Background
We aimed to evaluate the diagnostic utility of peak left atrial longitudinal strain (PALS) during left ventricular (LV) systole to differentiate children in the acute phase of Kawasaki disease (aKD) from controls. We also aimed to compare the diagnostic utility of PALS with those of conventional echocardiographic indices of diastolic function.
Methods
Retrospectively measured PALS, LV longitudinal peak systolic strain, and strain rate obtained via velocity vector imaging were compared in a derivation cohort comprising 95 aKD and 67 controls. The utility of PALS in differentiating aKD from controls was compared with those of E/E′, E/A, and maximum left atrial volume index (LAVImax). Derived cutoffs from receiver operating characteristic curves were validated in a separate validation cohort comprising 37 aKD and 19 controls.
Results
In the derivation cohort, PALS was significantly decreased in aKD as compared with in controls. For differentiating aKD from controls, PALS outperformed E/E′, E/A, and LAVImax. However, cutoffs of PALS (≤40% and ≤39%, before and after adjusting for the presence of significant mitral regurgitation and LV systolic dysfunction, respectively), like those of E/E′, E/A, and LAVImax, showed low sensitivity and poor discriminative ability for differentiating aKD from controls. In the validation cohort, for differentiating aKD from controls, both cutoffs of PALS showed low sensitivity, like those of E/E′, E/A, and LAVImax.
Conclusion
In aKD, impaired left atrial reservoir function could be detected as decreased PALS. For differentiating aKD from controls, PALS outperforms E/E′, E/A, and LAVImax. However, like E/E′, E/A, and LAVImax, PALS as a single parameter is limited in its clinical utility to differentiate aKD from controls because of its low sensitivity and poor discriminative ability.
Highlights
- •
Diagnostic utility of left atrial strain in Kawasaki disease was studied.
- •
Left atrial strain was compared with conventional echocardiographic indices.
- •
Left atrial strain is decreased in the acute stage of Kawasaki disease.
- •
Left atrial strain is not inferior to conventional echocardiographic indices.
- •
Low sensitivity of left atrial strain limits its utility as a single parameter.
Kawasaki disease (KD) is an acute systemic vasculitis occurring in young children. Left ventricular (LV) dysfunction can be detected in children in the acute phase of KD (aKD) and may be related to coronary vasculitis or myocarditis. In aKD, both LV systolic dysfunction (LVSD) and LV diastolic dysfunction (LVDD) have been detected. Since LVDD could precede LVSD and may occur in the absence of LVSD, the early detection of LVDD in aKD could aid in the diagnosis of KD, particularly when clinical criteria are insufficient, as in incomplete KD. Previously, the role of left atrial (LA) function in diastolic heart failure has been investigated in adults. However, to date, LA function via myocardial deformation analysis has not been evaluated in aKD.
LA acts as a reservoir of blood during ventricular systole, and peak LA longitudinal strain (PALS) during LV systole, which reflects LA reservoir function, is decreased in patients with heart failure and decreased ejection fraction (EF) due to impaired LA relaxation resulting from elevated LV end-diastolic pressure (LVEDP). Thus, LVDD, when present in aKD, may result in abnormalities in LA reservoir function, reflected as a decrease in PALS. We speculated that evaluating PALS in aKD for the detection of LVDD may aid in the diagnosis of KD. However, PALS could be affected by both significant mitral regurgitation (MR) and LVSD. Significant MR, observed in approximately 30% of aKD, can cause LA distension. LVSD, by impairing the descent of the mitral annulus to the apex, could impair the LA filling from the pulmonary veins in systole. Therefore, we evaluated the diagnostic utility of PALS in aKD both before and after, adjusting for the effects of significant MR and LVSD.
The aim of this study was to evaluate the clinical utility of PALS in differentiating aKD from controls. We also aimed to compare the utility of PALS in differentiating aKD from controls with that of conventional echocardiographic indices of diastolic function, such as E/A, E/E′, and LAVImax.
Methods
Study Design
We retrospectively studied the clinical and echocardiographic parameters of two separate cohorts, each cohort comprising aKD and controls, for the derivation and validation of cutoffs of PALS, E/A, E/E′, and LAVImax to differentiate aKD from controls. All aKD were further grouped into subgroups on the basis of the presence of significant MR and LVSD.
Derivation and Validation Cohorts
For the aKD in the derivation cohort, children fulfilling the criteria of the American Heart Association for KD 1 and admitted to the CHA Bundang Medical Center for treatment of KD from January 2013 to December 2016 were studied. Only patients with adequate imaging quality for Velocity Vector Imaging (Siemens Medical, Mountain View, CA) analysis were included. Patients with structural congenital heart disease, as well as those with fever and positive blood cultures or evidence of other febrile illnesses resembling KD, such as a history of exudative conjunctivitis, exudative pharyngitis, oral ulcerations, splenomegaly, and vesiculobullous or petechial rashes, were excluded. For controls, children who underwent echocardiography for evaluation of murmur without evidence of cardiac abnormalities were studied. For the validation cohort, a separate group of aKD who fulfilled the previously mentioned criteria for KD 1 and who were treated for KD, as mentioned previously, and a separate group of controls, defined as mentioned previously, were studied.
Echocardiographic Assessment
We reviewed the serial echocardiograms performed in all aKD prior to the initial treatment with intravenous immune globulin (IVIG) and at the convalescent phase (a median of 2 months after initial treatment) and compared them with the echocardiograms performed for the controls. Prior to echocardiography, all aKD received intravenous fluids to help alleviate dehydration and irritability from prolonged fever. Children who were uncooperative were sedated with oral chloral hydrate (25-50 mg/kg) so that they were calm and cooperative during echocardiography examination. After echocardiography was completed, IVIG was administered to all aKD.
Conventional Echocardiography
Transthoracic echocardiograms of all aKD and controls were obtained using commercial ultrasound equipment (Acuson SC 2000; Siemens Medical). Children were classified as having coronary artery lesions (CALs) when the z -scores of the internal diameter of the coronary arteries were found to be ≥2.5 in one or more of the right coronary and left anterior descending arteries. The z -scores of the coronary arteries were obtained using the measured dimensions of the coronary arteries as well as the nonlinear regression equations based on body surface area. LV EF, LV mass indexed to body surface area, peak early diastolic mitral inflow velocity (E), and peak atrial filling velocity during late diastole (A), E/A, peak early diastolic tissue Doppler velocity at the lateral mitral annulus (E′), and E/E′ were obtained according to the reported guidelines. Conventional LV measurements were recorded at the same time of echocardiography as the images for myocardial deformation analysis and were remeasured at the time of the deformation analysis.
Assessment of Myocardial Deformation
Echocardiographic digital images, which were previously obtained and stored, were analyzed offline. The Velocity Vector Imaging, version 3.0, software (Siemens Medical) was used to obtain PALS, LV longitudinal peak systolic strain (ε), and LV longitudinal peak systolic strain rate (SR). The median frame rate and frame rate ranges used for obtaining images were 54 and 51 to 76 frames per second, respectively. Clinical data and echocardiographic analysis were separately obtained by the different authors of our study. Echocardiographic images were analyzed offline by two independent observers (a pediatric cardiologist and an experienced cardiac sonographer), from whom the allocation of individual children into groups (KD vs controls) was concealed at the time of analysis and who were thus blinded at the time of echocardiographic analysis. After collection of both clinical and echocardiographic data was completed, the two groups of data were matched for statistical analysis. An average of three cardiac cycles was studied. From the apical four-chamber view, the endocardial border of the LA was manually traced at the end of systole, detected at the endpoint of the T wave on an electrocardiogram ( Figure 1 A). PALS was obtained from the three segments of the LA (septum, lateral wall, and roof) and was averaged ( Figure 1 B). LV longitudinal peak systolic ε and SR were obtained from the six segments of the LV from the apical four-chamber view and were averaged. Contours were evaluated for adequate tracking and adjusted if necessary. LA volume was obtained from the apical four-chamber view automatically by the Velocity Vector Imaging software as follows: After manual tracing at the end of systole, the LA wall was tracked automatically by the software during one cardiac cycle. The time-LA volume curve during one cardiac cycle was obtained automatically by measuring the LA volume from every frame using the single plane disk summation method (modified Simpson’s rule) ( Figure 1 C). The LA volume obtained from the time-LA volume curve has been shown to be accurate and in agreement with the LA volume obtained by manual tracking with the disk summation method. Maximum LA volumes were indexed to body surface area.

Assessment of Significant MR and LVSD
All aKD were further grouped into two subgroups, a subgroup of aKD without significant MR or LVSD as MR (−) LVSD (−) aKD and the remaining aKD as OtheraKD. MR was considered significant when it was more than mild MR. MR was graded as significant when the vena contracta width (VCW) was ≥ 0.3 cm and the continuous-wave Doppler jet was dense but partial or parabolic. LVSD was diagnosed as LV longitudinal peak systolic ε < 18%, according to published normal values of LV ε in children.
Clinical Parameters
Age, sex, duration of fever before and after initial treatment with IVIG, and prevalence of incomplete KD, CAL, and treatment nonresponse were studied. Nonresponders to IVIG treatment were defined as aKD who showed persistent or recrudescent fever 36 hours after completion of the initial IVIG infusion. Incomplete KD was diagnosed according to the American Heart Association guidelines. Data of blood samples from children prior to initial IVIG infusion were studied. White blood cell count, C-reactive protein, serum albumin, alanine aminotransferase, and N-terminal B-type natriuretic peptide (NT-proBNP) levels were studied.
The study protocol was approved by the Institutional Review Board and the Ethics Committee of the CHA University Bundang Medical Center (approval number 2015-10-180-005).
Statistics
Values are expressed as the mean ± SD for normally distributed data, or median [range] for nonnormally distributed data. The controls and all aKD were compared using the independent Student’s t -test or Mann–Whitney U -test. Data at the acute and convalescent phases of KD were compared using the paired t -test or Wilcoxon signed-rank test. The controls and the MR (−) LVSD (−) aKD and OtheraKD groups were compared using one-way analysis of variance or the Kruskal-Wallis test. Categorical variables were evaluated using the χ 2 test. Pearson or Spearman correlations were performed to determine the associations of variables and deformation data. The SPSS version 23 (IBM, Armonk, NY) was used for analysis. A P value < .05 was considered significant. To differentiate aKD from controls, cutoff values of PALS and E/E′, E/A, and LAVImax were obtained by receiver operating characteristic (ROC) curves using MedCalc for Windows, version 17.5 (MedCalc Software, Ostend, Belgium). Sensitivity and specificity of individual cutoffs were calculated according to standard definitions. Intraobserver variability was determined by offline reanalysis of PALS, LV longitudinal peak systolic ε, and SR in 20 randomly selected children 4 weeks apart. Interobserver variability was determined by a separate offline analysis of PALS, LV longitudinal peak systolic ε, and SR in 20 randomly selected children by two independent observers (a pediatric cardiologist and an experienced cardiac sonographer) who were blinded to patient clinical data at the time of analysis. Intraobserver and interobserver variabilities were calculated as intraclass correlation coefficients (ICCs) and 95% CIs of the ICCs and by the mean percentage error. This was obtained by calculating the absolute difference of the two data sets divided by the mean of the two data sets.
Results
After initially evaluating 162 aKD and 96 controls, we excluded 30 aKD and 10 controls because of poor image quality. In this study, 132 aKD and 86 controls were included. All aKD received 2 g/kg IVIG in a single dose at the time of diagnosis (at a median of 5 days after onset of fever) and were treated with high-dose oral aspirin (80 mg/kg/day) until afebrile for 3-4 days. Thereafter, they were given low-dose oral aspirin (5 mg/kg/day). All aKD showed no signs of overt heart failure, such as pulmonary edema or dyspnea. In the case of incomplete KD, prior to the diagnosis, the children received intravenous hydration and antipyretics and broad-spectrum intravenous antibiotics. Following the diagnosis of incomplete KD, children were treated with the same method as those with complete KD. For nonresponders, a second dose of 2 g/kg IVIG and high-dose oral aspirin were administered at the time of no response. No children in our study group received intravenous methylprednisolone because all nonresponders were afebrile after the second IVIG dose.
Derivation Cohort
Clinical Parameters
In the derivation cohort, 95 aKD and 67 controls were included. Thirty children (31.6%) had incomplete KD. The numbers of aKD who had CALs, initial treatment nonresponse, significant MR, and LVSD were 13 (13.7%), 15 (15.8%), 11 (11.6%), and 20 (21.1%), respectively. All 95 aKD were further grouped into the MR (−) LVSD (−) aKD group with 65 children and the OtheraKD group with 30 children. Clinical data of all aKD and controls are shown in Table 1 . Age, sex, body surface area, and heart rate were similar across all aKD and controls. The median systolic and diastolic blood pressures of all aKD were 100 mmHg and 60 mmHg, respectively, and both were similar to those of the controls. All laboratory parameters did not differ significantly between the MR (−) LVSD (−) aKD and OtheraKD groups.
Derivation cohort | Validation cohort | ||||||
---|---|---|---|---|---|---|---|
Variable | CTRL | All aKD | MR (−) LVSD (−) aKD | OtheraKD | CTRL | All aKD | MR (−) LVSD (−) aKD |
Age, mo | 24 [2-96] (67) | 24 [3-84] (95) | 24 [4-84] (65) | 29 ± 19 (30) | 31 ± 15 (19) | 24 [4-96] (37) | 24 [4-96] (34) |
Boys, % | 55.2 (37) | 55.8 (53) | 63.1 (41) | 40.0 (12) | 52.6 (10) | 56.8 (21) | 52.9 (18) |
BSA, m 2 | 0.55 [0.31-1.25] (62) | 0.54 [0.32-0.92] (93) | 0.54 [0.36-0.92] (63) | 0.54 ± 0.11 (30) | 0.56 ± 0.08 (19) | 0.57 ± 0.11 (37) | 0.57 ± 0.11 (34) |
Duration of fever at initial treatment, days | 5 [2-10] (95) | 5 [2-10] (65) | 5 [2-10] (30) | 5 [4-7] (37) | 5 [4-7] (34) | ||
WBC,/mm 3 | 15,185 ± 5,032 (87) | 15,166 ± 5,333 (59) | 15,224 ± 4,421 (28) | 12,847 ± 3,853 (37) | 12,961 ± 3,939 (34) | ||
CRP, mg/dL | 5 [0-30] (87) | 5 [0-30] (59) | 6 [1-18] (28) | 5 [1-29] (37) | 5 [1-29] (34) | ||
Albumin, g/dL | 3.9 [2.5-4.6] (86) | 3.9 [2.8-4.5] (59) | 3.9 [2.5-4.6] (27) | 3.9 ± 0.3 (37) | 3.9 ± 0.3 (34) | ||
ALT, IU/L | 25 [7-858] (87) | 22 [7-858] (59) | 44 [7-634] (28) | 28 [5-667] (37) | 29 [5-667] (34) | ||
NT-proBNP, pg/mL | 473 [30-7,736] (85) | 416 [30-3,703] (58) | 536 [76-7,736] (27) | 303 [24-1,667] (37) | 296 [24-1,667] (34) |
Echocardiographic Parameters
The echocardiographic parameters of all aKD and controls are shown in Table 2 . All aKD were in sinus rhythm and had LV EF ≥50%. None had severe MR. PALS in all aKD were decreased compared with those in controls ( Figure 2 A and 2B). In all aKD, E/A and LAVImax did not differ compared with those in controls. In the MR (−) LVSD (−) aKD group, E/E′ was increased as compared with in the controls ( Figure 2 B). No difference in PALS and E/E′ was observed between the MR (−) LVSD (−) aKD and OtheraKD groups ( Figure 2 B). LV longitudinal peak systolic ε, and SR in all aKD were decreased compared with those in controls. Representative examples of PALS in the MR (−) LVSD (−) aKD and OtheraKD groups and their convalescent examples are shown in Figure 3 .
Derivation cohort | Validation cohort | ||||||
---|---|---|---|---|---|---|---|
Variable | CTRL | All aKD | MR (−) LVSD (−) aKD | OtheraKD | CTRL | All aKD | MR (−) LVSD (−) aKD |
LVEF (%) | 67 ± 8 (66) | 63 ± 9 (92) ∗ | 63 ± 9 (62) | 62 ± 10 (30) ∗ | 66 ± 6 (19) | 66 ± 5 (37) | 66 ± 6 (34) |
HR, bpm | 111 ± 21 (67) | 108 [76-188] (95) | 108 [76-183] (65) | 108 [83-188] (30) | 106 ± 21 (19) | 110 ± 22 (37) | 113 ± 21 (34) |
LVMI, g/m 2 | 57 ± 14 (61) | 63 ± 14 (90) ∗ | 62 ± 12 (60) ∗ | 65 ± 17 (30) ∗ | 57 ± 14 (19) | 61 [21-79] (37) | 61 [21-79] (34) |
E/A | 1.6 [0.7-3.8] (60) | 1.6 [0.9-2.8] (93) | 1.6 [1.1-2.8] (63) | 1.7 ± 0.5 (30) | 1.6 [1.0-3.9] (19) | 1.5 [0.8-2.8] (37) | 1.5 [0.8-2.8] (34) |
E/E′ | 7 ± 2 (59) | 7 [3-20] (91) | 8 [4-20] (63) ∗ | 6 [3-14] (28) | 6 [4-12] (19) | 7 ± 2 (37) | 7 ± 2 (34) |
PALS (%) | 47 [32-73] (67) | 41 [19-72] (95) ∗ | 41 [22-72] (65) ∗ | 41 ± 10 (30) ∗ | 50 ± 10 (19) | 40 [28-70] (37) ∗ | 40 [28-70] (34) ∗ |
LAVImax, mL/m 2 | 24 [12-49] (62) | 26 [11-60] (93) | 25 [11-54] (63) | 29 ± 11 (30) | 20 ± 5 (19) | 25 ± 6 (37) ∗ | 25 ± 6 (34) ∗ |
LV longitudinal peak systolic ε (%) | 24 ± 3 (67) | 21 ± 4 (95) ∗ | 22 ± 3 (65) ∗ , † | 16 [11-29] (30) ∗ | 24 ± 3 (19) | 24 ± 3 (37) | 24 ± 4 (34) |
LV longitudinal peak systolic SR, s −1 | 1.7 ± 0.3 (67) | 1.4 [0.9-3.2] (95) ∗ | 1.5 [1.0-3.2] (65) ∗ , † | 1.3 [0.9-2.1] (30) ∗ | 1.7 ± 0.2 (19) | 1.6 [1.2-2.7] (37) | 1.7 ± 0.3 (34) |
∗ P < .05 when compared with controls.
Clinical Utility of PALS for Differentiating aKD from Controls
ROC curve analysis showed that, for the differentiation of all aKD and MR (−) LVSD (−) aKD from controls, PALS outperformed E/E′, E/A, and LAVImax ( Figure 4 ) but showed a low sensitivity and poor discriminative ability, like E/E′, E/A, and LAVImax ( Table 3 ).
All aKD | MR (−) LVSD (−) aKD | |||||||
---|---|---|---|---|---|---|---|---|
Variable | PALS, % | E/E′ | E/A | LAVImax, mL/m 2 | PALS, % | E/E′ | E/A | LAVImax, mL/m 2 |
Cutoff value | ≤40 | >7 | >1.3 | >20 | ≤39 | >7 | >1.3 | >20 |
Derivation cohort | ||||||||
Sensitivity, % | 49.5 | 46.2 | 78.5 | 79.6 | 47.7 | 49.2 | 76.2 | 79.4 |
Specificity, % | 80.6 | 67.8 | 31.7 | 33.9 | 83.6 | 67.8 | 31.7 | 33.9 |
Validation cohort | ||||||||
Sensitivity, % | 54.1 | 40.5 | 70.3 | 73.0 | 47.1 | 38.2 | 67.6 | 70.6 |
Specificity, % | 78.9 | 84.2 | 42.1 | 47.4 | 78.9 | 84.2 | 42.1 | 47.4 |
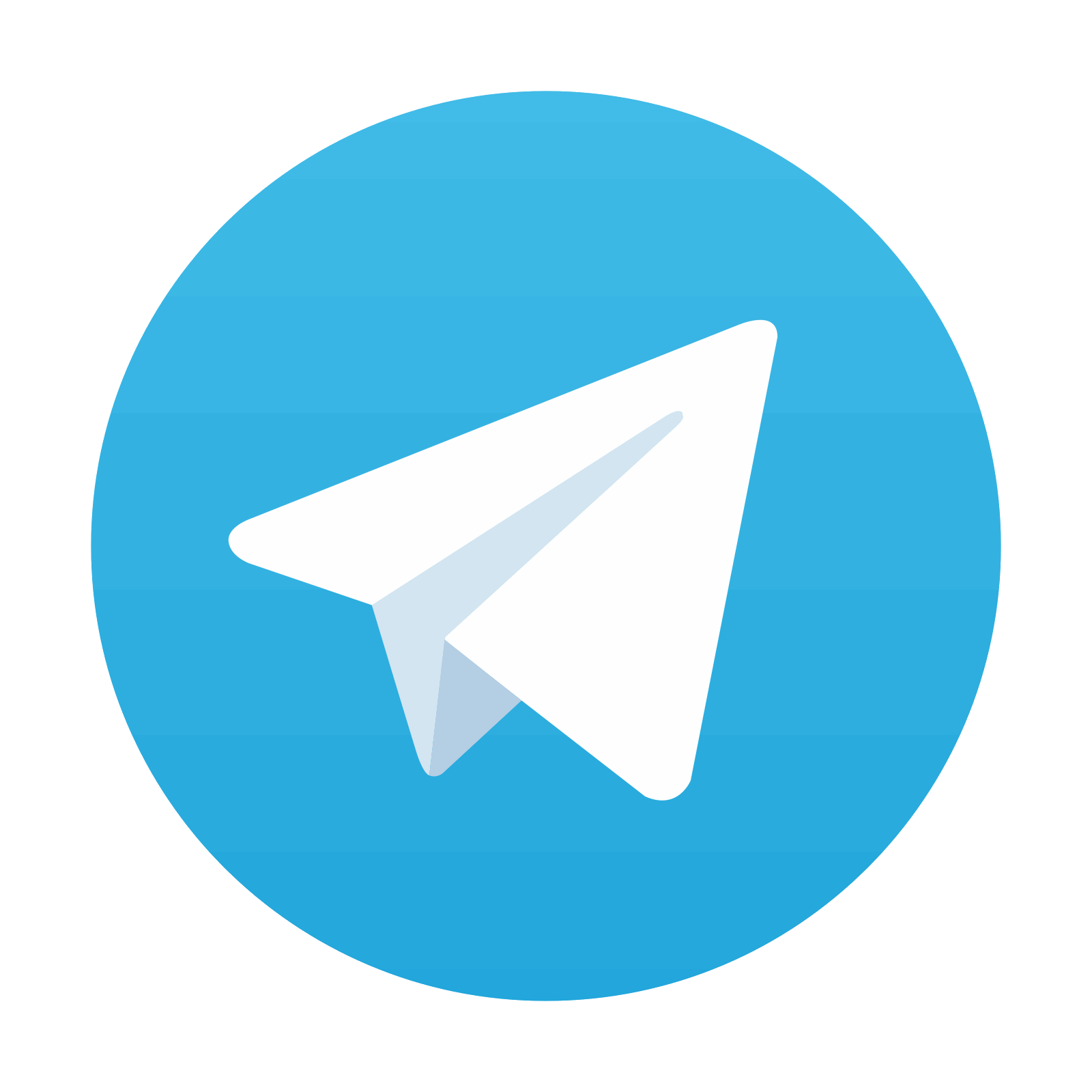
Stay updated, free articles. Join our Telegram channel

Full access? Get Clinical Tree
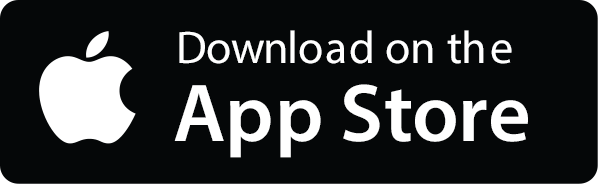
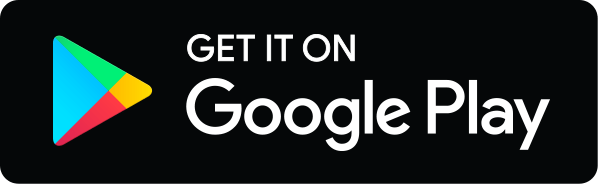
