.5-MHz transducer (Vivid 7; GE Vingmed Ultrasound AS, Horten, Norway). Routine digital grayscale 2D and tissue Doppler cine loops were obtained at end-expiratory apnea from apical and parasternal views. Sector width was optimized to allow for complete myocardial visualization while maximizing frame rate regardless of heart rate, and frame rate was adjusted from 60 to 90 Hz (average, 76 Hz). LVEF was calculated from apical two-chamber and four-chamber images using the biplane Simpson’s technique. Pulmonary artery systolic pressure was estimated from the peak continuous-wave Doppler velocity of the tricuspid regurgitation jet plus estimated central venous pressure. All echocardiographic examinations were performed within 48 hours of catheterization, and measurements were obtained by an observer blinded to cardiac catheterization data. Follow-up echocardiography 5 ± 3 months after the initial evaluation was performed in a subset of 23 patients with PH to evaluate the effect of adding medical treatment (bosentan, sildenafil, or beraprost sodium).
Conventional Echocardiographic Assessment of RV Performance
RV Fractional Area Change (FAC)
RV end-diastolic and end-systolic areas were calculated from the modified apical four-chamber view, which focused on the right ventricle including the RV apex. RV FAC was calculated as follows: RV FAC = (RV end-diastolic area − RV end-systolic area)/RV end-diastolic area × 100% ( Figure 1 A).

RV Myocardial Performance Index
The sum of RV isovolumic contraction and relaxation time was obtained by subtracting RV ejection time from the interval between the cessation and onset of the tricuspid inflow velocities using pulsed-wave Doppler. The RV myocardial performance index was obtained by dividing the sum of both isovolumic intervals by the ejection time ( Figure 1 B). RV outflow and tricuspid inflow velocities were not obtained simultaneously.
Tricuspid Annular Plane Systolic Excursion (TAPSE)
An M-mode cursor was oriented to the junction of the tricuspid valve plane and the RV free wall using the apical four-chamber view to measure TAPSE. TAPSE was calculated as an index of RV global systolic function, which was determined as the total excursion of the tricuspid annulus from its highest position to the peak descent during ventricular systole ( Figure 1 C).
Tricuspid Annular Peak Systolic Velocity
Doppler tissue imaging recordings were obtained from the modified apical four-chamber view with a high frame rate (>150 frames/sec). To avoid aliasing, the Nyquist limit was adjusted to the lowest level. Pulsed Doppler tissue imaging was performed at the lateral corner of the tricuspid annulus. The tricuspid annular peak systolic (Sa), early diastolic (Ea), and atrial (Aa) wave velocities and Ea/Aa ratio were measured ( Figure 1 D).
Pulsed Doppler RV Inflow
Pulsed Doppler RV inflow was recorded in the modified apical four-chamber view, placing the sample volume at the level of the tips. Tricuspid inflow was measured for peak early inflow velocity (E), peak atrial velocity (A), and the E/A ratio.
Pulmonary Acceleration Time
Pulmonary acceleration time was measured as the interval between the onset of ejection and peak pulmonary flow velocity from the parasternal short-axis view.
RV Thickness
RV free wall thickness was measured using M mode from the subcostal view at the peak of the R wave at the level of the tricuspid valve chordae tendineae. Care was taken to avoid overmeasurement by epicardial fat deposition and coarse trabeculations within the right ventricle.
Longitudinal 2D RV Strain
Two-dimensional speckle-tracking strain echocardiography of RV longitudinal deformation in the RV free and septal walls was performed using a routine grayscale modified apical four-chamber view. The measurements were performed offline using dedicated software (EchoPAC version BTO8; GE Vingmed Ultrasound AS). A region of interest was traced on the endocardium at end-diastole in the right ventricle from the modified apical four-chamber view using a point-and-click approach. A second, larger region of interest was then generated and manually adjusted near the epicardium. Special care was taken to fine-tune the region of interest, using visual assessment during cine loop playback, to ensure that segments were tracked appropriately. The right ventricle was divided into six standard segments (at the basal, middle, and apical levels), and six corresponding time-strain curves were generated. RV free wall longitudinal speckle-tracking strain (RV-free) and RV septal wall longitudinal speckle-tracking strain (RV-septal) were calculated by averaging each of three regional peak systolic strains along the entire right ventricle ( Figure 2 ). The interobserver and intraobserver variability of 2D RV strain was expressed as the absolute difference between the measurements divided by their mean value from 10 randomly selected patients in alphabetical order.

Hemodynamic Measurement
All patients underwent right-heart cardiac catheterization for hemodynamic measurements. Mean pulmonary artery pressure and PVR were calculated using the estimated Fick principle. An investigator blinded to the echocardiographic data acquired the pressure measurements.
Exercise Tolerance
Exercise capacity was measured with a self-paced, unsupported 6-min walking test for all patients without walking difficulties to test the association with 2D RV strain.
Cardiac Magnetic Resonance (CMR) Imaging
A subset of 16 patients with PH was also studied with CMR imaging to assess RV ejection fraction (RVEF) and volumes within 48 hours of echocardiography. This study was performed for a research purpose from randomly selected patients. CMR imaging was performed using a Philips Achieva 1.5-T system (Philips Medical Systems, Best, The Netherlands) using a retrospective balanced turbo field echo sequence, as previously reported (repetition time, 2.8 msec; echo time, 1.4 msec; 30 frames/cardiac cycle; matrix size, 360 × 360; pixel resolution, 1.4 × 1.4 mm). CMR analysis was performed offline using dedicated software (Virtual Place Fujin Plus; AZE, Tokyo, Japan) by a single experienced observer blinded to echocardiographic results. End-diastolic and end-systolic contours were manually traced for each slice in the short-axis view. RV volumes were calculated from the area within the contours, and the slice thickness was determined according to the modified Simpson’s rule.
Statistical Analysis
All group data were compared using two-tailed Student’s t tests for paired and unpaired data and are presented as mean ± SD. Proportional differences were evaluated using Fisher’s exact tests or χ 2 tests as appropriate. Univariate linear correlation analysis was used for comparison of echocardiographic data and catheterization data, CMR data, and 6-min walking distances. To determine independent predictors of the hemodynamic measurements, including mPAP and PVR, multiple linear regression analysis with stepwise selection was performed. Candidate predictors were age, gender, myocardial performance index, TAPSE, RV FAC, tricuspid annular peak systolic velocity, and 2D RV strain. We converted tricuspid annular peak systolic velocity into a logarithmic scale according to its distribution. For all tests, P values < .05 were considered statistically significant. All analyses were performed with SPSS version 15.0 (SPSS, Inc., Chicago, IL) and R version 2.10.1 (R Foundation for Statistical Computing, Vienna, Austria).
Results
Baseline Characteristic and Standard Echocardiography
The study group consisted of 45 patients for whom complete data sets consisting of baseline echocardiographic and hemodynamic data were available and 22 normal volunteers with baseline echocardiographic data. CMR data were available for 16 patients. Longitudinal 2D RV strain measurement was possible in 97% of 402 attempted segments from 67 subjects (45 patients and 22 normal controls) with technically adequate images. Only 3% of segments had to be eliminated. If all segments of a given patient could not be assessed, only assessable segments were included for RV-free and RV-septal analysis. However, in only five of 67 subjects (7%) could we not evaluate strain values from all segments, which may not have affected our findings for RV-free and RV-septal analysis. All subjects had complete data sets of conventional echocardiographic RV parameters.
The baseline clinical and standard echocardiographic characteristics of the 45 patients with PH and the 22 normal controls are summarized in Table 1 . Heart rates in patients with PH were significantly faster than in normal controls. As expected, pulmonary artery systolic pressure in patients with PH was significantly greater than in normal controls (67 ± 23 vs 20 ± 10 mm Hg; range, 12–29 mm Hg; P < .0001 vs normal controls). There were no significant differences in LVEF between the two groups ( Table 1 ). Twenty patients had visual wall motion abnormalities in the RV lateral free wall. Table 2 shows the baseline echocardiographic characteristics of patients with PH and normal controls. RV-free in patients with PH was significantly lower in normal controls (19.8 ± 6.9% vs 25.8 ± 5.2%, P < .005), while RV-septal was similar in the two groups (19.3 ± 4.1% vs 20.9 ± 3.6%, P = .17) ( Figure 3 ). Compared with the etiology of PH on the basis of Dana Point classification (a clinical classification of PH according to the 4th World Symposium on PH, held in 2008 in Dana Point, CA), RV strains in patients with pulmonary arterial hypertension (Dana Point classification group 1, n = 26) were similar to those in patients with chronic thromboembolic PH (Dana Point classification group 4, n = 19). The interobserver and intraobserver variability were 10 ± 10% and 11 ± 14% for RV-free and 13 ± 10% and 15 ± 11% for RV-septal, respectively.
Variable | Patients with PH ( n = 45) | Normal controls ( n = 22) | P |
---|---|---|---|
RV FAC (%) | 37 ± 13 | 51 ± 1 | <.001 |
RV myocardial performance index | 0.4 ± 0.1 | 0.2 ± 0.1 | <.001 |
TAPSE (mm) | 18 ± 4 | 21 ± 3 | <.001 |
E (cm/s) | 40 ± 15 | 46 ± 11 | .15 |
A (cm/s) | 43 ± 17 | 34 ± 80 | <.001 |
E/A | 0.98 ± 0.35 | 1.46 ± 0.58 | <.001 |
Sa (cm/s) | 11.8 ± 2.9 | 14.1 ± 2.4 | <.001 |
Ea (cm/s) | 9.49 ± 0.28 | 12.56 ± 3.63 | <.001 |
Ea/Aa | 0.65 ± 0.29 | 0.92 ± 0.26 | <.001 |
E/Ea | 4.6 ± 2.5 | 3.9 ± 1.2 | .26 |
Pulmonary acceleration time (msec) | 81 ± 17 | 143 ± 16 | <.001 |
RV wall thickness (mm) | 4.0 ± 1.0 | 2.3 ± 0.4 | <.001 |
RV-free | 19.8 ± 6.9 | 25.8 ± 5.2 | <.005 |
RV-septal | 19.3 ± 4.1 | 20.9 ± 3.6 | .17 |
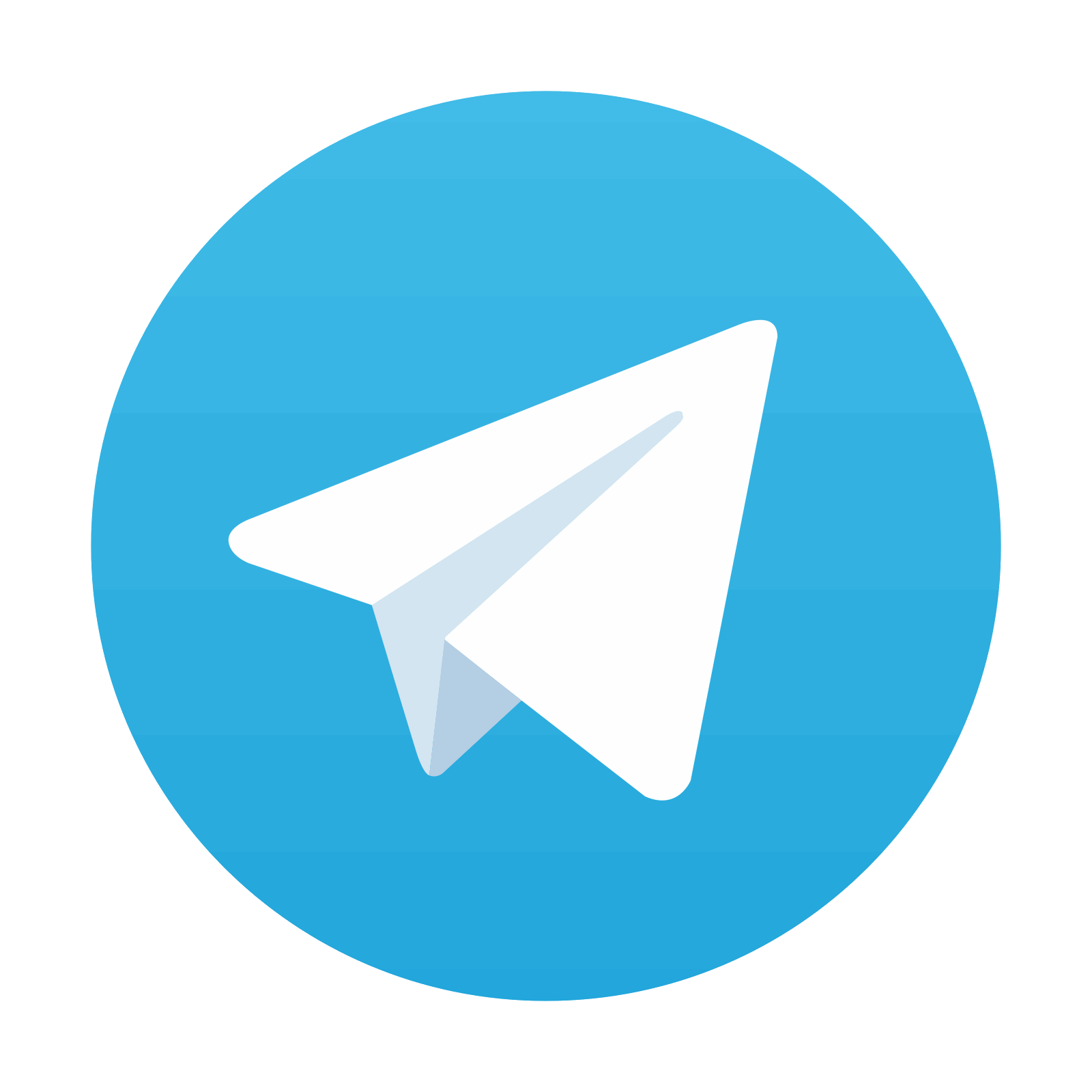
Stay updated, free articles. Join our Telegram channel

Full access? Get Clinical Tree
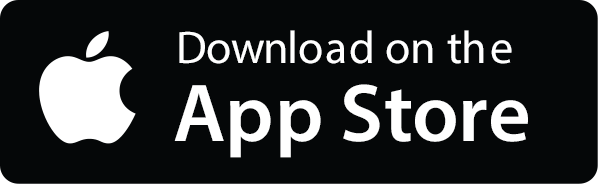
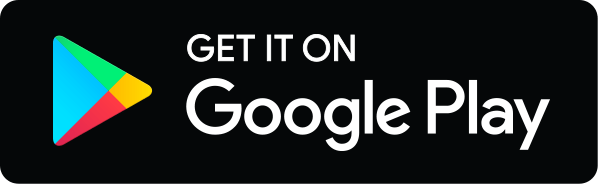
