Background
Right ventricular (RV) function augments with exercise, and loss of this adaptive ability often determines symptoms. Reports on exercise-related changes in RV function in healthy subjects are sparse. In this study, healthy volunteers were prospectively recruited, and changes in RV function with exercise were examined, optimal parameters determined, and the effects of gender and age examined.
Methods
Treadmill exercise stress echocardiography with concurrent expired gas analysis was performed in 121 healthy volunteers. Parameters of RV systolic function (RV fractional area change, Doppler tissue s′ velocity, and systolic strain and strain rate) and diastolic function (peak E and A velocity, Doppler tissue e′, a′ and early and late diastolic strain rate) were evaluated at baseline and after exercise, with the difference (Δ) being systolic and diastolic reserve. Changes in pulmonary arterial pressure (PAP) was measured when accurate estimation was possible.
Results
Most systolic functional parameters were augmented with exercise. However, systolic augmentation decreased with age (Δs′: r = −0.31, P < .01; Δ strain: r = −0.28, P = .008; Δ systolic strain rate: r = −0.31, P < .01). Similar changes were observed with diastolic function (Δe′: r = −0.33, P < .01; Δ early diastolic strain rate r = −0.20, P = .04). In the subgroup with PAP measurements, ΔPAP ( r = 0.32, P < .01) increased with age. Men had greater augmentation of systolic reserve, but differences were negated when corrected for workload. S′ velocity was the most robust measure of RV systolic function.
Conclusions
There is a modest yet significant reduction in RV systolic and diastolic reserve with age, with an increase in PAP. S′ velocity is a robust and feasible measure that should be considered given the increasing use of stress testing to evaluate RV function.
Echocardiographic evaluation of the right ventricle is limited by its complex geometry and retrosternal position. Alterations in right ventricular (RV) function are important prognostic markers in conditions affecting the right ventricle, such as in elite athletes and in pulmonary arterial hypertension (PAH). Evaluation of RV function is additionally relevant in conditions affecting the left ventricle, such as heart failure, valvular disease (particularly mitral regurgitation), and acute myocardial infarction.
More recently, the ability of the right ventricle to adapt to exercise was shown to be a determinant for symptom severity as well as survival in patients with PAH. Furthermore, the exaggerated increment in pulmonary arterial pressure (PAP) may also predict adverse outcomes in heart failure, PAH, and valvular heart disease. Additionally, exercise RV dysfunction has had additional value, as it correlates with exercise capacity in heart failure and valvular heart disease.
Evaluation of RV function with exercise is important especially if resting hemodynamics do not account for patients’ symptoms. Exercise places a greater demand on the right ventricle than the left ventricle because of several physiologic constraints. First, RV work at rest is low, because of low resistance and a highly compliant pulmonary vascular bed. With exercise, because of the limited reserve of the pulmonary vascular bed, there is a greater proportional increase in vascular pressures to enable the increases in cardiac output needed for exercise. However, our understanding of RV changes with exercise is relatively limited: previous studies have used varying exercise protocols, with the impact of age and gender in healthy subjects being largely unknown. Moreover, no details on optimal parameters for use with exercise evaluation of the right ventricle have been reported.
Our aims were to determine the impact of exercise on RV systolic and diastolic function in a healthy population using routine as well as newer echocardiographic parameters (tissue Doppler and strain) with treadmill exercise testing, which is available in most centers, and to determine the most feasible echocardiographic parameter to determine exercise-related alterations in RV function. We hypothesized that systolic and diastolic function would augment with exercise and that (1) these changes may be reduced with age and (2) gender would have no specific impact on changes in RV function with exercise once adjusted for workload.
Methods
Participants
The study protocol were approved by the research ethics committees of the University of New South Wales and the Sydney South West Area Health Service. Written consent was obtained from all participants. We prospectively recruited 121 healthy volunteers for participation in this protocol; no subject was a referral to the echocardiography laboratory. A detailed history was obtained and a physical examination performed. Subjects were excluded if they were previously or currently on cardioactive medications, had any cardiovascular risk factors (smoking, hypertension, hypercholesterolemia, and diabetes mellitus), or had histories of ischemic or valvular heart disease, cerebrovascular or peripheral vascular disease, chronic lung disease (chronic obstructive pulmonary disease, asthma, or sleep apnea), significant arrhythmias, or histories of palpitations. Resting pulse rate measurement, blood pressure measurement (average of two recordings), and 12-lead electrocardiography were performed. Resting RV parameters of this cohort have been previously reported.
Exercise Stress and Cardiopulmonary Testing
Cardiopulmonary testing was performed to maximum exercise tolerance on a graded treadmill (GE T-2100; GE Healthcare, Milwaukee, WI) test using a symptom-limited Bruce protocol. Breath-by-breath expired gas analysis of the volume and expired gas concentration was performed using a calibrated gas analysis system (Cosmed; Quark PFT, Rome, Italy). Arterial oxygen saturation (finger probe sensor) and heart rate and rhythm were monitored continuously (Marquette; GE Healthcare). Workload (metabolic equivalents) was estimated from maximum oxygen consumption achieved during expired gas analysis.
Echocardiographic Measurements
Transthoracic echocardiography was performed using the Vivid 7 system (GE Healthcare) by one of three experienced sonographers, with images obtained from standard views (parasternal, apical, and subcostal views). The RV-focused view recommended by the American Society of Echocardiography was also obtained ( Figure 1 A). Five cardiac cycles were stored digitally and analyzed offline. After exercise, images and measurements were only obtained from the RV-focused view to minimize acquisition time. Postexercise data were obtained within 90 sec of the completion of exercise.

RV dimensions were measured in accordance with ASE guidelines. At baseline and after exercise, RV end-systolic and end-diastolic areas were measured and fractional area change (FAC) derived. Images were acquired at end-expiration to minimize variability in RV size with respiration. Pulsed Doppler tissue imaging (DTI) RV s′ velocity was obtained from the lateral RV annulus. Tricuspid regurgitant (TR) velocity was also measured after exercise ( Figures 1A–1C ).
RV diastolic function was assessed as transtricuspid peak E and A velocities and E/A ratio. Baseline and postexercise DTI RV lateral annular e′ and a′ velocities were obtained and the RV E/e′ ratio calculated.
In the subgroup of subjects in whom the TR trace could be assessed adequately, systolic PAP was estimated at rest and after exercise from the TR velocity using the Bernoulli equation with the addition of right atrial pressure estimated from the inferior vena cava diameter and collapsibility obtained at rest.
RV systolic and diastolic function was also evaluated by two-dimensional (2D) strain analysis from the RV-focused view (>60 frames/sec), at rest and immediately after exercise. Two-dimensional strain measurements were obtained offline using a two-step tracking algorithm with software used for the left ventricle (EchoPAC; GE Healthcare). A region of interest was manually traced along the RV endocardial border (septal and lateral basal, middle, and apical segments) in end-systole ( Figure 2 A), with its width adjusted to RV wall thickness, excluding pericardium and left ventricular endocardium. Peak systolic strain, systolic strain rate (S-Sr), and early diastolic strain rate (E-Sr) and late diastolic strain rate (A-Sr) curves were derived ( Figure 2 B). Strain measurements were included only if all three RV lateral wall segments were approved by software analysis, as previously described. Global strain and strain rate were the averages of the three (basal, middle, and apical) RV free wall segments. All values are averages of three consecutive cardiac cycles.

RV systolic reserve was defined as augmentation in RV systolic function (i.e., the differences between resting and postexercise, denoted Δ, of FAC, s′, strain, and S-Sr). Similarly, diastolic reserve was defined as the Δ values of e′ and E-Sr. The percentage changes in these variables were also examined to account for the baseline magnitude of the parameter.
The subjects were also divided into three two-decade groups (20–39 years, 40–59 years, and ≥60 years), and RV systolic function parameters were evaluated in the three groups. In a subset of 20 patients, resting and postexercise measurements was performed by the same observer, ≥4 weeks apart. The measurements were repeated by a second observer blinded to the initial results.
Statistical Analysis
Analysis was performed using SPSS version 16 (SPSS, Inc, Chicago, IL). All values are expressed as mean ± SD.
The Kolmogorov-Smirnov test verified the normality of distribution of the variables. Paired t tests were used to analyze significant differences between resting and postexercise variables. Linear regression was used to evaluate the relationship of RV function with age. Stepwise multiple regression analysis of significant univariate and clinically important parameters was used to determine the independent predictors of changes in parameters with exercise. Student’s t tests were used to analyze gender differences. P values < .05 were considered to indicate statistical significance. Additionally, the cohort was divided into three two-decade groups (group 1, 20–39 years; group 2, 40–59 years; and group 3, ≥60 years) for further comparison of systolic function using one-way analysis of variance with post hoc Bonferroni correction to evaluate group differences. Univariate analysis was also performed after adjusting for workload achieved to examine the relationship of Δ and percentage change of the variable with age.
Intra- and interobserver variability is expressed using intraclass correlation coefficients and coefficients of variation.
Results
Clinical and Echocardiographic Characteristics
Of the 121 subjects (mean age, 42 ± 13 years; range, 20–81 years; 51% men), RV lateral s′ velocity was obtained in all subjects at rest and in 118 subjects (97.5%) after exercise. Strain analysis could not be performed in 10 subjects (4.5%) at rest and in 31 (25.6%) after exercise, because of suboptimal image quality, especially after exercise. Additionally, TR traces were inadequately obtained in six of 121 subjects (5%) at rest and 22 of 121 (18%) after exercise. All subjects had normal estimated right atrial pressures at rest (5 mm Hg), and this value was also used after exercise. Postexercise measurements were derived at a mean heart rate of 130 ± 16 beats/min, which represented 75.0 ± 7.6% of the peak heart rate achieved.
There was no significant difference in demographic variable with age, as previously reported ( Table 1 ). There were increases in systolic blood pressure and mean arterial pressure with exercise ( Table 2 ). Although resting heart rate did not vary with age, peak heart rate achieved after exercise decreased with age. Heart rate reserve (Δ heart rate) decreased with increasing age, as did exercise duration and consequently maximum workload achieved.
Variable | Value | r | P |
---|---|---|---|
Men | 62 (51%) | ||
Body surface area (m 2 ) | 1.83 ± 0.19 | 0.04 | NS |
Height (cm) | 167 ± 9.0 | 0.15 | NS |
Weight (kg) | 71.2 ± 13.2 | 0.03 | NS |
Maximal workload (METs) | 11.9 ± 3.8 | −0.37 | <.001 |
Exercise time (min) | 10.0 ± 3.8 | −0.35 | <.001 |
Peak V o 2 (mL/kg/min) | 30.3 ± 8.6 | −0.44 | <.001 |
Variable | Rest | Exercise | P | ||
---|---|---|---|---|---|
Mean ± SD | n | Mean ± SD | n | ||
Systolic BP (mm Hg) | 118 ± 11 | 121 | 168 ± 18 | 121 | <.001 |
Diastolic BP (mm Hg) | 73 ± 9 | 121 | 75 ± 9 | 121 | <.001 |
Mean arterial pressure (mm Hg) | 88 ± 8 | 121 | 107 ± 10 | 121 | <.001 |
Heart rate (beats/min) | 77 ± 11 | 121 | 174 ± 17 | 121 | <.001 |
RV systolic function | |||||
RV FAC (%) | 42.6 ± 5.8 | 121 | 53.7 ± 6.4 | 121 | <.001 |
RV strain (%) | 27.3 ± 3.3 | 111 | 28.4 ± 7.8 | 89 | <.001 |
s′ (cm/sec) | 12.1 ± 1.3 | 121 | 20.8 ± 3.1 | 118 | <.001 |
RV diastolic function | |||||
e′ (cm/sec) | 11.5 ± 2.6 | 121 | 15.3 ± 5.0 | 118 | <.001 |
a′ (cm/sec) | 10.8 ± 2.8 | 121 | 21.7 ± 6.6 | 118 | <.001 |
RV e/e′ ratio | 4.39 ± 1.05 | 121 | 5.06 ± 1.54 | 118 | <.001 |
E-Sr (sec −1 ) | 1.92 ± 0.43 | 111 | 2.84 ± 1.01 | 89 | <.001 |
A-Sr (sec −1 ) | 1.50 ± 0.33 | 111 | 2.49 ± 0.89 | 89 | <.001 |
Hemodynamics | |||||
Systolic PAP (mm Hg) | 20.9 ± 6.6 | 115 | 26.5 ± 10.6 | 99 | <.001 |
RV systolic function was augmented after exercise, with increases in all systolic functional parameters. However, systolic augmentation of the right ventricle decreased with age, with a negative correlation of Δ s′ velocity, Δ strain and ΔS-Sr ( Figure 3 ). Δs′ and ΔS-Sr correlated modestly with workload ( r = 0.22 and r = 0.21, P < .05, respectively). Δ strain showed no correlation with workload, while ΔFAC showed no correlation with age or workload ( Table 3 ).

Variable | Rest | Postexercise | Δ | ||||
---|---|---|---|---|---|---|---|
r | P | r | P | Mean ± SD | r | P | |
Systolic BP (mm Hg) | 0.41 | <.001 | 0.24 | .01 | 51 ± 14 | −0.05 | NS |
Diastolic BP (mm Hg) | 0.21 | <.001 | 0.16 | NS | 3 ± 8 | −0.03 | NS |
Mean arterial pressure (mm Hg) | 0.33 | <.001 | 0.23 | .01 | 19 ± 7.4 | −0.13 | NS |
Heart rate (beats/min) | −0.24 | NS | −0.71 | <.001 | 97 ± 17 | −0.54 | <.001 |
RV systolic function | |||||||
RV FAC (%) | −0.06 | NS | −0.18 | NS | 10.9 ± 7.7 | −0.07 | NS |
Strain (%) | −0.23 | .02 | 0.05 | NS | 1.37 ± 7.24 | 0.16 | NS |
s′ (cm/sec) | −0.43 | <.001 | −0.37 | <.001 | 8.6 ± 3.0 | −0.31 | .004 |
S-Sr (sec −1 ) | −0.23 | .02 | −0.22 | .02 | 1.4 ± 0.8 | −0.31 | .004 |
Diastolic function | |||||||
e′ (cm/sec) | −0.48 | <.001 | −0.40 | <.001 | 4.5 ± 4.1 | −0.33 | .002 |
a′ (cm/sec) | 0.46 | <.001 | 0.04 | NS | 11.1 ± 6.5 | −0.26 | .01 |
RV e/e′ ratio | 0.04 | NS | 0.23 | .02 | 0.68 ± 1.45 | 0.21 | .02 |
E-Sr (sec −1 ) | −0.31 | .001 | −0.23 | .02 | 0.97 ± 0.84 | −0.20 | .04 |
A-Sr (sec −1 ) | 0.40 | <.001 | −0.18 | NS | 0.98 ± 0.88 | −0.36 | .001 |
Hemodynamics | |||||||
Systolic PAP (mm Hg) | 0.36 | <.001 | 0.56 | <.001 | 7.38 ± 8.8 | 0.32 | .003 |
Similarly diastolic function was augmented after exercise, with increases in peak E, e′, and E-Sr, with a negative correlation with age ( Figure 3 ). A positive correlation with age was seen with RV e/e′ after exercise ( Table 3 ). There was also a correlation of diastolic reserve (Δe′) with workload achieved ( r = 0.21 P < .05).
Univariate predictors of Δs′ were age, gender, body surface area, ΔPAP, heart rate reserve, and maximal heart rate achieved. Stepwise multivariable analysis performed using univariate predictors of Δs′ demonstrated that heart rate reserve was the strongest predictor of Δs′ velocity ( r = 0.39, r 2 = 0.15, P < .001); however, when Δ heart rate was excluded, age and gender were the only independent predictors ( r = 0.37, P < .001). Similarly for ΔS-Sr, heart rate reserve was the strongest predictor ( r = 0.40, P < .001); however, when excluded, age and gender were again the only significant predictors ( r = 0.31, P = .001).
When systolic reserve was assessed after adjusting for workload, FAC ( r 2 = 0.60, P = .02), s′ ( r 2 = .54, P = .05), strain ( r 2 = 0.62, P = .05), and S-Sr ( r 2 = 0.64, P = .008) showed significant relationships with age, indicating that systolic reserve was still influenced by age regardless of workload (i.e., oxygen consumption achieved). However, the percentage changes of the systolic parameters were not influenced by age (s′, r = −0.15; strain, r = −0.17; S-Sr, r = −0.15; P = NS for all) when adjusted for workload.
Stepwise multivariate analysis for Δe′ showed that age was the only univariate predictor ( r = 0.33, P < .001); however, both age and gender were predictors of ΔE-Sr ( r = 0.27, P = .006).
The two-decade group analysis ( Figure 4 ) of systolic function revealed that resting systolic function decreased early (i.e., between groups 1 and 2 for resting strain [ P < .001] and S-Sr [ P = .04]). With exercise, however, this early alteration in systolic function was magnified and seen with s′ ( P = .001) and strain ( P < .001). Unfortunately, postexercise S-Sr failed to demonstrate a significant difference.
Pulmonary Pressure
The TR trace could not be adequately obtained in a subgroup ( n = 6 [5%] at rest and n = 22 [18%] after exercise), and PAP analysis was not performed in these subjects. When the results of PAP analysis ( n = 115 [95%] at rest, n = 99 [82%] after exercise) were examined, resting PAP, postexercise PAP, and ΔPAP correlated significantly with age ( Figure 5 ). Both postexercise PAP and ΔPAP correlated with age, even after indexing to workload achieved ( r = 0.57 [ P < .001] and r = 0.36 [ P < .001], respectively).
ΔPAP correlated negatively with exercise duration ( r = −0.31, P = .003) and workload achieved ( r = −0.33, P = .002). Interestingly, subjects with greater ΔPAP also had a diminished ability to augment their RV systolic and diastolic function, with negative correlations with Δs′ ( r = −0.24, P = .02) and Δe′ velocity ( r = −0.20, P = .04).
Gender Differences
Men had higher body surface areas and mean arterial pressures ( Table 4 ), exercised longer, and achieved higher maximal heart rates. There were significant gender-related differences: men had greater augmentation of RV systolic function (ΔFAC, Δs′, and ΔS-Sr) ( Table 4 ) and of diastolic function (Δe′ and ΔE-Sr). Similarly, when we looked at the percentage change in the variable, the gender differences remained, except for S-Sr. However, when these functional parameters were corrected for workload (metabolic equivalents achieved), no significant differences in systolic or diastolic reserve were present on the basis of gender.
Variable | Female ( n = 59) | Male ( n = 62) |
---|---|---|
Age (y) | 43.6 ± 12.8 | 40.0 ± 12.6 |
BSA (m 2 ) | 1.67 ± 0.14 | 1.92 ± 0.16 ∗ |
Exercise time (min) | 8.5 ± 2.8 | 11.5 ± 3.2 ∗ |
Maximum heart rate achieved (beats/min) | 167 ± 15 | 180 ± 16 ∗ |
Maximum workload achieved (METs) | 10.0 ± 3.2 | 13.6 ± 3.5 ∗ |
Δ mean arterial pressure (mm Hg) | 17.7 ± 7.4 | 20.2 ± 7.7 |
ΔPAP (mm Hg) | 9.8 ± 7.0 | 6.0 ± 8.5 ∗ |
ΔFAC (%) | 8.0 ± 6.6 | 13.6 ± 4.3 ∗ |
Δ RV S′ (cm/sec) | 7.9 ± 2.7 | 9.3 ± 3.1 ∗ |
Δ RV e′ (cm/sec) | 3.6 ± 3.9 | 5.3 ± 4.1 ∗ |
ΔS-Sr (sec −1 ) | 1.2 ± 0.7 | 1.6 ± 0.8 ∗ |
ΔE-Sr (sec −1 ) | 0.7 ± 1.0 | 1.1 ± 0.9 ∗ |
% change in s′ | 67.5 ± 24.5 | 76.4 ± 26.1 ∗ |
% change in strain | 22.2 ± 12.1 | 16.8 ± 11.2 ∗ |
% change in S-Sr | 44.1 ± 21.1 | 50.1 ± 19.0 ∗ |
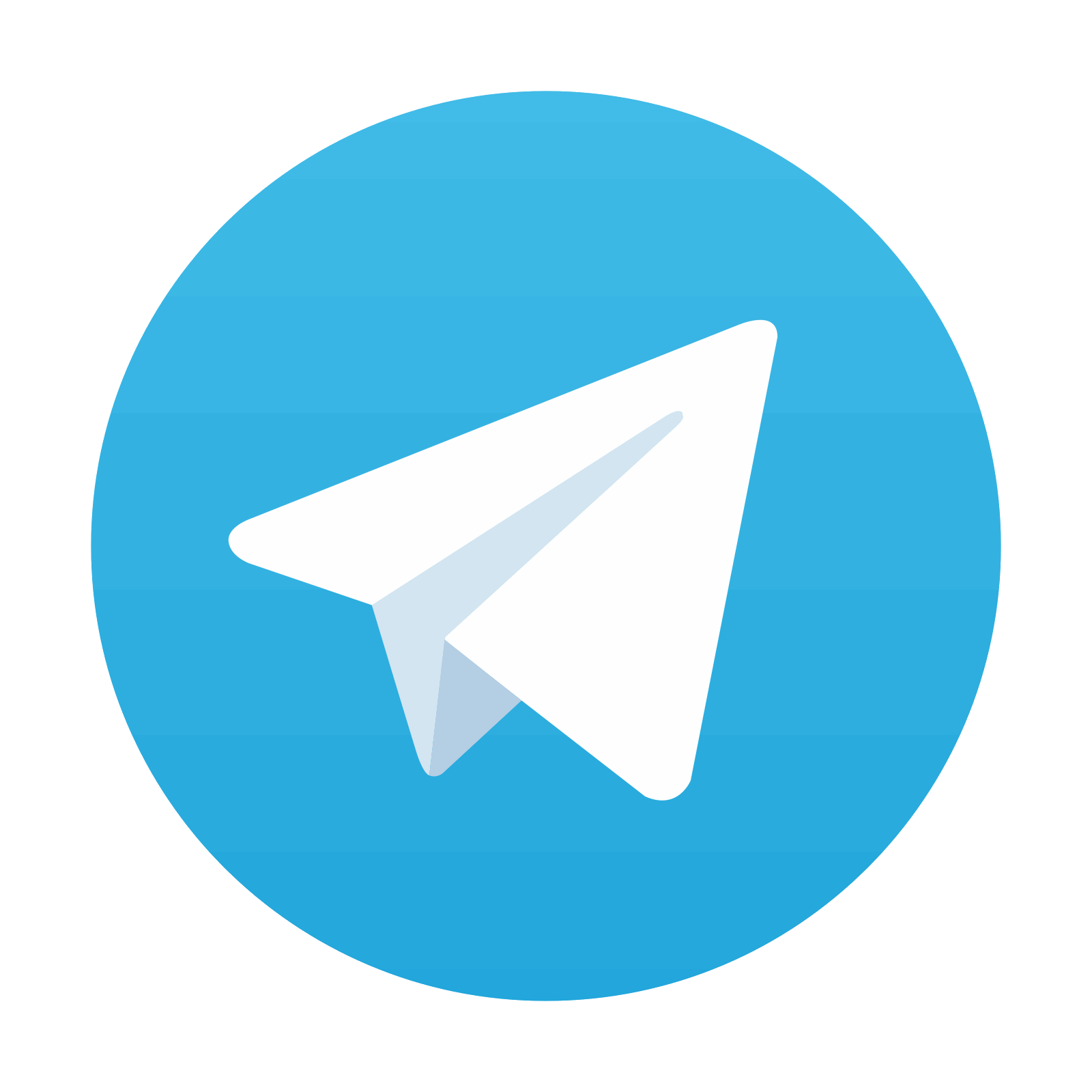
Stay updated, free articles. Join our Telegram channel

Full access? Get Clinical Tree
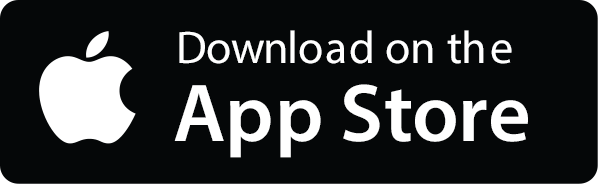
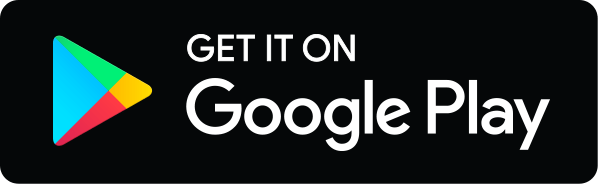
