Chronic Heart Failure in Children
Matthew J. O’Connor
Robert E. Shaddy
Introduction
Chronic heart failure is a clinical syndrome with diverse etiologies and broad variation in its clinical manifestations, all of which ultimately result from impaired ventricular filling or ejection of blood (1). Traditionally, chronic heart failure has been viewed as a syndrome of inadequate cardiac output to maintain end-organ perfusion during rest or exercise, and has historically been equated with reduced left ventricular ejection fraction. Newer definitions of chronic heart failure in adults aim to be more inclusive by incorporating disorders of ventricular filling, that is, diastolic dysfunction or heart failure with preserved ejection fraction (HFpEF) (2,3). In this chapter, the various causes of chronic heart failure in children will be described, along with their management and prognosis. Acute heart failure syndromes in the child, fetus, and adolescent, including myocarditis, as well as mechanical circulatory support and heart transplantation, will be discussed in further detail elsewhere within this textbook.
The topic of chronic heart failure in adults is of great significance for public health given its substantial incidence and burden on healthcare costs and utilization in developed countries, and is only expected to increase in coming years (4). While the comparative impact of pediatric chronic heart failure is much more limited relative to that of the adult syndrome, it is a resource-intensive disease (5,6) with higher rates of hospital-related mortality compared to adults, in addition to having high rates of hospital-associated morbidity (7). The treatment of chronic heart failure has recently been subject to comprehensive evidence-based review and recommendations by professional societies for both adults and children (1,8,9,10,11).
Nomenclature and Classification
The term “heart failure” is used in several contexts throughout the medical literature as well as common usage, and can hence be confusing to the novice practitioner. In clinical practice, heart failure is often defined on the basis of limitation in an individual’s tolerance for physical activities, combined with imaging (usually echocardiography, but increasingly cardiac MRI) evidence of depressed systemic ventricular function. This would be considered to be heart failure with reduced ejection fraction (HFrEF). In infants and young children, objective assessment of activity limitation can be difficult, so surrogate measures such as growth failure may be used in this patient population. However, “heart failure” may also be used to describe a constellation of acute signs and symptoms seen in conjunction with systemic ventricular dysfunction: left atrial hypertension, pulmonary edema, hepatomegaly, tachycardia, and gallop rhythm, without consideration given to assessment of cardiac output or ventricular function. Adding another layer of ambiguity to the definition of heart failure is the frequent application of this term to infants with large left-to-right intracardiac shunt lesions, who will often manifest the aforementioned symptom complex but have no evidence of systemic ventricular dysfunction; their symptoms merely resemble that of patients with systemic ventricular dysfunction due the common symptoms of left atrial hypertension, pulmonary vascular congestion, and systemic vascular congestion (hepatomegaly and peripheral edema). In this chapter, the term “chronic heart failure” will refer to the clinical syndrome of cardiac output inadequate to meet metabolic demand, that is, HFrEF, unless specified otherwise.
Several classification schemes have been proposed to define the clinical severity of chronic heart failure in children and adults, similar to the staging criteria utilized for various malignancies. The New York Heart Association (NYHA) functional classification remains in widespread use, but has limited application to young children. The Ross classification was developed to make the NYHA criteria more relevant to young children; recent revisions to the Ross classification incorporating biomarkers and stratifying children into five distinct age groups has been proposed (12). Recently, the American College of Cardiology Foundation and American Heart Association (ACCF/AHA) have proposed staging criteria for heart failure that incorporate functional and risk factor criteria (Table 73.1). Finally, with respect to ventricular function, heart failure has been classified into HFrEF and HFpEF. While the syndrome of HFpEF is an increasingly recognized and very common syndrome in adults, it is likely observed in children only in the setting of restrictive and/or hypertrophic cardiomyopathies, and will be discussed as part of those specific entities elsewhere in this textbook.
Etiology
An approach to understanding chronic heart failure in children begins with an investigation of the cause (Table 73.2). While there is no ideal classification scheme (see above), the etiology of chronic heart failure in children can best be considered by starting with two factors: the child’s age and the presence or absence of structural heart disease. For the purposes of this chapter, “structural heart disease” encompasses primary anatomic cardiac abnormalities such as congenital heart disease, valvar stenosis and/or regurgitation independent of a congenital abnormality, and coronary artery disease. This is in distinction to cardiomyopathies and pericardial disease, which primarily affect cardiac muscle and the pericardium, respectively. Broadly speaking, from the standpoint of age, children can be divided into two broad groups: neonates/infants (<1 year of age) and children/adolescents; the etiologies of chronic heart failure in these populations tend to be distinct, although overlap certainly exists.
Neonates and Infants
The neonate or infant with heart failure typically presents for care after an episode of acute illness in which the diagnosis of heart failure is made, hemodynamic instability and symptoms are stabilized, and medical management is initiated. In some instances, the diagnosis is clear, although in a substantial proportion of cases, the diagnosis remains unclear and the child is left with the diagnosis of “idiopathic dilated cardiomyopathy.” Less commonly, the onset of symptoms can be more gradual, such that there is no acute presentation and the child is brought to clinical attention either incidentally or through seemingly unrelated symptoms.
TABLE 73.1 Classification Systems for Chronic Heart Failure | ||||||||
---|---|---|---|---|---|---|---|---|
|
TABLE 73.2 Etiology of Chronic Heart Failure | ||
---|---|---|
|
In either case, a logical and comprehensive approach to evaluation will ensure that important diagnoses are not missed while avoiding unnecessary and possibly risky testing. Broadly speaking, potential etiologies of chronic heart failure in neonates and infants can be grouped under infectious, inflammatory, toxic, structural/congenital, metabolic, arrhythmogenic, and idiopathic. The differential diagnosis for the unusual presentation of heart failure at the time of birth includes birth asphyxia with myocardial dysfunction from hypoxemia, hypoglycemia, hypocalcemia, sepsis, anemia or polycythemia, myocarditis, arrhythmias (congenital complete heart block, supraventricular tachyarrhythmias), large arteriovenous malformations, severe atrioventricular valve regurgitation, or Ebstein abnormality of the tricuspid valve (13).
Essentially any form of congenital heart disease can precipitate ventricular dysfunction, although ventricular dysfunction with resultant symptoms of low cardiac output are generally restricted to the following physiologic derangements: (a) obstructive lesions (i.e., critical aortic stenosis, hypoplastic left heart syndrome); (b) volume overload lesions with excessive left-to-right shunting (i.e., ventricular septal defect, patent ductus arteriosus, aortopulmonary window, common atrioventricular septal defect); and (c) regurgitant lesions (i.e., truncus arteriosus with severe truncal regurgitation, severe Ebstein abnormality). Much of what is termed “critical” congenital heart disease consists of defects within the obstructive lesion group, in which severe hypoplasia or atresia of left heart structures and/or the aorta leads to inadequacy of cardiac output upon closure of the ductus arteriosus. Without maintenance of ductal patency with prostaglandin E1, affected infants usually present within the first 1 to 2 weeks of life with signs and symptoms of low cardiac output, including but not limited to pallor, poor feeding, weak cry, acidosis, shock, and varying degrees of cyanosis due to intracardiac mixing. The advent of pulse oximetry screening has made it possible to identify readily infants with ductal-dependent cyanotic congenital heart disease who may otherwise escape detection before discharge from the newborn nursery (14); the effects on infant outcomes of a nationwide implementation of this practice remain unclear, but at least appear to be cost effective (15,16,17).
The clinical presentation of volume overload lesions depends upon the size of the defect and the ratio of pulmonary to systemic vascular resistance. During the neonatal period, the ratio may be nearly equal, leading to a well-balanced circulation and little net left-to-right shunt. However, as pulmonary vascular resistance falls normally with age, the proportion of pulmonary blood flow increases, with a decrease in the ratio of systemic blood flow. This progressive net left-to-right shunt may lead to impaired cardiac output (18), which may be accentuated by periods of increased metabolic demand (fever) or by the physiologic fall in hemoglobin (19).
The prototypic regurgitant lesion causing symptoms of a low cardiac output and heart failure in the neonate and/or infant is Ebstein anomaly of the tricuspid valve. The anatomy and physiology of this lesion is described in detail in Chapter 38 of this textbook. When severe, the combination of significant tricuspid regurgitation and functional right ventricular hypoplasia leads to a state in which the right ventricle is unable to generate sufficient forward output. In the absence of the ductus arteriosus, severe cyanosis will ensue. Frequently, however, the pulmonary valve in Ebstein abnormality is incompetent, leading to an inefficient cycle in which deoxygenated blood is recirculated through the heart, leading to worsening cyanosis even in the setting of ductal patency (20). This sets off a vicious cycle, sometimes termed a “circular shunt,” in which cyanosis and low cardiac output are intertwined.
Many complex congenital abnormalities are associated with both obstruction and volume overload, which may result in a combination of heart failure and cyanosis. Patients with single ventricle physiology, will frequently fall into this category. The specific manifestations of heart failure and cyanosis, and their severity, depend on the balance of systemic and pulmonary vascular resistance as well as the specific anatomic characteristics of the defect. As an example, in tricuspid atresia with normally related great vessels, a large muscular ventricular septal defect, and no pulmonary stenosis, the patient may require no interventions as a neonate but eventually require an additional source of pulmonary blood flow later in infancy due to progressive cyanosis due to the development of restriction at the ventricular septal defect. The numerous categories and variations of the complex defects capable of causing both pressure and volume overload are discussed in detail in their respective chapters.
An important congenital heart defect that may mimic a dilated cardiomyopathy in newborns is anomalous origin of the left coronary artery from the pulmonary artery (ALCAPA) (21,22). The anatomy, physiology, and treatment of this lesion are described in detail in Chapter 32. Its clinical presentation is dependent upon the normal fall in pulmonary vascular resistance; as pulmonary vascular resistance and pressure fall, perfusion pressure in the left coronary artery falls in concert. This leads to the gradual development of myocardial ischemia in the regions supplied by the left coronary artery, with symptoms typically becoming manifest between 1 week and 6 months of age. Recognition of this defect as the underlying cause of ventricular dysfunction is of paramount importance because the resulting heart failure syndrome may be largely reversed by prompt surgical revascularization of the ischemic myocardium supplied by the normal left coronary artery distribution (23,24,25). Misguided attempts at prolonged medical management may worsen the underlying physiology, lose important time in rescuing ischemic myocardium, and ultimately provide no long-term benefit to the patient.
Myocarditis and all of the various cardiomyopathies (dilated, hypertrophic, restrictive, noncompaction, arrhythmogenic right ventricular dysplasia, tachycardia-induced) may present during the neonatal period or infancy with symptoms of heart failure, typically directly related to ventricular dysfunction. Following hemodynamic stabilization, treatment and prognosis are frequently directed by the underlying diagnosis. In neonates and infants, a low index of suspicion should be maintained for cardiomyopathies associated with inborn errors of metabolism, including mitochondrial disease, until consultation with an expert in metabolic disorders is obtained (26,27,28). Some practitioners advocate empiric carnitine supplementation for all suspected metabolic cardiomyopathies, or at least until the diagnosis of carnitine deficiency is excluded, although the benefits of these practices are questionable (29).
Childhood and Adolescence
Structurally Abnormal Hearts
Although presentation with heart failure because of unoperated congenital heart disease is uncommon beyond the first few months of life in developed countries, it is more common in developing countries with poor access to health care (30,31). It is useful to subdivide heart failure in this age group into left versus right heart failure.
Left heart failure may occur in patients with structural heart disease and severe atrioventricular valve insufficiency (i.e., atrioventricular septal defects or the tricuspid valve in congenitally corrected transposition) (32,33) or aortic valve insufficiency (i.e., ventricular septal defect-associated aortic valve prolapse) (34,35). Although progression of valvar regurgitation may occur spontaneously over time, infective endocarditis is an important etiology of altered valvar function in this group of patients (36). Less commonly, patients with either progressive or undiagnosed severe left ventricular outflow tract obstruction may present with heart failure due to ventricular dysfunction (37).
An important cause of right heart failure in this group of patients is Ebstein anomaly of the tricuspid valve with an increase in the degree of tricuspid regurgitation with or without associated arrhythmias (38,39). Severe elevation of pulmonary vascular resistance associated with an intracardiac shunt or great vessel shunt
(i.e., Eisenmenger syndrome) may be associated with right ventricular dysfunction, tricuspid and/or pulmonary regurgitation, and right heart failure (40,41).
(i.e., Eisenmenger syndrome) may be associated with right ventricular dysfunction, tricuspid and/or pulmonary regurgitation, and right heart failure (40,41).
Postoperative Structural Heart Disease
More common is the patient who has undergone previous intervention (transcatheter or surgical) for structural heart disease. Such patients may develop left or right heart failure due to ventricular dysfunction, valve disease, residual shunts, and/or rhythm abnormalities (32,42).
Left heart failure may occur in the postoperative patient with ventricular dysfunction, a residual left-to-right shunt, outflow tract obstruction, or valve regurgitation (various combinations of these may coexist in any given patient). Examples include (a) aortic valve stenosis and/or insufficiency after previous surgical valvotomy (43,44,45) or balloon valvuloplasty (46,47,48), (b) truncal valve stenosis and/or regurgitation after repair (45,49,50,51), and (c) severe atrioventricular valve regurgitation after atrioventricular septal defect repair (52,53,54). Patients with a systemic right ventricle (i.e., transposition of the great arteries after Senning’s or Mustard’s atrial switch repair or congenitally corrected transposition of the great arteries after repair) are at risk for ventricular dysfunction, tricuspid (systemic atrioventricular valve) regurgitation, and arrhythmias that may result in heart failure (55,56,57,58,59,60,61,62,63,64).
Right ventricular hypertension owing to pulmonary hypertension (65) or residual pulmonary outflow tract obstruction (native or conduit) (66,67,68) may progress after initial intervention and result in right heart failure. Right heart failure may also occur after previous intervention owing to significant pulmonary insufficiency, such as may occur after surgery for tetralogy of Fallot (69,70,71). A promising new therapy in the treatment of residual right ventricular outflow tract disease is transcatheter pulmonary valve replacement, which allows a less invasive method of restoring pulmonary valve competence and reducing obstruction without exposing the patient to cardiopulmonary bypass (72,73,74). The long-term effects of this new treatment option on the evolution of right heart dysfunction in tetralogy of Fallot and related lesions remain to be seen.
Patients who have undergone a Fontan procedure represent another group at high risk for ventricular dysfunction, arrhythmias, and heart failure (63,75,76,77,78). Heart transplantation may offer the best opportunity for long-term survival in the population of Fontan patients in whom heart failure develops, as they frequently respond poorly to conventional medical and device therapy for heart failure. However, they appear to have poorer posttransplantation outcomes than patients without pre-existing Fontan physiology or congenital heart disease (79).
As stated previously, patients after transcatheter or surgical intervention for structural heart disease may develop either acute or chronic rhythm disturbances that may result in heart failure (77,80,81,82). Ventricular dyssynchrony, manifested by conduction delay on the electrocardiogram, may also contribute to symptomatic ventricular dysfunction. Efforts to restore ventricular electrical and mechanical ventricular synchrony through biventricular pacing are described later in this chapter.
Structurally Normal Heart and Acquired Heart Disease
In developing countries, rheumatic heart disease is the most common cause of acquired heart disease in children and young adults, manifesting as carditis in the acute phase of illness (83,84). Acute rheumatic fever is uncommon in the United States, although intermittent outbreaks of the disease continue to occur in clusters (85). The carditis that occurs in rheumatic heart disease is more properly termed as valvitis, as myocardial involvement does not occur (86,87,88). Typically the mitral and/or aortic valves are affected. Acute insufficiency of one or either of these valves may lead to acute ventricular dilation and symptoms of heart failure from acute changes in ventricular loading conditions in 15% to 47% of patients (89,90,91). Valvar stenosis and/or insufficiency develops with chronic rheumatic heart disease, with a low incidence of involvement of the tricuspid and/or pulmonary valves (92). Heart failure may result from progressive valvar dysfunction (83,92,93,94). Less commonly, left ventricular dysfunction may develop secondary to chronic severe volume overload if significant mitral and/or aortic regurgitation are present. Although rheumatic mitral stenosis severe enough to result in symptomatic heart failure may occur in the first two decades of life in developing countries (95), chronic rheumatic heart disease with mitral stenosis in the United States typically presents in the fourth to fifth decade of life (83).
Kawasaki disease is an important cause of acquired heart disease in developed countries and is the most common cause of acquired heart disease in children in the United States (96). Children less than 5 years are most commonly affected, with a median age of 2 years at diagnosis. Untreated, approximately 25% of children will develop coronary artery aneurysms or ectasia; administration of intravenous immune globulin (IVIg) reduces the risk to <5% (97,98). Another hallmark feature of Kawasaki disease is that nearly all affected individuals develop a pancarditis, which may manifest with echocardiographic evidence of ventricular dysfunction and/or symptomatic heart failure (99). Long-term survivors of Kawasaki disease with residual coronary disease are at risk for ischemic heart disease, myocardial infarction, and valvular heart disease in long-term follow-up (98); however, in the more recent era in which a higher proportion of patients routinely receive IVIg and antiplatelet therapy in the early stages of illness, the risks of these complications may be lower on the basis of recent epidemiologic (100) and advanced imaging (101) studies. Whether the results of a recent randomized trial of infliximab, the monoclonal antibody to tumor necrosis factor-alpha (TNF-α), in Kawasaki disease, in which significant reductions in left anterior descending diameter Z scores were seen 2 weeks after randomization, will translate into improved longer-term coronary artery outcomes in Kawasaki remains unclear (102).
Infective endocarditis may also cause heart failure in older children with structurally normal hearts, although the incidence of endocarditis is significantly less than that observed in patients with unrepaired or palliated congenital heart disease with residual intracardiac shunts, who represent the highest risk for endocarditis (103,104). Increasing risk-taking behaviors (i.e., intravenous drug use, tattooing) in the adolescent age population account for the additional risk compared to younger children. However, chronically ill and medically complex children with indwelling central catheters, ventriculoperitoneal shunts, and other similar devices are at risk for bacteremia that may lead to endocarditis. Acute infective endocarditis results in acute valvar dysfunction, typically regurgitation, with adverse loading conditions imposed by acute regurgitation leading to ventricular dilation and impaired forward cardiac output. In the setting of bacteremia, a septic shock scenario may ensue with release of cytokines and other vasoactive peptides that may further depress cardiac contractility.
Apart from the entities above, myocardial dysfunction in structurally normal hearts may be due to a wide array of etiologies, but the clinical presentation is generally that of clinical heart failure regardless of etiology. Significant overlap exists between the clinical presentations of heart failure in patients with myocarditis and dilated cardiomyopathies. In many cases, dilated cardiomyopathies that are labeled as “idiopathic” may be residual complication of an episode of acute myocarditis (105). Infectious agents are common triggers of myocarditis, with viruses, bacteria, protozoa, and fungi
all potential etiologies (106,107). In the United States, viral etiologies predominate, with adenoviruses, coxsackieviruses, enteroviruses, and parvoviruses being frequently identified viral genomes in cardiac biopsy specimens with myocarditis. However, other important causes of myocarditis include toxins, ethanol, giant cell myocarditis, and systemic inflammatory processes such as Kawasaki disease and connective tissue disease, notably systemic lupus erythematosus (108,109). In cases of idiopathic dilated cardiomyopathies, obtaining an extended family history is important, as many (20% to 35%) cases may be familial in nature (110). Other cardiomyopathies less likely but not incapable of producing a clinical heart failure syndrome include the hypertrophic (111,112), restrictive (113), noncompaction (114), and arrhythmogenic right ventricular dysplasia (115) cardiomyopathies. Finally, tachycardia-induced cardiomyopathies may present with clinical heart failure (116), although this is less common than in the infant age group as symptoms may brought to attention before the development of myocardial dysfunction. Incessant supraventricular tachyarrhythmias such as permanent junctional ectopic tachycardia (PJRT) are an example of such a tachyarrhythmia (117).
all potential etiologies (106,107). In the United States, viral etiologies predominate, with adenoviruses, coxsackieviruses, enteroviruses, and parvoviruses being frequently identified viral genomes in cardiac biopsy specimens with myocarditis. However, other important causes of myocarditis include toxins, ethanol, giant cell myocarditis, and systemic inflammatory processes such as Kawasaki disease and connective tissue disease, notably systemic lupus erythematosus (108,109). In cases of idiopathic dilated cardiomyopathies, obtaining an extended family history is important, as many (20% to 35%) cases may be familial in nature (110). Other cardiomyopathies less likely but not incapable of producing a clinical heart failure syndrome include the hypertrophic (111,112), restrictive (113), noncompaction (114), and arrhythmogenic right ventricular dysplasia (115) cardiomyopathies. Finally, tachycardia-induced cardiomyopathies may present with clinical heart failure (116), although this is less common than in the infant age group as symptoms may brought to attention before the development of myocardial dysfunction. Incessant supraventricular tachyarrhythmias such as permanent junctional ectopic tachycardia (PJRT) are an example of such a tachyarrhythmia (117).
Children treated for various malignancies with anthracycline chemotherapy (doxorubicin, daunorubicin) are at a lifelong risk for the development of cardiotoxicity, which most commonly manifests as a dilated cardiomyopathy (118). Risk factors for the development of cardiotoxicity include young age at receipt of chemotherapy, higher cumulative total dose, and concomitant receipt of radiation to the chest. Awareness of the cardiotoxicity of anthracycline chemotherapy has been known for years; as such, efforts have been made for some time to minimize anthracycline exposure. In general, the lifetime risk of developing any degree of cardiotoxicity from anthracycline chemotherapy during childhood is approximately 10%. For most patients, the degree of myocardial dysfunction is mild, and most patients are without symptoms. Nonetheless, patients remain at risk for many years following completion of chemotherapy and declaration of cure of their malignancy, so ongoing surveillance and vigilance are warranted. For those who have developed cardiotoxicity, standard heart failure medical management is typically utilized, although there is little evidence to suggest that specific agents are superior to one another for this particular entity (see subsection “Angiotensin-Converting Enzyme Inhibitors and Angiotensin-Receptor Blockers”).
Other causes of heart failure in children and adolescents are worth noting. Patients with Marfan syndrome with mitral valve prolapse may develop heart failure if the mitral valve becomes progressively insufficient or aortic regurgitation progresses because of the progressive aortic root dilation (119). New therapies showing promise in Marfan syndrome include beta-blockers and angiotensin-receptor blockers (ARBs), which may abrogate the progressive aortopathy seen in this disease (120,121). Sickle cell disease may be associated with myocardial ischemia or infarction (122), although overt systolic dysfunction is uncommon in children (123,124). Renal dysfunction with systemic hypertension and fluid overload may result in left ventricular dysfunction (125,126). The classic entity of “cor pulmonale” or right heart failure from pulmonary pathology is relatively uncommon in children, in comparison to adults. However, overt right ventricular failure or precursors to right ventricular failure such as abnormalities of right ventricular diastolic function may be seen in pulmonary hypertension (65,127,128) or chronic lung diseases (129,130,131).
Biomarkers in Chronic Heart Failure
Cardiac biomarkers can be defined as any substance measured in the blood (other than traditional laboratory-based tests) that provides information related to the cardiovascular system (132). Biomarkers have assumed a prominent role in the diagnosis and management of chronic heart failure. The ideal biomarker would have the following properties: (a) is specific for the myocardium; (b) is released in quantities sufficient to be detected by an assay with sufficient sensitivity and specificity to distinguish between healthy and disease states; (c) a relationship can be determined between the serum level of the biomarker and the clinical severity of the disease state; and (d) can provide diagnostic and prognostic information. Biomarkers in chronic heart failure have been identified which potentially meet some or all of these criteria, including those associated with hemodynamic abnormalities, myocardial inflammation, myocardial fibrosis, myocardial necrosis, and remodeling (1). The ideal biomarker in heart failure would provide diagnostic and prognostic information; however, to date no biomarkers have clearly been able to do so with sufficient sensitivity and specificity despite a proliferation of potential biomarkers in the literature over the past decade (133).
The prototypical and still most useful cardiac biomarker is B-natriuretic peptide (BNP), also (historically) known as brain natriuretic peptide. BNP is one of four natriuretic peptides: ANP, BNP, CNP, and DNP. ANP is primarily released from the atria, BNP from ventricular myocardium, CNP from vascular endothelium, and DNP from ventricular myocardium (134,135). BNP and its inactive processed product, N-terminal-pro-BNP (NT-proBNP), are released from ventricular myocardium in response to increased myocardial fiber stretch. BNP and NT-proBNP have different biochemical properties, are measured by different assays, and can cause therapeutic confusion if values for each are unwittingly compared for an individual patient. NT-proBNP has a longer half-life in plasma than BNP; therefore NT-proBNP levels are generally higher than BNP and display less variability. NT-proBNP also appears to be more affected by renal function than BNP (134). The primary action of BNP is to respond to intravascular volume expansion. BNP acts upon the distal tubule in the kidney to secrete sodium ions, which results in the transit of free water into the distal tubule for excretion. However, as will be shown to be of importance as a therapeutic target in chronic heart failure therapy below, BNP also affects smooth muscle relaxation with improved myocardial relaxation and vasodilation of the systemic, pulmonary, and coronary vascular beds. In addition, in the kidneys, BNP and the other natriuretic peptides inhibit the renin–angiotensin–aldosterone (RAA) system, play a role in blunting the sympathetic nervous system, and inhibit endothelin release, thereby contributing to vasodilation, diuresis, and natriuresis (135,136). They also appear to have antifibrotic properties as well as other properties that contribute to processes that mitigate the adverse myocardial remodeling that occurs in chronic heart failure (137). Normal values for BNP and NT-proBNP vary with age and gender, with levels rising with age and women having higher levels than men after controlling for age. BNP levels are very high in newborns, peaking at 1 to 2 days of age and falling thereafter (138). Obesity is known to depress BNP levels (134); this relationship appears to hold true in children as well (139). Normative values for BNP and NT-proBNP are available (140,141,142).
Validated extensively in adults, BNP is abnormally elevated in acute and chronic heart failure. BNP correlates with heart failure severity and prognosis (143,144). BNP is frequently used in acute care scenarios such as the emergency department to distinguish cardiac from noncardiac causes of dyspnea in adults (i.e., pneumonia, asthma) (145). Similar data regarding the discriminating ability of BNP exist for children, albeit on a smaller scale (146,147). BNP values have also been used as an adjunct to discriminate between critical congenital heart disease and noncardiac conditions in infants (148). In children, elevations in BNP and/or NT-proBNP have been associated with the presence of intracardiac left-to-right shunt lesions (149,150,151), adverse events in patients with left ventricular dysfunction (152,153,154), lack of recovery in patients with myocarditis (155), recipients of anthracycline chemotherapy, with or without ventricular dysfunction (156,157,158,159,160), disease severity in hypertrophic cardiomyopathy, (161) cardiac rejection (162,163), pulmonary hypertension (164,165), and Kawasaki disease recalcitrant to IVIg
therapy (166,167) (Table 73.3). BNP and NT-proBNP have also been studied in patients with single ventricle physiology (168,169), where elevations in NT-proBNP may have particular utility in predicting the development of clinical heart failure in patients with a single ventricle of right ventricular morphology (169). In a post-hoc analysis of the prospective Pediatric Carvedilol Trial (170) (see below), in children with moderately symptomatic heart failure, a BNP ≥140 pg/mL identified patients at a higher risk of poor outcomes (152). Threshold BNP and NT-proBNP values suggestive of cardiac disease versus other etiologies are presented in Table 73.4.
therapy (166,167) (Table 73.3). BNP and NT-proBNP have also been studied in patients with single ventricle physiology (168,169), where elevations in NT-proBNP may have particular utility in predicting the development of clinical heart failure in patients with a single ventricle of right ventricular morphology (169). In a post-hoc analysis of the prospective Pediatric Carvedilol Trial (170) (see below), in children with moderately symptomatic heart failure, a BNP ≥140 pg/mL identified patients at a higher risk of poor outcomes (152). Threshold BNP and NT-proBNP values suggestive of cardiac disease versus other etiologies are presented in Table 73.4.
TABLE 73.3 Serum Concentrations of BNP and NT-proBNP Suggestive of Cardiac Disease in Children | ||||||||||||||||||||||||||||||||
---|---|---|---|---|---|---|---|---|---|---|---|---|---|---|---|---|---|---|---|---|---|---|---|---|---|---|---|---|---|---|---|---|
|
The wide array of conditions associated with abnormally elevated BNP levels is indicative of its strength as an assay to rule out cardiac disease, rather than to rule in cardiac disease. The use of BNP as a biomarker has been extended into the realm of cardiac surgery in children, as a prediction tool of acute kidney injury (171,172) and other adverse outcomes following cardiac surgery, with mixed results (173,174). Other commonly encountered conditions in which BNP and/or NT-proBNP levels may be elevated include end-stage renal disease (175), sepsis (176), and brain death (177).
TABLE 73.4 Clinical Utility of Natriuretic Peptide Levels in Children | ||||||||||
---|---|---|---|---|---|---|---|---|---|---|
|
In adults, attempts have been made to utilize BNP as a guide to direct therapy in heart failure. It is unclear as to whether BNP is a reliable guide of effective heart failure management, and current heart failure guidelines do not recommend serial BNP measurement to guide heart failure therapy (1). The ongoing prospective Guiding Evidence Based Therapy Using Biomarker Intensified Treatment in Heart Failure (GUIDE-IT) study is evaluating whether adjusting heart failure therapy in patients with moderate heart failure (ejection fraction ≤40%) to achieve a target NT-proBNP of <1,000 pg/mL is superior to current guideline-directed medical therapy at attaining the primary endpoint of cardiovascular death or first hospitalization for heart failure (178).
The greatest value of BNP as a biomarker likely lies in its serial measurement, with changes over time having greater predictive value than an individual measurement in adult studies (179). The data regarding the predictive value of serial BNP measurements in children are less clear but some studies are illustrative. For example, in a study of hospitalized children with acute decompensated heart failure, absolute NT-proBNP values at admission did not predict heart failure severity or the eventual need for mechanical circulatory support, while the rate of decrease in NT-proBNP levels was greater in those patients who did not require mechanical support during the hospitalization compared to those who did require mechanical circulatory support (180).
Other biomarkers are available, but are not as widely used in clinical practice as the natriuretic peptides. Examples include ST2 and galectin-3, among several others (181). Some biomarkers are more conventionally regarded as indicators of inflammation, but can be properly regarded as cardiac biomarkers as well. These include troponin and C-reactive protein (CRP) as biomarkers of cardiac damage and inflammation, respectively. Studies of troponin as a biomarker in pediatric heart failure are generally limited to use of this biomarker for the detection of anthracycline cardiotoxicity, with mixed results (160,182). High-sensitivity CRP assays have been studied extensively in adults, mainly as a measure of low-level inflammation associated with coronary artery disease; evaluations of their utility in pediatric heart failure are limited to exploratory studies (183,184).
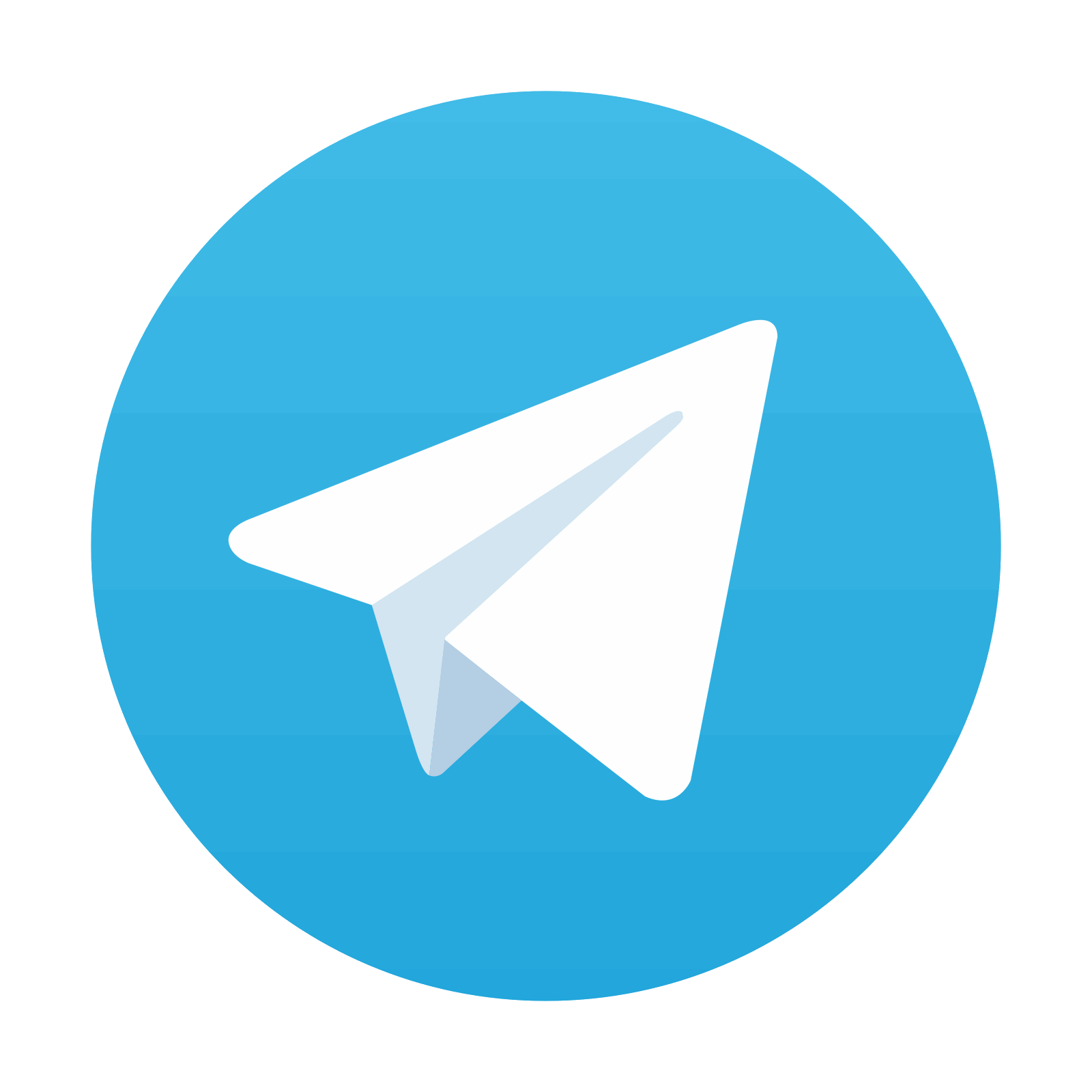
Stay updated, free articles. Join our Telegram channel

Full access? Get Clinical Tree
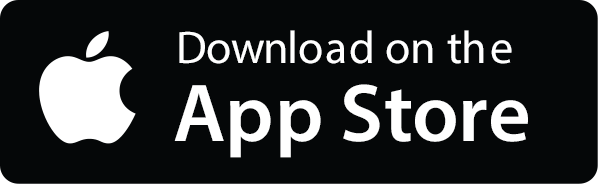
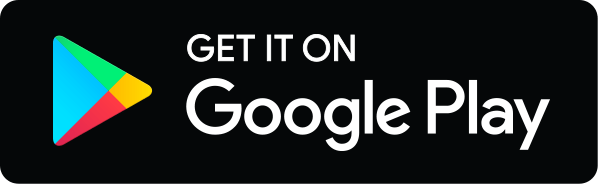