Much of what is known about the effect of high altitude on humans, including the physiologic responses to hypoxia and susceptibility to acute altitude illness, is based on studies of fit young individuals, while few studies have addressed these issues as they pertain to children or elderly individuals. In addition, while the physiologic responses to hypoxia and risks for and management of acute altitude illness are generally the same in women as for men, there are several unique issues for women traveling to high altitude including hormonal changes over the course of the menstrual cycle, menopause, and pregnancy, which may affect responses relative to men. The goal of this chapter is to consider each of these groups of people in greater detail. The focus throughout will be on unacclimatized lowlanders traveling to high altitude. Issues pertaining to high altitude residents are considered in Chapters 4, 6, and 24. The increased accessibility of the mountainous regions of the world to adults means that more children are now being taken to high altitude for a variety of reasons. Infants have been carried over 6000 m peaks in Nepal (Pollard et al. 1998), for example, while lowland Han Chinese children are taken to high altitude in Tibet and Qinghai. Issues associated with travel to high altitude in children of varying ages, which has been reviewed in detail elsewhere (Pollard et al. 2001; Yaron and Niermeyer 2008), are considered below. The physiologic responses to acute hypoxia vary based on the age of the child. There are several unique physiological issues regarding high altitude exposure in the neonate and very young infants. Following birth, the low flow, high resistance fetal circulation transitions over a variable time period to the high flow, low resistance adult circulation with closure of the ductus arteriosus, serving as a key step in that process. Hypoxic exposure during this period can slow and possibly reverse this circulatory transition, making oxygenation difficult for the infant, particularly now that the placenta is no longer available for gas exchange (Niermeyer 2007). For this reason, prolonged exposure to hypoxia is not recommended within the first four to six months of life, although short airline flights are likely okay (Niermeyer 2007). Immaturity of respiratory control mechanisms may also pose challenges for the neonate and young infant at high altitude. In adults, hypoxia stimulates an increase in ventilation, whereas in neonates and young infants, hypoxia can have a depressant effect. This pattern usually resolves after four to six weeks, but a study by Parkins et al. (1998) suggests the issue can persist for longer than that time frame. They exposed infants between 1 month and 6 months of age (mean age 3.1 months) to an FIO2 of 0.15 and found a significant decrease in the time spent in a regular breathing pattern and a 3.5-fold increase in the time spent in periodic breathing. While the mean saturation for all infants during this exposure was 92.8%, the responses were highly variable, with some infants experiencing oxygen saturations below 80% for up to a minute, at which time the intervention was stopped. Even greater degrees of hypoxemia may be seen in those with a history of neonatal lung disease. Udomittipong et al. (2006), for example, exposed 47 infants (median corrected age 1.4 months) with a history of neonatal lung disease but who no longer required supplemental oxygen to an FIO2 of 0.14–0.15 for 20 minutes and found that 81% experienced falls in their oxygen saturation below 85%. These issues appear to resolve as infants age beyond 3 months. Yaron et al. (2003) took infants aged 3–36 months who normally resided in Denver, Colorado (1610 m), to 3109 m and, after 24 hours at that altitude, noted an increase in respiratory rate from 45 ± 13 to 51 ± 9 breaths/min, a decrease in the end-tidal PCO2 from 31 ± 3 mmHg to 28 ± 2 mmHg, and a reduction in SpO2 from 95 ± 2% to 91 ± 2%. Brain tissue oxygenation, estimated using near-infrared spectroscopy, fell from 78 ± 8% to 67 ± 13%, with the lower saturations seen in the younger children. Data were not presented for the adults who accompanied the children, but the authors reported that the changes observed in the infants were similar to those seen in adults. A few studies have examined physiologic responses to high altitude in older children. Scrase et al. (2009), for example, studied nine children ages 6–13 years during a trek to 3500 m in Nepal and found that, compared to their sea-level baseline, at 3500 m, average heart rate was 24 beats min−1 higher while daytime end-tidal PCO2 was 7 mmHg lower and daytime and nocturnal oxygen saturation were 9% and 11% lower, respectively. After nine days of acclimatization at 3500 m, oxygen saturation improved 3% on average, while end-tidal carbon dioxide fell by an additional 3 mmHg, changes indicative of ongoing ventilatory acclimatization. The responses seen in this study demonstrate a similar pattern to those reported in adults, but the study did not include direct comparisons with adult data. Such a comparison has been done by Kriemler et al. (2016) who examined 20 father-child pairs (average ages 44 ± 4 and 11 ± 1 years) at 450 m and then over a three-day stay at 3450 m. They found that the hypoxic ventilatory response (HVR) was higher in the children than the adults at 450 m, but increased to a similar extent in each group following ascent. Both groups experienced a roughly 20% decrease in peak oxygen consumption ( Figure 26.1Changes in hypoxic ventilatory response (HVR, panel A), peak oxygen uptake ( As with adults, children are susceptible to acute altitude illnesses, including AMS, HACE, and HAPE. In addition to considering whether the risk of these problems varies compared to adults, it is also important to recognize challenges that arise in the diagnosis of AMS in children as well as differences in the approach to prevention and treatment. The reported incidence of AMS in children varies across studies from 15% to 50% depending on the altitude at which AMS is assessed and the rate of ascent (Kriemler et al. 2008; Kriemler et al. 2016; Pradhan et al. 2009; Rexhaj et al. 2011; Theis et al. 1993; Yaron et al. 2002; Yaron et al. 1998). From the studies that also reported data from adults undertaking the same ascent profile as the children, the majority would suggest there are no significant differences in incidence between adults and children (Kriemler et al. 2008; Kriemler et al. 2016; Yaron et al. 2002; Yaron et al. 1998), although one study has reported markedly different rates despite similar ascent profiles (Rexhaj et al. 2011). It is worth noting that in many of the studies demonstrating similar incidence, the children were traveling with their biological parents and, as a result, the observed similarities in incidence rates may be due to the effect of heritable traits rather than being reflective of equal incidences across broader populations of unrelated children and adults. While the preponderance of studies suggests children and adults share the same susceptibility to AMS, the reproducibility of symptoms upon subsequent ascent may differ between the groups. Rexhaj et al. (2011) studied 27 unacclimatized children and 29 adults during two ascents to 3450 m nine to 12 months apart and found that none of the six children who developed AMS on the first ascent experienced this problem on repeat ascent, compared to 14 of 18 adults who had AMS with both exposures. Four children who remained symptom-free on the first ascent also developed AMS with the second exposure. In addition to AMS, children are also susceptible to the more severe forms of acute altitude illness. There are no official case reports of HACE in children in the literature, but a consensus statement on Children at High Altitude references several unpublished HACE cases encountered by one of the consensus committee members while a study examining AMS incidence in children undertaking a pilgrimage to Gosaikunda Lake in Nepal describes the presence of ataxia in one of their AMS cases, a finding indicative of HACE rather than simply AMS (Pradhan et al. 2009). HAPE has been more widely reported. Wu (1994), for example, reported an incidence of 1.51% among 464 children ascending by road to Lhasa, Tibet compared to 1.27% in adults. Respiratory infection (Durmowicz et al. 1997) and underlying cardiopulmonary abnormalities, such as pulmonary hypertension (Durmowicz 2001), may predispose to development of HAPE, although it can occur in the absence of these issues. Like adults, children residing at high altitude have also been noted to experience re-entry pulmonary edema, whereby pulmonary edema develops following rapid reascent to their home elevation after a sojourn to lower elevation (Scoggin et al. 1977). In fact, when Hultgren and Spickard (1960) first described HAPE in Peru, several of the cases were in children who had returned with their family to their high altitude residence after spending time at sea level. Recently, Ebert-Santos (2017) has reported development of a clinical syndrome consistent with HAPE in children despite a lack of recent travel to and from a lower elevation. Whether this entity shares the same underlying pathophysiology as HAPE is unclear at this time. While examination findings and diagnostic studies can be used to confirm the presence of HACE or HAPE, the diagnosis of AMS is based solely on reported symptoms, a situation that poses challenges when evaluating children, particularly those in the preverbal age range. In their study of children trekking in Nepal, for example, Scrase et al. (2009) found that the children over- and under-reported symptoms on the Lake Louise Scoring System (LLSS) when compared to the objective physical measures or observations by parents and investigators. Similarly, Southard et al. (2007) reported that the language used in the LLSS tended to underestimate AMS symptoms in children ages 4–11. In younger and, in particular, preverbal children who cannot articulate how they feel, the diagnostic challenges are likely to be worse. In such children, AMS manifests as lack of playfulness, fussiness, anorexia, nausea, and altered sleep patterns (Yaron et al. 2002), but such changes are nonspecific and hard to distinguish from other potential causes. In the setting of a recent increase in altitude, the only safe course is to assume a young child’s fractiousness is due to AMS unless there are signs clearly pointing to some other cause. Yaron et al. (1998) have proposed a scoring system, referred to as the Children’s Lake Louise Score (Figure 26.2) for preverbal children incorporating a “fussiness score” and other questions the parents answer including “How well has your child eaten?” “How playful is your child today?” and “How has the child napped today?” To evaluate this scoring system, they studied 23 children aged 3–36 months who ascended over four days from 1610 m to 3488 m by car. Twenty-one percent of the infants were diagnosed as having AMS using this Children’s Lake Louise Score, compared to 20% of the adults who were evaluated using the standard LLSS. In a subsequent study, the proposed scoring system was shown to have good inter-observer agreement (Yaron et al. 2002). Figure 26.2The Children’s Lake Louise Score. Acute mountain sickness is present if there has been a recent gain in altitude and the Children’s Lake Louise Score is >7 with both FS ≥4 and (E + P + S) ≥3. (Adapted from Yaron and Niermeyer 2008.) Consensus guidelines recommend against pharmacologic prophylaxis for acute altitude illness and instead emphasize the importance of a slow ascent profile (Pollard et al. 2001). Medications can be used for prevention, however, in cases where overly rapid ascent is unavoidable or the child has a history of altitude illness or re-entry HAPE. Acetazolamide is the preferred agent for prevention of AMS, while nifedipine can be used to prevent HAPE. Although nifedipine is used in children and adolescents for other purposes, its safety and efficacy in this population have not been established in this group (Chu et al. 2014). Appropriate doses for children are listed in Table 26.1. Dexamethasone is generally reserved in children for treatment of severe altitude illness only and is not used for prevention. Tadalafil and sildenafil should not be used in children for HAPE prophylaxis or treatment due to the lack of safety and efficacy data in this age group. Drug Dose Route Acetaminophen 10–15 mg kg every 6 h; max 75 mg kg−1 day−1 for infants and lesser of 100 mg kg−1 day−1 or 1625 mg day−1 in children Oral Acetazolamide AMS prophylaxis: 1.25 mg kg−1 per dose every 12 h; max 125 mg per dose Oral AMS treatment: 2.5 mg kg−1 per dose every 8 h to 12 h; max 250 mg per dose Oral Dexamethasone* 0.15 mg kg−1 per dose every 6 h Oral, IM, or intravenous Nifedipine*** 0.5 mg kg−1 per dose every 8 h; max 20 mg for capsules, 40 mg for tablets; slow release preparation preferred** Oral Source: Pollard et al. (2001). Abbreviation: AMS, acute mountain sickness; IM, intramuscular. * Dexamethasone should be reserved for treatment of severe altitude illness only. ** Sustained release preparations cannot be broken to obtain smaller doses. In those cases, regular (i.e., non-sustained release) preparations should be used). *** Safety and efficacy not established in children. Mild AMS can be managed with rest and nonopiate analgesics while remaining at the same elevation, provided the child has a reliable caregiver and options for descent or more advanced care are available if the child’s condition worsens. Aspirin should be avoided because of the slight risk of Reye’s syndrome. Further ascent should be avoided unless all symptoms have resolved. There have been no formal trials of any drugs in children in this setting, but the same medication can be used in children as in adults at the appropriate pediatric doses (Table 26.1) (Pollard et al. 2001). Symptoms and signs of HAPE or HACE mandate immediate descent or transfer to a health facility with supplemental oxygen. Management of these disorders is similar to that in adults. Portable hyperbaric chambers have been used in children when descent is not feasible (Taber 1994) although care must be taken to assess for and prevent the inner ear problems that can be seen with these devices. Given reported associations between HAPE and underlying cardiopulmonary abnormalities, such as pulmonary hypertension (Durmowicz 2001) or unilateral absence of a pulmonary artery (Hackett et al. 1980; Rios et al. 1985), children who develop exaggerated hypoxemia or other evidence of HAPE at high altitude should undergo diagnostic evaluation by their pediatrician upon return to sea level (Yaron and Niermeyer 2008). Given concern that hypoxemia contributes to the pathophysiology of SIDS, questions have been raised about whether the risk of SIDS is increased at high altitude. Two older case-controlled studies suggested the risk of SIDS was, in fact, increased at high altitude (Getts and Hill 1982; Kohlendorfer et al. 1998), but these studies were performed in an era before current programs emphasizing avoidance of the prone position during sleep and, as a result, the observed differences may have reflected the effects of local child-rearing practices rather than the altitude, per se. More recently, Katz et al. (2015) have shown that the altitude at which a child resides is associated with increased incidence of SIDS, particularly among those living above 2400 m in elevation, and that the risk persists even in an era with a strong public health emphasis on avoiding sleep in the prone position. It is important to note, however, that these studies have focused on children living at high altitude on a long-term basis. There is currently no evidence regarding risk associated with short-term exposure, as would be the case on a family vacation, for example, and, as a result, no basis for changing a family’s approach to sleep for their young infant. Sleep disturbances at high altitude are well documented in adults (see Chapter 17), but only a few studies have examined these issues in children. Yaron et al. (2004) monitored motion in infants and young children (range, 4–33 months) with ankle actigraphy during sleep at home at just above 3100 m and found significant disturbances in sleep that were most pronounced on the first night at altitude. More recently, Gavlak et al. (2013) performed sleep studies on children aged 6–13 years in London (130 m) and at 1300 m and 3500 m during a five-day trek in Nepal and found that the children spent increasing amounts of time in periodic breathing with increasing altitude and had a greater number of episodes of hypoxemia. While these studies indicate children experience changes in sleep with increasing elevation, the extent of the changes relative to adults has only been examined in a single study. Kohler et al. (2008) compared sleep responses in 20 child-father pairs during one night at 490 m and two nights at 3450 m and found that the children (ages 9–12 years) spent less time in periodic breathing on both nights at high altitude than the adults and had a lower apnea threshold, but had similar drops in their nocturnal oxygen saturation and end-tidal carbon dioxide tension. Total sleep duration was similar between the children and adults, although they were unable to assess the distribution of sleep stages. There is a limited literature on pharmacologic interventions to improve nocturnal periodic breathing and sleep at high altitude (Luks 2008), but no studies have examined this issue in children. As a result, it is likely prudent to avoid use of medications for this purpose in the pediatric population. Children face an increased risk of hypothermia when traveling at high altitude. Due to their large body surface area to weight ratio and lack of subcutaneous fat, they lose heat faster than adults. In addition, children being carried on their parents’ back generate less heat than those engaging in exercise, while very young infants have a less efficient shivering mechanism than adults, which can impair their ability to generate heat as their body temperature begins to fall (Klein 2017). Increased susceptibility to heat illness is often cited in reviews on travel with children in the wilderness with emphasis placed on the fact that they generate more heat per kilogram with exercise, have lower cardiac output for a given work rate, and have impaired sweat responses relative to adults (Klein 2017). However, a detailed review of thermoregulation in the heat in children (Rowland 2008) suggests that these concerns may be overstated and that children do not face a greater risk than adults from exercising in warm temperatures. Independent of any differences in physiologic responses to cold or heat, one key issue that does affect risk is the fact that preverbal children cannot communicate about symptoms related to heat or cold illness while older children lack the knowledge necessary to recognize the onset of these problems and take appropriate actions in response. Parents traveling at high altitude should carefully monitor their children and ensure they have proper clothing, such as windproof and water resistant outer garments in cold weather, and add and remove layers of clothing as needed as conditions change. Careful attention should be paid to ensure adequate fluid intake, which can be useful for preventing both cold and heat illness. Further details of preventing and treating cold and heat injury in children have been reviewed in detail elsewhere (Klein 2017). Because about 23% of lifetime ultraviolet light exposure occurs before the age of 18 (Godar et al. 2003) and the risk of developing melanocytic nevi, a major risk factor for malignant melanoma, is related to the number of sunburns that occur at a young age (Dulon et al. 2002), emphasis has been placed on limiting childhood sun exposure. This concern is heightened during travel to high altitude, as the amount of ultraviolet light increases significantly at higher elevations, particularly when traveling on snow-covered terrain where there is a large increase in the amount of reflected light. Care should be taken to ensure children wear clothing and hats that cover as much skin as possible given the ambient temperatures and to liberally apply sunscreen to any sun-exposed areas. Proper eye protection, including wrap-around glasses, should be used to decrease the risk of ultraviolet keratitis (discussed in Chapters 23 and 27). Due to the fact that they are living longer than in the past and have the money, time, and inclination to go sightseeing, skiing, trekking, and climbing in the mountains, increasing numbers of individuals over the age of 50 are traveling to high altitude. For example, whereas Hultgren (1992) reported in 1992 that 10% of trekkers in Nepal were 50 years of age or older, a much more recent study by Keyes et al. (2016b) from the Solukhumbu region of Nepal demonstrated that 47% of trekkers were over the age of 50, while another 15% were over the age of 60. Given these increasing numbers of older individuals traveling to high altitude, it is worth considering potential effects the aging process may have on an individual’s ability to travel and engage in physical exertion in this environment. As individuals age, the likelihood they have a known or as yet unrecognized medical problem increases. In addition to assessing and mitigating the risk of acute altitude illness faced by all high altitude travelers, consideration must be given to whether the underlying problem(s) will worsen at high altitude or predispose the individual to various complications during their travels. This concern is nicely demonstrated by the Tenth Mountain Division study (Levine et al. 1997) in which an individual with a history of prior myocardial infarction, coronary artery bypass graft surgery, and atrial flutter sustained a myocardial infarction after a cardiopulmonary exercise test done as part of the study at 2500 m in Colorado. Patients with underlying medical problems or who are of advanced age warrant pretravel evaluation to assess the risk of planned travel and devise strategies for managing these issues. A more complete discussion of the range of chronic medical problems that can affect individuals at high altitude is provided in Chapter 25. While some people who travel to high altitude may not engage in physical activity, there are substantial numbers of older individuals who participate in trekking, ski touring, climbing, and other activities involving exertion, including those at the extremes of elevation. Given the well-established effects of ascending to high altitude on maximum exercise capacity, physiologic responses to exercise, and perceptions of dyspnea with exertion—described in Chapter 18—it is worth considering the potential effects of the aging process on exercise performance in older individuals at high altitude. It is well established that all individuals experience a decline in maximum exercise capacity or From a theoretical standpoint, one factor that might contribute to greater decrements in older individuals is the age-related change in exercise capacity. This decline, which has been consistently demonstrated in cross-sectional (Heath et al. 1981; Inbar et al. 1994) and longitudinal studies (McGuire et al. 2001; Rogers et al. 1990), results from a variety of factors, including changes in peripheral oxygen extraction and decrements in maximal cardiac output, depending on the individual’s age (McGavock et al. 2009; McGuire et al. 2001). The rate of decline varies across studies from as low as 0.28 mL/kg/min/yr (Rogers et al. 1990) to as high as 1.04 mL/kg/min/yr (Dill et al. 1967), with the differences in reported rates likely resulting from variations in study design such as participant age, the time intervals between assessments, and the participants level of fitness at the time of enrollment (McGuire et al. 2001). The decline may accelerate at the extremes of old age (Loe et al. 2013), but whether it is affected by the individual’s level of regular physical activity is unclear as studies have reported conflicting results in this regard (Rogers et al. 1990; Wilson and Tanaka 2000). Due to the lack of direct comparisons between exercise responses in younger and older individuals at high altitude, as noted previously, it is difficult to determine whether these age-related changes augment the effect of altitude on exercise capacity and, if so, whether that interaction is additive or synergistic. Most individuals who engage in exercise at high altitude do not exert themselves at maximal exercise capacity. Unfortunately, information is also lacking with regard to the ability of older individuals to perform and sustain submaximal levels of exercise at high altitude. Theoretically, given the effects of age noted above, an older individual exerting themselves at the same work rate as a younger individual at high altitude would be operating at a higher percentage of their maximum exercise capacity, which might, in turn, make it difficult to sustain that level of activity. In the end, whether the aging process has an effect on exercise capacity at high altitude, there is no basis for precluding exercise in older individuals in this environment. The fact that 92 people 60 years or older, including 10 people over the age of 70 have successfully climbed Mount Everest (8848 m) between 1953 and 2018 (2004), as well as the fact that many hundreds of individuals over the age of 60 have attempted to climb peaks >7000 m (Figure 26.3) clearly demonstrate that substantial physical activity, even at extremely high altitude, is feasible for some. The key factors that will most likely limit exercise capacity will be the baseline fitness level of the individual as well as their underlying health status. Sedentary individuals and/or those with underlying cardiopulmonary disease, such as coronary artery disease, cardiomyopathy, or chronic obstructive pulmonary disease will experience greater limitation than those who are fit and lack cardiopulmonary disease. Sedentary individuals should engage in a training program prior to planned high altitude travel with physical activity, provided they are deemed safe to do so by their medical providers. Figure 26.3Number of climbers ≥60 years of age who have attempted to climb mountains >7000 m in elevation between 1953 and 2017. Attempt is defined as traveling above base camp. Figure created with data from the Himalayan Database (Hawley and Salisbury 2004). Accessed March 2018. As described in Chapter 6, exposure to acute hypoxia blocks degradation of HIF-1α, leading eventually to a broad range of downstream cellular and molecular level responses with subsequent effects on energy utilization, angiogenesis, erythropoiesis, nitric oxide metabolism, and chemoreceptor function. The extent to which aging affects these and other physiologic responses that occur as part of acclimatization to high altitude has only been examined to a limited extent. This is one aspect of acclimatization that has been studied extensively. Lhuissier et al. (2012), for example, analyzed data from a cross-sectional study of 4675 subjects examined over a 20-year period and noted that the HVR increased with age in men both at rest and with exercise. The observed changes, which were not seen in women, were greater in trained rather than untrained individuals (Figure 26.4). Similar changes were seen in a longitudinal study of 30 subjects who underwent repeat assessment at a mean interval of 10.4 years (range 5–19 years). This finding of an increased HVR is similar to that reported by Chapman and Cherniack (1987), but stands in contrast to other studies that have reported either no change (Pokorski et al. 2004; Vovk et al. 2004) or a decrease (Garcia-Rio et al. 2007; Serebrovskaya et al. 2000) in HVR with aging. The reason for the differences in results is not clear, but likely relates to methodological differences between the various studies, including the technique for assessing HVR, whether the assessments were done at rest or with exercise and whether the measurements were done under isocapnic or poikilocapnic conditions. Figure 26.4Changes in the ventilatory response to hypoxia (HVR) at rest with aging in untrained (N = 1411) and trained (N = 475) women and untrained (N = 1709) and trained (N = 1080) men. P values refer to the effect of age; ns, nonsignificant. (Source: Lhuissier et al. 2012.) Another aspect of acclimatization where one might expect changes with aging is the hematologic response to hypoxemia and, in particular, the increase in red cell mass and hemoglobin concentration that occurs over a period of weeks following ascent and helps maintain tissue oxygen delivery (discussed in Chapter 13). It has been recognized, for example, that the prevalence of anemia increases with aging (Price 2008). While anemia in the elderly is often related to underlying medical problems, such as iron deficiency or renal insufficiency, up to 37% of older individuals with anemia fall in a category referred to as unexplained anemia of the elderly in which no obvious cause is apparent (Ferrucci et al. 2007). Given this finding, one might expect older individuals to have suppressed erythropoietic responses following ascent. However, no studies have compared changes in erythropoietin levels, red cell mass, or hemoglobin concentrations following ascent in younger and older individuals and, as a result, it remains unclear as to whether this aspect of acclimatization does, in fact, change with age. While theoretically not a form of acclimatization in the sense that it does not improve tolerance to hypoxia and may, in fact, predispose to problems such as diminished exercise capacity or HAPE, hypoxic pulmonary vasoconstriction (HPV) does appear to change with age. Turner et al. (2015), for example, exposed younger (<25 years of age) and older participants (>60 years of age) to the equivalent of 2500 m for two hours in a hypobaric chamber and estimated higher systolic pulmonary artery (PA) pressures in the older group. While this result was suggestive of increased HPV, the authors did not control for differences in alveolar oxygen tensions between the two groups that would affect the magnitude of HPV or assess cardiac output, another contributor to the measured pulmonary artery pressure. In a more recent study, however, Balanos et al. (2015) isolated the effects of HPV on changes in PA pressure by maintaining isocapnia and measuring cardiac output with echocardiography in 12 younger (mean age 20.5 ± 0.5 years) and nine older (mean age 55.8 ± 2.1 years) men exposed to 20 minutes of hypoxia (end-tidal PO2 50 mmHg). They found a greater increase in pulmonary artery pressures in the older subjects (15.2 ± 1.3 mmHg vs. 9.6 ± 0.9 mmHg), which, given the lack of differences in cardiac output between the two groups, could be attributed to enhanced HPV in the elderly (Figure 26.5). Figure 26.5Changes in estimated systolic pulmonary artery pressure during exposure to hypoxia (PETO2 = 100 mmHg) in younger (circles, N = 12) and older individuals (squares, N = 9 individuals). (Source: Balanos et al. 2015.) The clinical implications of this change in HPV with age are not clear. HPV does contribute to the reduction in maximum exercise capacity at high altitude (Ghofrani et al. 2006) and, when excessive, plays a key role in the pathogenesis of HAPE (Bartsch et al. 2005). However, no systematic studies have investigated whether the risk of HAPE is increased in the elderly, while, as noted above, the effects of age on exercise capacity at high altitude remain unclear. Coffman et al. (2019) have shown that extravascular lung-water accumulation, which may be related to changes in pulmonary artery pressure following ascent, was greater in older (58 ± 5 years) versus younger (32 ± 6 years) individuals during a climb of Kilimanjaro, but there were actually no statistically significant differences in systolic pulmonary artery pressure at any altitude between the two groups. This suggested some variable other than HPV may have been responsible for the observation. Because development of acute altitude illness is an indicator of poor acclimatization, rather than looking at how individual parameters, such as HVR, change with aging, perhaps the best method for assessing the effects of age on acclimatization is to determine whether older individuals are more susceptible to acute altitude illness than younger individuals. Anecdotal evidence has suggested that older mountaineers actually do better than the young, but this may be because they do not climb as fast as younger individuals and, therefore, have a lower tendency to move too high too quickly, which is a major risk factor for developing acute altitude illness. Another attractive hypothesis that would lead one to expect differences in the incidence of AMS with aging is the “tight fit hypothesis.” This theory, which remains unproven, states that subjects with a large brain in relation to their skull experience a greater rise in pressure for a given degree of cerebral edema compared to those with smaller brains relative to skull capacity and a similar degree of edema (Ross 1985). The elderly, who have greater age-related atrophy than younger individuals, would be expected to have more intracranial space and, as a result, greater tolerance of cerebral swelling and less AMS. This could provide a rare example of the advantage of growing old, but has yet to be proven in systematic studies. Among the many studies that have assessed the incidence of AMS and risk factors for its development at a variety of high altitude locations around the world, the majority of studies report either no difference (Horiuchi et al. 2016; Kayser 1991; Stokes et al. 2010; Ziaee et al. 2003) or decreased (Gonggalanzi et al. 2016; McDevitt et al. 2014; Salazar et al. 2012) incidence of AMS in older individuals compared to younger individuals. A few studies have reported higher risk of AMS with increasing age (MacInnis et al. 2013; Tang et al. 2014), but the average age of the subjects included in these studies was low and, as a result, the studies provide little insight into risk in individuals that would be classified as elderly. Given the preponderance of data on this issue, it would be reasonable to conclude that aging alone does not adversely affect acclimatization and increase the risk of acute altitude illness. It remains unclear, however, whether aging has beneficial effects on acclimatization. As a result, older individuals should still adhere to the standard recommendations regarding rate of ascent and other measures to prevent acute altitude illness. They should not use the fact that they are older to justify faster than recommended ascents. Importantly, as previously noted, many elderly individuals will have underlying medical conditions that predispose to problems at high altitude, and for these reasons, slower ascents and increased time for acclimatization before starting physical activity may be warranted in certain circumstances. While much of the early research into the effect of hypoxia on humans used fit young men as their subjects, more recent studies now routinely include or focus solely on women, thereby providing an opportunity to consider women’s responses to acute hypoxia and susceptibility to altitude illness. In general, women respond to acute altitude exposure the same way as men. They demonstrate similar physiologic responses to acute hypoxia, experience similar changes in exercise performance, and face the same risk of acute altitude illness (Hackett et al. 1976; Maggiorini et al. 1990) as men. In addition, the fact that many women have now ascended peaks >8000 m in elevation, including several who have ascended all 14 of such mountains, shows they are equally capable of physical performance at the extremes of elevation. There are, however, several issues unique to women traveling to high altitude that warrant additional consideration. These issues are discussed next. One obvious difference between men and women that theoretically might affect responses to high altitude is the fact that women experience menstrual cycles. Given the known effects of ovarian hormones on ventilation, it is reasonable to ask whether hormonal changes in the menstrual cycle affect ventilatory responses to hypoxia that play a role in acclimatization. Muza et al. (2001) investigated this question by studying ventilatory responses in 14 eumenorrheic women in the luteal phase of the menstrual cycle and eight different women in the follicular phase at sea level during a 12-day stay at 4300 m. Whereas at sea level, alveolar ventilation, as assessed by the end-tidal PCO2, was higher in the luteal than the follicular phase, there were no differences between the luteal and follicular phase subjects in either resting ventilation or the hypoxic and hypercapnic ventilatory responses during the stay at high altitude. They also noted that the observed levels of alveolar ventilation and time course of ventilatory acclimatization were no different than those reported in men in separate studies. These results are similar to those of Loeppky et al. (2001), who showed no significant differences in resting minute ventilation, as assessed by the alveolar PCO2, between the luteal and follicular phases in 40 women exposed to a barometric pressure of 426 mmHg (∼4880 m) for a period of 12 hours. Ventilatory responses in the Loeppky study were assessed at rest. Given that menstruating women have been shown to have higher hypoxic and hypercapnic respiratory drives and, thus, greater exercise ventilation during the luteal phase of the menstrual cycle at sea level (Schoene et al. 1981), it is also reasonable to assess whether exercise ventilation and performance vary at high altitude based on the menstrual cycle phase. Beidleman et al. (1999) tested this hypothesis by studying exercise responses during Another key aspect of acclimatization, erythropoiesis, also does not appear to be affected by the phase of the menstrual cycle. Using a similar design as the study by Muza et al. (2001) noted previously, Reeves et al. (2001) examined erythropoietic responses in women during the luteal (N = 5) and follicular phases (N = 11) of the menstrual cycle at sea level and after 11 days at 4300 m and found no differences by cycle phase in erythropoietin concentration, reticulocyte percentage, hemoglobin concentration, hematocrit, red cell volume, or serum ferritin concentration at either time point. The lack of differences at high altitude was contrary to their original hypothesis that erythropoiesis would be decreased in the luteal phase. The authors thought this might be related to the imbalance in numbers of subjects between the luteal and follicular phase groups as well as a large number of factors, including changing saturation, acid-base balance, and iron stores over time at altitude and diurnal variation in erythropoietin concentrations, which might have confounded the analysis of a small overall sample size. Another relevant question is whether the decline in ovarian hormone secretion with menopause has any effect on ventilatory or other responses to hypoxia. Pokorski and Marczak (2003) compared the hypoxic ventilatory response in old and young women and found no difference between the two groups. More recently, in their study of changes in ventilatory responses to hypoxia with aging described previously, Lhuissier et al. (2012) found that the age-related increase in the ventilatory response to hypoxia seen in men was blunted in untrained postmenopausal women. Non-statistically significant increases with aging were seen in trained women (Figure 26.4). The method for distinguishing between trained and untrained subjects, however, was not clearly delineated in the study. Whether the changes observed in this study have any effect on susceptibility to altitude illness following menopause is unclear as no studies have examined this question. However, given the lack of clear differences in AMS incidence between men and women and the lack of clear differences in AMS incidence with aging noted above, it would be reasonable to conclude that menopause likely has no effect on the risk of AMS, HACE, or HAPE. Postmenopausal women should adhere to the same recommendations for altitude illness prevention as other individuals. Survey data suggest up to 30% of women trekking at high altitude may be using hormonal contraception (Miller 1999). One issue to consider regarding use of these medications at high altitude is whether use of hormonal contraception alters the risk of acute altitude illness. On the one hand, it has been suggested that by suppressing ovulation and progesterone secretion, hormonal contraception may impair respiratory acclimatization and, as a result, increase the risk of altitude illness (Keyes 2015; Wright et al. 2004). On the other hand, the respiratory stimulant effect of exogenously administered progesterone may yield a protective effect (Keyes 2015; Skatrud et al. 1978). At present, there is no conclusive evidence for either position. Sandoval et al. (2001) have shown that the incidence of AMS was lower in women taking oral contraceptives compared to men following a nine-hour exposure to a simulated altitude of 4572 m, but the low number of study participants and short duration of exposure make it difficult to draw firm conclusions from this study. A second issue is whether use of hormonal contraception increases the risk of thromboembolic events at high altitude. It is well known that hormonal contraception is associated with increased risk of thrombosis at sea level, particularly when used in women >35 years of age in conjunction with cigarette smoking, although the risk does vary based on the particular contraceptive preparation. Numerous cases of venous thromboembolism have been documented at high altitude, including in women using oral contraceptives (Shlim and Papenfus 1995), but there have been no systematic studies assessing the risks associated with hormonal contraceptive use at high altitude. In a survey of 316 women trekking to Everest base camp (Miller 1999), a significant number of women reported irregularities of menstruation, particularly when pills were not taken regularly, but no episodes of venous thromboembolism or other complications were reported. The study design and relatively small numbers of patients, however, prevent firm conclusions regarding safety of use in this environment. Given the widespread use of these medications and lack of clear evidence of complications related to their use at high altitude, women already on such therapy can remain on it during high altitude travel, but should maintain adequate hydration and mobility and avoid smoking. Women planning long high altitude expeditions, particularly in more austere environments, who would like more control over their menses or to avoid it altogether can also consider hormonal contraception during their sojourn, but should start the medications several months ahead of time to get used to the side effects. Progestin-secreting intrauterine devices warrant strong consideration for this purpose as they are safe for use in nulliparous women, suppress menstruation, carry a lower risk of thromboembolism, and are more effective at pregnancy prevention (Keyes 2015). Although published guidelines recommend against certain types of high altitude activities during pregnancy (Opinion 2002), survey data indicate that large numbers of women travel to high altitude while pregnant and even engage in a wide range of physical activities including hiking or backpacking, trail running, climbing, mountain biking, and skiing (Keyes et al. 2016a). Of note, despite the published recommendations, women report low rates of counseling about the safety of such activities (Keyes et al. 2016a). Despite the large numbers of people participating in such activities while pregnant, only limited data are available to assess whether exposure to hypobaric hypoxia poses risks to the mother and the fetus. Artal et al. (1995), for example, performed submaximal and maximal exercise tests on seven sedentary women at 34 weeks, gestation at sea level, and after two to four days of acclimatization at 1830 m, found no difference in fetal heart rate responses, or maternal lactate or epinephrine concentrations between the study time points, while Baumann et al. (1985) studied 12 pregnant subjects during exercise after ascent to 2225 m and found no evidence of abnormal fetal heart rate responses. Survey studies of obstetrical providers and high altitude travelers suggest that short-term exposure does not increase the risk of pregnancy-induced hypertension, spontaneous abortion, placental abruption, or placenta previa, although preterm labor and bleeding complications are sometimes encountered (Keyes et al. 2016a; Niermeyer 1999). Recognizing the limited number of studies on this question and the methodological issues that affect survey studies, high altitude travel should still be regarded as safe for women with normal, low-risk pregnancies (Huch 1996; Jean and Moore 2012). Pretravel evaluation, including ultrasound, is warranted to ensure the pregnant individual lacks conditions that would qualify as contraindications to high altitude activities, particularly after the 20th week of pregnancy (Table 26.2) (Jean and Moore 2012), although women who live near and make frequent trips to high altitude (e.g., weekend ski trips) do not need such evaluation before every sojourn. Once at high altitude, pregnant women should maintain adequate hydration, avoid travel into remote areas, and not exceed levels of exertion done at home. Care should be taken to prevent acute altitude illness, as severe hypoxemia during HAPE could impair fetal oxygenation. Medications can be used for prophylaxis and treatment of altitude illness (Table 26.3), but slow ascent remains the primary prevention strategy and descent the primary treatment strategy. Anemia Chronic hypertension Impaired placental function Intrauterine growth retardation Maternal heart or lung disease Pre-eclampsia Pregnancy-induced hypertension Smoking
Introduction
Children
Physiologic responses at high altitude
Neonates and Infants
Older Children
Acute altitude illness
Incidence
Diagnostic Challenges
Prevention and Treatment
Other issues with high altitude travel
Sudden Infant Death Syndrome (SIDS)
Sleep Disturbances
Susceptibility to Cold Illness and Heat Illness
Sun Exposure
Advanced Age
Underlying medical conditions
Changes in exercise performance with age
Effect of age on acclimatization
Ventilatory Responses to Hypoxia
Hematologic Responses
Hypoxic Pulmonary Vasoconstriction
Susceptibility to acute altitude illness
Women
Effects of the menstrual cycle on acclimatization and exercise performance
Effects of menopause
Hormonal contraception
High altitude travel during pregnancy
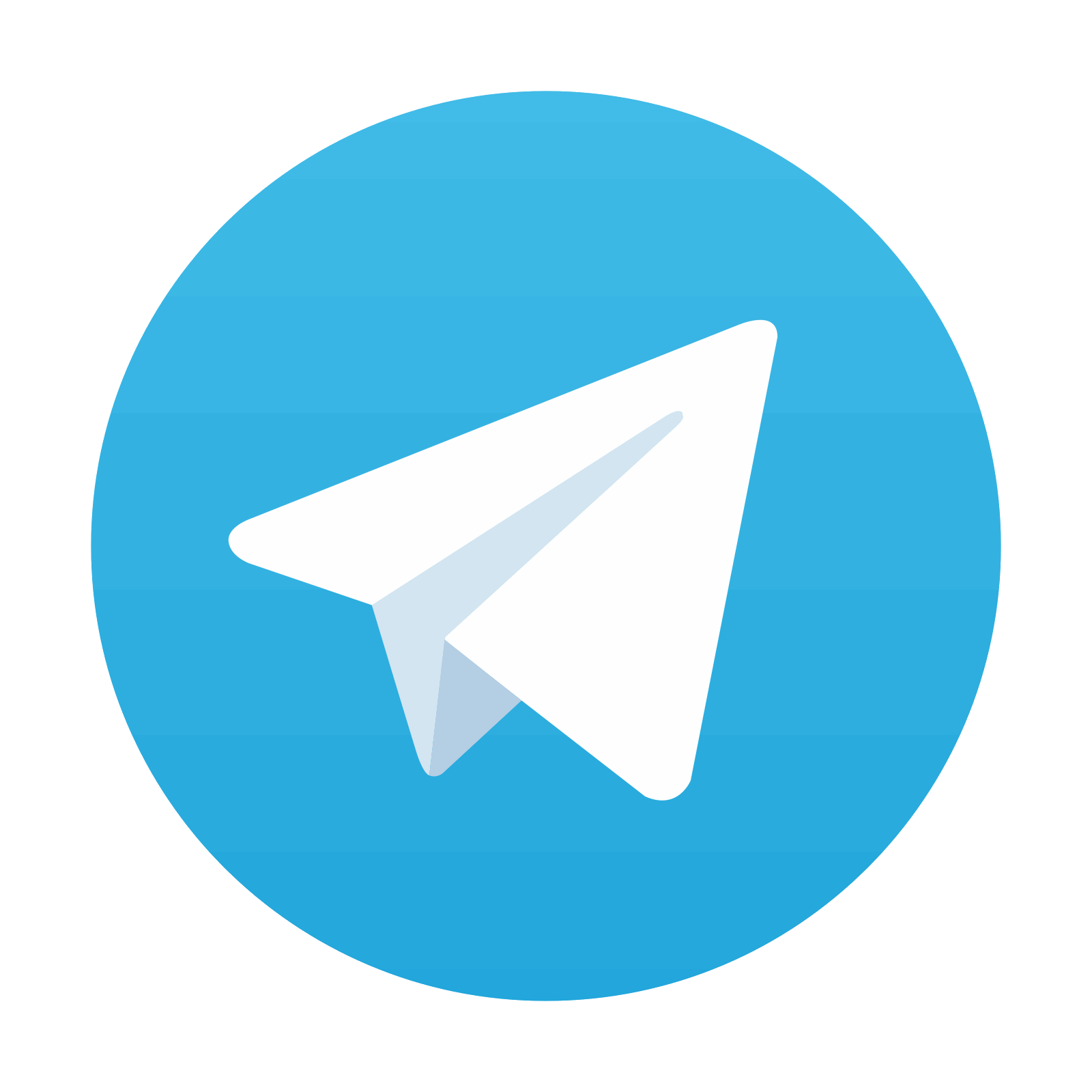
Stay updated, free articles. Join our Telegram channel

Full access? Get Clinical Tree
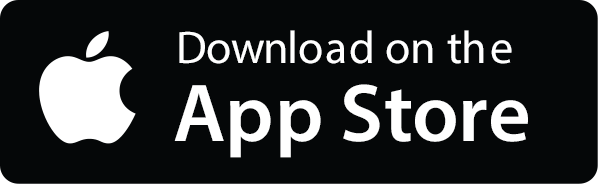
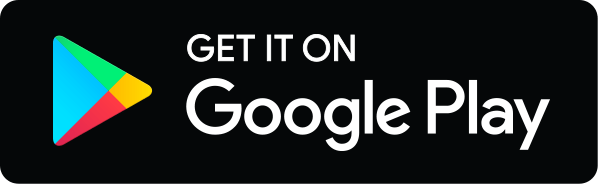