Abstract
While there are areas of overlap, childhood interstitial lung diseases (ILD) frequently include differing entities and histologic patterns than those described in the literature focusing on adult ILD. The areas of distinction are particularly apparent for ILD in infants and young children; therefore this chapter reviews specific forms of childhood ILD that are “more prevalent in infancy.”
Keywords
alveolar capillary dysplasia, interstitial glycogenosis, neuroendocrine cell hyperplasia of infancy
Overview
While there are areas of overlap, childhood interstitial lung diseases (ILD) frequently include differing entities and histologic patterns than those described in adult ILD. The areas of distinction are particularly apparent for ILD in infants and young children; therefore this chapter reviews specific forms of childhood ILD that are “more prevalent in infancy.”
One factor prompting the need for distinct consideration of childhood ILD in infants is that prominent case clustering occurs in infancy. In a series reported by Fan et al., the median age of onset was 8 months. Two subsequent large multicenter studies have also shown a predominance of cases in young children. In the European Respiratory Society task force survey of chronic ILD, children under age 2 years comprised 31% of cases. Because this study’s inclusion criteria required symptom duration of at least 3 months, neonates with a rapidly progressive course would have been excluded, and the proportion of cases in young children is likely underestimated. In studies utilizing lung biopsy ascertainment, the Children’s Interstitial Lung Disease Research Network (ChILDRN) has identified that approximately 50% of lung biopsies for diffuse lung disease are performed in children less than 2 years of age. Furthermore, within the cohort of young children, infants represent a large proportion of cases. Of 187 cases reviewed in the ChILDRN infant study, 30% underwent biopsy by 3 months of age and 52% underwent biopsy by 6 months of age.
As detailed in a 2013 American Thoracic Society Clinical Practice Guideline, it is useful to separately consider the clinical differential diagnosis of ILD in an infant versus an older child. Certain entities are either not seen in older children or clearly have symptom onset in infancy. Finally, there is high variability in morbidity and mortality associated with infant ILD disorders, emphasizing the critical need for accurate diagnosis. This chapter highlights several disorders that present in infancy or within the first year of life, including Alveolar Capillary Dysplasia with Misalignment of Pulmonary Veins (ACDMPV), Pulmonary Interstitial Glycogenosis (PIG), and Neuroendocrine Cell Hyperplasia of Infancy (NEHI). Additionally, while bronchopulmonary dysplasia is discussed in Chapter 20 , lung growth abnormalities are included in this chapter, because they manifest in infancy and their clinical and radiographic presentation frequently overlaps with other forms of infant ILD. Disorders of surfactant production and homeostasis, more prevalent in infancy but also present in older children, are discussed separately in Chapter 57 .
Alveolar Capillary Dysplasia With Misalignment of Pulmonary Veins
Epidemiology
Alveolar capillary dysplasia with ACDMPV is a rare and generally lethal developmental disorder of the lung that typically causes very early postnatal respiratory distress. The disorder is named based on distinctive abnormalities of the pulmonary vasculature and is also variably termed alveolar capillary dysplasia and congenital alveolar capillary dysplasia . As definitive diagnosis currently depends on histologic examination of the lung on biopsy or at autopsy, the true prevalence is unknown; over 200 cases of ACDMPV have been reported in the literature to date. While the majority of cases are sporadic, approximately 10% are familial with affected siblings, and no sex predilection has been identified.
Etiology and Pathogenesis
Heterozygous inactivating point mutations and microdeletions involving FOXF1 and its upstream interval on chromosome 16q24.1 have been found in a large number (~50%) of sporadic and familial cases of ACDMPV and concurrent anomalies. Among deletions in which parental origin can be determined, all arose de novo on the maternal chromosome consistent with FOXF1 being paternally imprinted. The ability to noninvasively test for this gene has recently uncovered that patients with FOXF1 mutations can have a variable phenotype, including milder symptoms with long-term survival. This variability may be because of somatic mosaicism, partial imprinting, or modifier genes. The frequently associated cardiac and gastrointestinal anomalies in patients with ACDMPV are thought to be due to haploinsufficiency for the neighboring FOXC2 and FOXL1 genes in the Forkhead box (FOX) transcription gene cluster.
FOXF1 in mice is regulated by hedgehog and bone morphogenetic protein (BMP) signaling and encodes a transcription factor expressed in the lung mesenchyme and involved in murine vasculogenesis and lung development. Mice with reduced levels of pulmonary Foxf1 die of pulmonary hemorrhage with deficient alveolarization and vasculogenesis, but they do not have malposition of pulmonary veins.
Mechanisms linking the abnormal lung vasculature with severe hypoxemia and pulmonary hypertension in ACDMPV remain to be fully explained. It is currently unclear whether the prominent arterial and lobular abnormalities in this disorder are primary or secondary to the vein misalignment. Medial hypertrophy of small pulmonary arteries may result from deficient lobular development with poor gas exchange and resultant hypoxemia, and intrapulmonary right-to-left shunts through bronchial veins have been demonstrated by three-dimensional image reconstruction of lung from infants who died from ACDMPV.
Clinical Features and Presentation
Infants with ACDMPV are usually born at term, and, in the majority of cases, the onset of cyanosis and respiratory failure occurs within 48 hours after birth. Delayed onset by weeks or even months has been described rarely, but this is becoming more recognized as genetic testing for FOXF1 mutations and deletions are now available. The initial presentation is indistinguishable from persistent pulmonary hypertension of the newborn, and echocardiogram typically demonstrates suprasystemic right ventricular pressures and right-to-left shunting through a patent ductus arteriosus or patent foramen ovale. Approximately 80% infants with ACDMPV have additional malformations with cardiac (commonly hypoplastic left heart), gastrointestinal (intestinal malrotation and atresias), and renal abnormalities (hydronephrosis) being the most frequent.
Radiologic Findings
The initial chest radiograph is often normal with subsequent development of diffuse hazy opacities, and the findings are also dependent on concurrent anomalies. Pneumothoraces are frequently reported, which may be a consequence of abnormal lung architecture or aggressive ventilation. Computed tomography (CT) or magnetic resonance lung imaging of patients with lethal ACDMPV have not been described, although a long-term survivor (36 months) had bilateral widespread ground glass opacities with relatively less affected areas on chest CT.
Histologic Findings
ACDMPV is defined by a characteristic constellation of features, of which anomalously situated pulmonary veins running alongside small pulmonary arteries is essential ( Fig. 56.1A–D ). Normally, pulmonary veins are in the interlobular septa, arising from small veins that drain pulmonary lobules. In addition to aberrant placement of the veins in ACDMPV, there is reduction or absence of veins in interlobular septa. Muscularization of small pulmonary arteries and arterioles is often striking, and there is reduced capillary density in alveolar walls (see Fig. 56.1E and F ). Simplification of the lobular architecture with lymphangiectasia is variably present.

Diagnosis and Differential Diagnosis
The diagnosis of ACDMPV should be considered in infants who present with severe hypoxemia and pulmonary hypertension for which no anatomical cause can be established. Historically lung biopsy or autopsy was required to confirm the diagnosis. Currently, genetic testing for disruption of FOXF1 may obviate the need for a tissue diagnosis. On histologic examination, the characteristic constellation of findings may be subtle, particularly when the lung sample is limited with few bronchovascular bundles for evaluation. Patchy distribution has rarely been reported, and multiple lung sections may be required for confirmation.
The presentation of ACDMPV with persistent pulmonary hypertension of the newborn overlaps with other etiologies of severe idiopathic neonatal lung disease, including genetic abnormalities in surfactant metabolism (particularly SFTPB and ABCA3 mutations ), diffuse PIG, and lymphangiectasia. Histologically, ACDMPV should be distinguished from other disorders that demonstrate a similar arrest in lung development, including congenital alveolar dysplasia and advanced pulmonary hypoplasia. While these disorders also demonstrate lobular simplification and frequently vascular changes (hypertensive changes of the pulmonary arteries and reduced capillary density within alveolar walls), they do not have malpositioned veins in the lobules.
Management and Treatment
Standard therapy is supportive and includes mechanical ventilation, inhaled nitric oxide, and extracorporeal membrane oxygenation (ECMO). These therapies prolong life by days to weeks but have rarely led to long-term survival, and lung transplantation has rarely been attempted.
Prognosis
Historically initial presentation of ACDMPV in the neonatal period denoted a grim prognosis. However, variable phenotypes and longer survival are now increasingly reported. The clinical presentation should be considered in determining on a case-by-case basis.
Lung Growth Abnormalities Presenting as Childhood Interstitial Lung Diseases
Epidemiology
The epidemiology of lung growth abnormalities is best considered in the context of their associated conditions including prematurity, oligohydramnios, congenital heart disease, and Trisomy 21. While only a small portion of cases undergo lung biopsy, it is important to acknowledge that lung growth abnormalities are a principal cause of diffuse lung disease in young children that prompts surgical lung biopsy. In the ChILDRN study, lung growth abnormalities represented the primary diagnostic category, with 25% of lung biopsies from children less than age 2 being classified in this category. Furthermore, this was the leading diagnostic category for five of the eleven participating centers.
Etiology and Pathogenesis
Lung growth is a continual process that occurs well into the postnatal period. As such both prenatal and postnatal insults can impact lung maturation and growth. Distension of the lung with liquid and fetal respiratory movements is required for normal fetal lung growth, so any mechanism that interferes with these processes can result in a prenatal growth disorder. Prenatal onset pulmonary hypoplasia ranges from mild to severe, depending on the mechanism of hypoplasia and timing of the insult in relation to the stage of lung development. Early insults that occur before 16 weeks gestation (renal anomalies, congenital diaphragmatic hernia) may interfere with airway branching as well as acinar development, while later events (premature rupture of membranes) will exclusively impact acinar development. Postnatal onset growth abnormalities impact late alveolarization, most evident in the subpleural space. Multiple factors likely lead to deficient alveolarization in infants with congenital heart disease, including hypoxia and abnormal pulmonary blood flow.
In addition to chromosomal disorders, single gene disorders are also associated with abnormal lung growth, particularly deficient alveolarization. For example, while mutations or deletions in NKX2.1 may present with a phenotype of surfactant dysfunction, alveolar simplification may be the predominant finding. Mutations in FLNA (filamin A), which cause X-linked periventricular nodular heterotopia, have also been associated with severe diffuse lung disease with alveolar simplification, lung cysts, and pulmonary hypertension.
Clinical Features and Presentation
While not traditionally considered a classic form of ILD, abnormalities of lung growth represent a prominent proportion of cases that undergo lung biopsy to define the nature of the diffuse lung disease. Furthermore, the features of tachypnea, retractions, hypoxemia, and often diffuse radiologic abnormalities overlap with other forms of childhood ILD.
Although lung growth abnormalities are traditionally considered in the context of prematurity and prenatal onset pulmonary hypoplasia, they also occur in the setting of congenital heart disease, chromosomal abnormalities (particularly Trisomy 21), and in otherwise normal-term infants with early postnatal lung injury ( Table 56.1 ). While difficult to objectively define, most infants diagnosed with lung growth abnormalities by lung biopsy were reported to have clinical severity deemed out of proportion to their known comorbidities or circumstances. In other words, typically an additional form of ILD is suspected, leading to the decision to pursue surgical lung biopsy in these patients.
Factors limiting prenatal lung growth |
|
|
|
Prematurity-related chronic lung disease (classically termed bronchopulmonary dysplasia ) |
Congenital heart disease |
|
|
Genetic defects |
|
|
|
|
Radiologic Findings
Radiologic findings are variable based on the etiology, age of the infant, and severity of the growth abnormality ( Fig. 56.2 ). Subpleural cysts may be present and are frequently seen in pulmonary hypoplasia associated with Down’s syndrome.

Histologic Findings
The ratio of lung weight to body weight is the most reliable parameter of lung growth, especially in preterm infants. Obviously, this criterion is not useful for lung biopsies; therefore microscopic criteria are employed. The simplest method is the radial alveolar count, which is the number of alveoli transected by a perpendicular line drawn from the center of a respiratory bronchiole to the nearest septal division or pleural margin. The radial alveolar count in a full-term infant should average five alveolar spaces. While a valuable tool to evaluate lung complexity and growth, this method requires standardized inflation of the lung, as the radial alveolar count is substantially reduced in uninflated lungs. In prenatal onset pulmonary hypoplasia, there is a reduction of number of alveolar spaces for gestational age, which is often accompanied by prominence of the bronchovascular structures and a widened interstitium. Lobular simplification with alveolar enlargement, often accentuated in the subpleural space, characterizes deficient alveolarization of postnatal onset ( Fig. 56.3A and B ). Following the widespread use of surfactant replacement and other therapies, the histology of “new bronchopulmonary dysplasia (BPD)”/chronic neonatal lung disease is characterized by less fibrosis and more uniform inflation than the classic form of BPD. Hypertensive changes of the pulmonary arteries are commonly seen and are especially prominent in infants with a severe lung growth abnormality or Trisomy 21 (see Fig. 56.3C ). As discussed below, PIG is a frequent histologic finding in biopsies demonstrating impaired lung growth.

Diagnosis and Differential Diagnosis
Clinical context provides high pretest probability of this diagnosis, and consideration of the clinical setting may obviate the need for lung biopsy in many cases. Infant pulmonary function tests may suggest pulmonary hypoplasia, but they do not exclude concurrent processes. For infants with respiratory morbidity out of proportion to their clinical context, lung biopsy may still be required for diagnosis and to exclude alternative diagnoses. Proper tissue handling is essential, and expert pathologic review may be required.
The clinical differential diagnosis is broad and may include other abnormalities in lung development such as lymphangiectasia, ACDMPV, PIG, and genetic disorders of surfactant production and metabolism. From a pathology perspective, the presence of alveolar enlargement and simplification is often misinterpreted as emphysematous change or a consequence of lung remodeling after injury. However, in lung growth abnormalities, there is no evidence of a destructive process in a lung growth abnormality. Without proper orientation and handling, alveolar simplification may be overlooked or difficult to recognize. Indeed, in the ChILDRN study, the majority of lung growth abnormality cases were not suspected clinically or recognized histologically at the initial institution, especially when occurring outside the setting of prematurity.
Management and Treatment
Management is focused largely on supportive care and underlying conditions. Recognition of this category of diagnosis may have important clinical implications, particularly as corticosteroids may not be indicated in this setting.
Prognosis
Lung growth and maturation are key determinants of postnatal outcome, especially in premature infants or those with chromosomal or congenital abnormalities. Lung growth abnormalities are associated with considerable morbidity and mortality when compared to other causes of diffuse lung disease. In the ChILDRN study, mortality was 34% for lung growth abnormality cases, a proportion similar to the entire study cohort. However, among lung growth abnormality cases, prematurity and pulmonary hypertension were independent clinical predictors of mortality. On lung biopsy, severe lung growth abnormality, as judged by degree of alveolar enlargement and simplification, was associated with a high mortality (80%).
Pulmonary Interstitial Glycogenosis
Epidemiology
PIG is a form of childhood ILD unique to neonates and young infants. Although the incidence and prevalence of PIG are unknown and it is rare, PIG occurs more frequently and in a broader clinical spectrum than previously recognized.
Etiology and Pathogenesis
The etiology of PIG is incompletely understood, although clearly related to lung development. Expert pathologic review of lung biopsies from the multiinstitutional ChILDRN study found that patchy PIG was present in over 40% of cases classified as having a lung growth abnormality. This included not only chronic neonatal lung disease due to prematurity but also pulmonary hypoplasia and lung growth abnormalities in the setting of Trisomy 21 and congenital heart disease. Patchy PIG was also present in 22% of cases classified as vascular disorders masquerading as ILD. Published and personal experience indicates that it is very unusual to observe PIG in lung biopsies beyond 6 months of age.
In their original report, Canakis and colleagues speculated that PIG reflects a delay or aberration in the maturation of pulmonary mesenchymal cells that do not normally contain abundant glycogen. Others have suggested that PIG might reflect a nonspecific feature of several conditions that transiently alter lung growth and development in the neonatal period. Recently, the presence of interstitial lipofibroblasts, cells reportedly seen only during prenatal lung development, were demonstrated in PIG biopsies. Pulmonary lipofibroblasts are known to play critical roles in lung maturation through stimulating alveolarization during the period of septation and in lipid homeostasis by mediating lipid transfer to type 2 alveolar epithelial cells for surfactant phospholipid synthesis. Furthermore, these mesenchymal cells have been demonstrated to have transient proliferative capacity, which is similar to interstitial fibroblasts in animal models of lung development and injury. Within these models, coordinated control of fibroblast proliferation and apoptosis is necessary for septation of the distal airspaces during alveolarization. Collectively, this evidence including the presence of lipofibroblasts in PIG strongly suggests that aberrant lung development is a critical component of PIG.
Clinical Features and Presentation
Most infants with PIG are symptomatic in the first days to weeks of life, which may follow an initial period of well-being after birth. The clinical presentation can be variable, ranging from indolent tachypnea and hypoxia to neonatal acute respiratory failure and pulmonary hypertension. The initial report of PIG included seven neonates, and five of whom presented in the first 24 hours of life. In the ChILDRN study, six cases (3.2% of infant lung biopsies) were identified in which PIG was the only significant histologic finding. All but one case presented with hypoxemia at birth, and the mean age at biopsy was 1.3 ± 0.4 months. There is clinical and pathologic evidence to support that PIG is often a self-limited disorder, and the diagnosis is typically made by 6 months of age.
PIG can occur in either term or premature infants and may be an isolated disorder or a component of other congenital conditions. PIG commonly occurs in the setting of lung growth abnormalities (see earlier) and has also been observed in infants with congenital heart disease, Trisomy 21, pulmonary hypertension, meconium aspiration, and rarely other developmental anomalies.
Radiologic Findings
Case series have reported that chest radiographs have diffuse infiltrates or hazy opacities, but no common high-resolution CT (HRCT) scan pattern has been identified ( Fig. 56.4A–D ).

Histologic Findings
Formally known as cellular interstitial pneumonitis and histiocytoid pneumonia, the term PIG was coined by Canakis et al. based on the histologic finding of increased glycogen-laden mesenchymal cells in the alveolar interstitium. Lung biopsy shows a patchy or diffuse expansion of the alveolar walls by bland spindle-shaped cells with pale or bubbly cytoplasm (see Fig. 56.4E and F ). Patchy distribution is frequent when there is a concomitant lung growth abnormality, and associated inflammation or fibrosis in the interstitium is absent. The cells are strongly immunopositive for vimentin, a mesenchymal marker, and periodic acid–Schiff (PAS) stain may demonstrate PAS positive diastase labile material within the cytoplasm of the cells (see Fig. 56.4G and H ). As the preservation of glycogen is influenced by using aqueous fixatives (i.e., 10% formalin), the presence of PAS positivity may be difficult to demonstrate on routine sections. Electron microscopy is considered the best approach to reveal the accumulation of monoparticulate glycogen in these interstitial cells, and treating ultrathin sections with tannic acid enhances visualization of glycogen.
Diagnosis and Differential Diagnosis
Currently, lung biopsy is the only way to diagnose PIG. The diagnosis may be suspected in the context of a neonate with respiratory compromise, particularly when the severity is disproportionate to the degree of coexisting conditions, including prematurity and congenital heart disease.
Disorders to be considered in the clinical differential diagnosis include sepsis, congenital heart disease, lung developmental disorders (e.g., alveolar capillary dysplasia with misalignment of the pulmonary veins), pulmonary hypoplasia, pulmonary vascular disease, lymphangiectasia, and genetic disorders of surfactant production and metabolism. Primary ciliary dyskinesia (PCD) could also mimic milder cases of PIG due to the frequent occurrence of neonatal tachypnea in PCD. From the perspective of the pathologist, careful attention is required to identify potential concurrent histologic findings, such as lung growth abnormalities or structural alterations of the pulmonary vasculature.
Management and Treatment
Most children will require supplemental oxygen and some will require aggressive support including mechanical ventilation and therapy for pulmonary hypertension. When present, comorbidities such as congenital heart disease or complications of prematurity are often the focus of management.
High-dose intravenous corticosteroids have been reported to have benefit in case reports and case series, but no controlled studies have been performed and there is little evidence to guide therapeutic recommendations. It has been suggested that consideration for use of corticosteroids should be assessed in the context of clinical severity and the potential detrimental impact on postnatal alveolarization and neurodevelopment in this patient population.
Prognosis
The natural history is unknown, but mortality is largely related to complications of prematurity or pulmonary hypoplasia. Patients may remain symptomatic for months, and supportive care is a mainstay of therapy. In the original report by Canakis et al., six of the seven infants improved over time, with mortality occurring in one infant born at 25 weeks gestation. Similarly, in the ChILDRN study, no mortality occurred among the six cases of diffuse PIG, and clinical improvement has also been reported in the small number of published case reports. However, high mortality has occurred when significant pulmonary conditions are present along with PIG, particularly lung growth abnormalities.
Neuroendocrine Cell Hyperplasia of Infancy
Epidemiology
NEHI is a rare disorder previously termed “Persistent Tachypnea of Infancy.” The incidence and prevalence of NEHI are unknown, and it is suspected that many cases remain undiagnosed, although cases are increasingly recognized worldwide. In the ChILDRN study, NEHI cases represented 10% of all lung biopsies from children less than 2 years of age. A recent study from a large referral center identified 19 cases (14%) from among 138 lung biopsy cases accrued over a 10-year period. The original series of 15 cases had a slight male predominance, which was not observed in a subsequent study.
Etiology and Pathogenesis
The etiology of NEHI is unknown, but genetic mechanisms play a role in at least some cases. In one family, a heterozygous mutation in NKX2.1 (also called thyroid transcription factor 1, or TTF1) was identified in association with familial NEHI. The mutation results in a nonconservative amino acid substitution in the homeodomain in a codon extensively conserved through evolution. To date, NKX2.1 mutations have not been identified in other NEHI cases.
Lung biopsies in NEHI are characterized by prominent pulmonary neuroendocrine cells (PNECs) in the distal airways, but the role of PNECs in NEHI pathogenesis remains uncertain. A study correlating PNEC prominence and the severity of small airway obstruction on infant PFTs in NEHI patients suggests a potential causal role. Based on the role of PNECs and bombesin in oxygen sensing as well as airway and arterial tone, it has been hypothesized that neuroendocrine cells may lead to ventilation/perfusion mismatch within the lung. The physiologic abnormalities observed on infant pulmonary function tests in NEHI suggest that the disorder is not fully explained on this basis. The absence of active neuroendocrine cell proliferation in lung biopsies and lack of correlation with airway injury suggest that PNEC prominence in NEHI is not due to injurious stimuli.
Clinical Features and Presentation
NEHI occurs in otherwise healthy-term or near-term infants who present with tachypnea and retractions, generally of insidious onset, in the first few months to year of life. Rare cases in preterm infants have been documented. Hypoxemia is common, while chronic cough and wheezing are rare. Many patients come to medical attention because of failure to thrive or exacerbation of chronic symptoms with upper respiratory infections. However, respiratory symptoms are often mistakenly attributed to more common disorders such as bronchiolitis, gastroesophageal reflux, or aspiration. On physical exam, crackles are prominent but variably present. Chest wall deformity with increased anteroposterior diameter is common, while clubbing has not been reported.
Infant pulmonary function testing (PFT) in NEHI patients demonstrates a mixed physiologic pattern including profound air-trapping and proportionate reductions in the forced expiratory volume in 0.5 seconds (FEV0.5) and forced vital capacity (FVC), with particularly reduced FEF75 and FEF85 and markedly elevated functional residual capacity (FRC), residual volume (RV), and RV/TLC. Pulmonary function testing has not been systematically studied in older children, although limited published data and personal experience suggest that this physiologic pattern can persist in at least some cases.
Radiologic Findings
Chest radiographs may be normal or may reveal hyperinflation. HRCT findings are distinctive with geographic ground-glass opacities centrally and in the right middle lobe and lingula ( Fig. 56.5A–C ). Air-trapping is often demonstrated when expiratory images are performed. Brody et al. evaluated chest HRCT scans from 23 children with biopsy-proven NEHI and 6 children with other forms of ILD. While HRCT specificity for the diagnosis of NEHI was 100% in this study when the CTs were reviewed by two expert radiologists, the sensitivity of HRCT was incomplete, as readers did not suggest NEHI in up to 22% of the cases.

Histologic Findings
In contrast to the clinical severity of the disorder, lung biopsies in NEHI often show minimal to no pathologic alterations and may be initially interpreted as normal (see Fig. 56.5D ). Patchy mild inflammation or fibrosis (bronchiolitis) may be present, especially following a viral infection, but this not sufficient to support an etiology for the patient’s significant symptomatology. Pathologic diagnosis of NEHI rests on finding an increased proportion of neuroendocrine cells within distal airways, best seen by bombesin and serotonin immunohistochemistry. Neuron-specific enolase, calcitonin, synaptophysin, and chromogranin have been shown to be less reliable in demonstrating this increase in neuroendocrine cell numbers. Immunohistochemical assessment of this disorder requires an adequate biopsy with at least 10–15 airways for evaluation. As wide intra and intersubject variability in neuroendocrine cell number has been reported, which does not relate to imaging appearance of the region biopsied, more than one biopsy site is recommended. Morphometric quantification of bombesin staining may be required. In general, bombesin immunopositive cells in NEHI are prominent in the distal respiratory bronchioles and as clusters (neuroepithelial bodies) in the alveolar ducts (see Fig. 56.5E and F ). Two individual airways with more than 10% bombesin-immunopositive area or cell number of the airway epithelium is also suggestive of the diagnosis, although may not be present. A minor degree of airway injury does not alter pathologic confirmation of the diagnosis.
Diagnosis and Differential Diagnosis
Clinical suspicion is essential in the diagnosis of NEHI. The initial diagnostic approach should be to exclude more common causes of the clinical symptoms such as infection, cystic fibrosis, and congenital heart disease. In the appropriate clinical context, the diagnosis may be made by chest HRCT in a majority of cases and sometimes be supported by data from infant PFTs, which may play a role in supporting the diagnosis of NEHI. At this time, genetic testing is not routinely helpful in making the diagnosis.
Although lung biopsy is the historical gold standard, lung biopsy is not required to make the diagnosis except in patients with atypical clinical or HRCT findings. There are important limitations to lung biopsy. Specifically, the numbers of PNECs are variable and not always seen without sufficient airway sampling. Furthermore, the presence of increased number of neuroendocrine cells on lung biopsy is not sufficient for the diagnosis, as PNEC prominence is associated with a variety of other pulmonary conditions, including BPD, sudden infant death syndrome, pulmonary hypertension, cystic fibrosis, and follicular bronchiolitis.
Disorders to be considered in the clinical differential include acute or chronic infection, asthma, and airway injury including bronchiolitis obliterans. In addition, other infant lung disorders such as pulmonary hypoplasia, PIG and genetic disorders of surfactant production, and metabolism should be considered. As discussed previously, neuroendocrine cell prominence on lung biopsy should be correlated with other potential pathologic findings, and other entities associated with increased neuroendocrine cells excluded.
Management and Treatment
There is no known definitive therapy for NEHI, and management largely consists of general supportive and preventative care. Most children will require supplemental oxygen, and many will require nutritional supplementation. Corticosteroids are not helpful in most cases ; therefore establishment of the diagnosis of NEHI helps to avoid the complications of long-term steroid therapy. Genetic counseling and family support are important components of care for all families who experience the diagnosis of a rare disease.
Prognosis
While long-term outcomes are not well established for this recently recognized disorder, a diagnosis of NEHI brings a cautious but welcomed prognosis relative to other forms of childhood ILD. There have been no deaths reported, and no patients have required lung transplantation. The clinical course is prolonged, but most children demonstrate gradual improvement. The need for supplemental oxygen is variable and may be many years in duration. Patients may also remain symptomatic with respiratory infections or exercise intolerance. In one report, six of nine infants developed a phenotype consistent with nonatopic asthma. Exacerbations, with concomitant air-trapping, have also been described. The CT abnormalities may persist into adulthood in at least some cases, but the time course of radiologic resolution in relationship to clinical parameters has not been systematically studied.
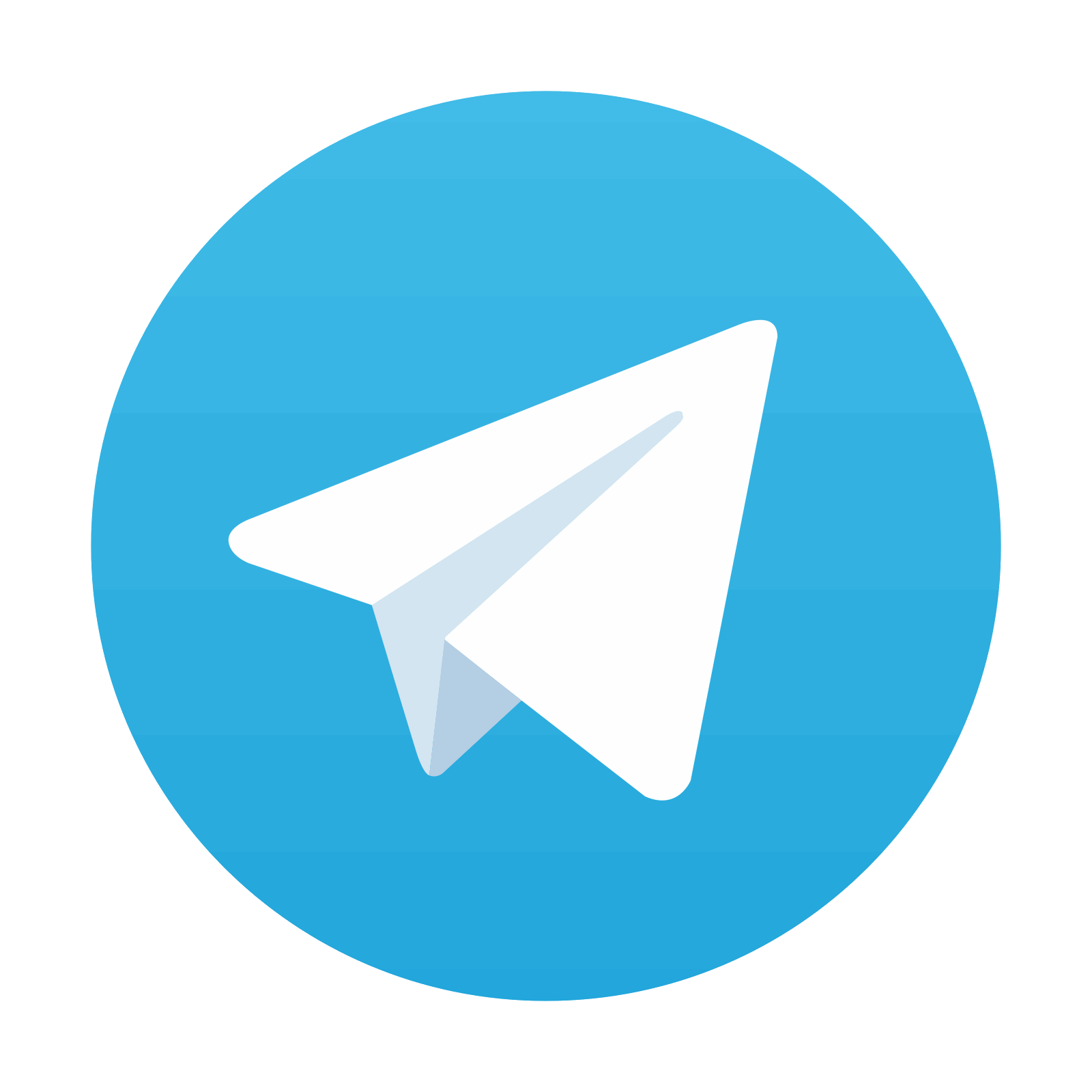
Stay updated, free articles. Join our Telegram channel

Full access? Get Clinical Tree
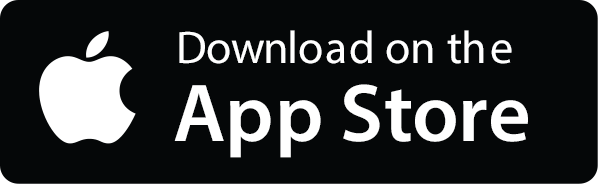
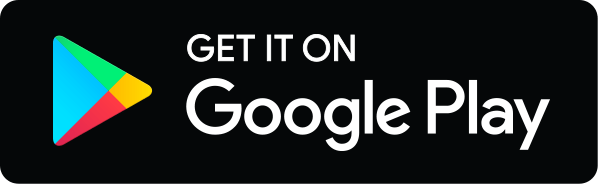