CHANGES IN OXYGEN SATURATIONS DURING THE FIRST FEW MINUTES OF LIFE IN THE DELIVERY ROOM
Introduction
Physiological events that occur during transition from fetal to extra-uterine life at birth have attracted the attention of investigators for several decades. Because of the critical life-threatening possibilities during this transition, the period from birth to first breath was labeled as “Period of Grace” by Smith1 more than half a century ago. This reflected the fact that many children never manage to adapt to extra-uterine life and succumb immediately after birth without additional help. Sadly, it is true even today in many developing countries around the world. However, the advances in perinatal and neonatal medicine gained during the last century are making their impact on better neonatal outcome worldwide.
The physiology of fetal circulation and changes during transition has been discussed in detail in the first chapter of this book.2 Nevertheless, it is pertinent to review these critical changes to better understand the principles of neonatal resuscitation in the delivery room.3 Resuscitation of the newborn is dependent on initiation of respiration and effective oxygenation soon after birth. Until recently, assessment of the improvement in oxygenation of the newborn during resuscitation was mainly based on clinical observation of the newborn.4 Clinical observation alone may be inaccurate in judging the degree of oxygenation. O’Donnell et al have shown that clinical assessment of oxygenation based on color can be inaccurate.5 The innovative noninvasive method of assessing oxygenation using pulse oximetry and its simplicity in application in the newborn has made it possible to measure oxygenation continuously during transition soon after birth. Pulse oximetry can also be used to assess oxygenation during neonatal resuscitation.3,6 In this chapter, we will review the physiological aspects of cardiopulmonary changes and the value of pulse oximetry of the newborn during the first minutes of life in assessing the normal and abnormal transition that occurs soon after birth.
Fetal Circulation
Fetal circulation is distinguished by its unique physiology.7,8 Fetal blood is oxygenated at the placental level, while the fetal lung remains dormant in its function as an organ of oxygenation. The main features of fetal circulation are: oxygenated blood is carried by the umbilical vein from the placenta to the right atrium and deoxygenated blood is carried by the umbilical arteries (UAs) to the placenta. Three shunts are functional during fetal circulation, namely, the ductus arteriosus (DA), ductus venous (DV), and foramen ovale (FO). High pulmonary vascular resistance (PVR) and low placental resistance essentially help in exchange of gases at the placenta. The mechanisms for the presence of a high degree of fetal PVR have been explained on the basis of active and passive resistance.8–10
Passive resistance is due to compression of pulmonary capillaries by fetal lung fluid. The active vasomotor tone secondary to the hypoxic level of pulmonary venous blood is mainly responsible for pulmonary vasoconstriction in the fetus.11,12 Because of the high PVR, most of the blood pumped from the right ventricle (RV) enters the descending aorta (DAo) through the DA for distribution to the lower part of the body and the placenta via UAs for oxygenation.
Transitional Neonatal Circulation
Dramatic changes occur during fetal to neonatal transition at birth to adapt to extra-uterine life. These events follow initiation of the first breath of the newborn. The first breath of the newborn is triggered both by physiological interaction of low oxygen levels and high CO2 and by environmental changes that stimulate the respiratory center. With subsequent breaths, lungs continue to expand until normal functional residual capacity (FRC) is established. Concomitantly, there is a marked decrease in PVR, resulting in increased blood flow from the RV to the lungs. The decrease in PVR increases the blood flow to the left atrium. The pressure in the left atrium increases, which closes the FO by pressing the valve of the FO against the septum secundum. The FO closes functionally at birth.13,14
As the PVR is now lower than the systemic resistance, blood flow in the DA reverses, passing from the aorta to the pulmonary trunk. The DA constricts at birth, but there is often a small left-to-right shunt for 24 to 48 hours in a normal, healthy infant. Several events promote ductus constriction in the full-term newborn. These include an increase in arterial partial pressure of oxygen, a decrease in blood pressure within the ductus (due to postnatal decrease in PVR), a decrease in circulating prostaglandin (PG) E2 (due to loss in placental PG production and increase in PG removal by the lung), and a decrease in the number of PGE2 receptors in the ductus wall. Fifty percent of ducts are functionally closed at the end of 24 hours, 90% by 48 hours, and 100% by 72 hours. Anatomical closure of the ductus and formation of the ligamentum arteriosum occurs by the twelfth week.
Transitional Pulmonary Physiology: The Science of First Breath
It is important to note that circulatory changes that occur alone during transition do not completely explain improved oxygenation soon after birth. In order to sustain oxygenation after birth and placental separation, parallel to circulatory changes, critical changes take place in the previously dormant lung during the first few breaths of the newborn. Two major developmental changes in the lung prepare the fetal lung for extra-uterine gas exchange. First, lung in utero is not a completely collapsed organ that is filled with lung fluid. The mechanisms of production and constituents of lung fluid have been well studied. Lack of production of lung fluid or loss of lung fluid from chronic leakage is associated with hypoplasia of the lung with consequent difficult neonatal transition. Second, although not consequential in oxygen delivery, fetal breathing movements are considered important for the newborn to have smooth fetal to neonatal transition. Fetal breathing movements start midway through gestation. These are intermittent breathing movements lasting for varying periods, but about 20 minutes every hour near term. Sensory stimulation and cooling of the skin, changes in the arterial oxygen and carbon dioxide levels, and detachment of the placenta are factors found to be responsible for the onset of continuous breathing after birth.15–17
In normal fetus-to-neonatal transition, replacement of fetal lung fluid with air is an important step toward establishment of smooth respiration. Karlberg in 1959 and Milner in 1978 successfully conducted studies on newborns to understand changes that occur with the first breath.18,19 They studied air flow, pressure, and volume changes in the lung using an intraesophageal catheter, a face mask, and a low-resistance pneumotach. In addition, they also took serial chest x-rays starting at the time of birth and during initiation of first breath and continued to take chest x-rays during subsequent breaths until establishment of sustained ventilation. They found that with the first inspiration, the pressure generated varied from 10 to 70 cm H2O and the inspiratory volume was 20 to 75 mL. Expiration was passive, and there was no pause at the end of inspiration. Residual volume varied from 0 to 70 ml and subsequent earliest breaths required lower intrathoracic pressures. The total respiratory work was equal to the square of tidal volume. The total inspiratory work during the first 3 breaths showed no difference. A significant correlation has been shown between first inspiratory volume and FRC at the end of the first breath (P < 0.004). Figure 8.1 shows the sequential changes that occur in inspiratory pressure, fluid in alveoli, DA, and FO during the “First Breath” and subsequent breaths. Full inflation and prolonged expiration associated with the baby’s crying drives out nearly 100 ml of fluid with the first few breaths. A healthy newborn when taking the first breath after birth exerts a negative intrathoracic pressure of approximately 50 cm H2O (0–100 cm H2O) and with adequate surfactant supply will develop an FRC of approximately 5 to 6 ml/kg.18,19 There is increased production of adrenaline by fetus and thyrotropin-releasing hormone and glucocorticoids by the mother with onset of labor that stimulates reabsorption of fluid by pulmonary epithelial cells. During vaginal delivery at term, up to 35 ml of fluid is expelled from the lungs by uterine contraction and passage through the birth canal. Similar findings were observed by other investigators in various experimental models.20–25
Figure 8.1. The figure shows diagrammatically the sequential changes in 4 physiological parameters:
- Left vertical panel. Pressure (P)–volume (V) loop during the “First Breath” starting in the top portion to the lower panel. Note that the lungs open up only after reaching a certain opening pressure. Functional residual volume is established at the end of the first breath (third panel from top), facilitating the easier second and subsequent breaths.
- The second vertical panel from the left shows changes in the alveoli starting from the top. The liquid-filled alveoli open up with increasing intra-alveolar pressure as seen in the pressure/volume (PV) curve, eventually establishing functional residual volume and formation of an air liquid phase with a monolayer of surfactant lining the alveoli (bottom). Note that the horizontal lines below the alveolus depict pulmonary vessels and blood flow. With an increase in alveolar pressure, there is an increase in PBF.
- The third vertical panel diagrammatically shows changes in PBF and patency of PDA before the first breath and subsequent breaths. See text for details.
- The fourth vertical panel shows changes in the left atrial filling and closure of the FO prior to and following the “First Breath” (top to bottom). See text for details.
Sequential changes with first and subsequent breaths. Note that less pressure is required to open the alveolus with subsequent breaths, air displaces fluid from the alveolus with each breath, and the DA and FO close.
In summary, with the first breath there is a mechanical expansion of lungs, fluid from the alveoli is pushed out and absorbed into the lymphatics. With subsequent breaths alveoli get filled with air that contains 21% oxygen. As a result of gaseous distension and increased oxygen in the alveoli, blood vessels in the lungs relax, pulmonary blood flow (PBF) increases, and well-oxygenated blood is pumped for the whole body. These pulmonary changes in conjunction with improved pulmonary circulation establish normal postnatal circulation and oxygenation.
Abnormal Cardiopulmonary Transition and Oxygenation
Compromised and at-risk newborn infants born by cesarean section, and premature infants are prone to experience difficulties in establishing normal transition at birth. The consequent burden of birth asphyxia is a major concern. Prevention requires attention to proper resuscitation in the delivery room. It is estimated that 10% to 15% of babies require resuscitation in the delivery room. At the global level, it is estimated that 1 million babies may require resuscitation to establish transition and normal oxygenation for the baby to prevent hypoxia. With this background, the American Heart Association (AHA) and the American Academy of Pediatrics (AAP) developed the Neonatal Resuscitation Program (NRP) to train healthcare workers to gain skills to provide proper resuscitation in the delivery room.3,4 The main objective was to establish normal breathing and adequate oxygenation. Clinical observation of color is the main guide to assess neonatal oxygenation. However, clinical assessment of oxygenation in the newborn has been proven to be inadequate.5 Then, the invasive method of blood gas monitoring became the standard way to precisely monitor blood PaO2. The introduction of the noninvasive method of pulse oximetry decades ago opened the door to monitor blood oxygenation continuously in newborns. Now pulse oximetry is a standard method of monitoring the newborn in the Neonatal Intensive Care Unit (NICU). Indeed, it is considered to be the important “fifth vital sign” Vital sign after heart rate, respiratory rate, blood pressure, and temperature.26 However, the use of pulse oximetry in the delivery room to assess the degree of desaturation and guide resuscitation of the newborn in the delivery room is a relatively new practice. In recent years, several investigators have demonstrated the importance of pulse oximetry in the delivery room to assess oxygenation of the newborn after the first breath.27–39 We shall in the following pages provide a review of studies done and the current knowledge of pulse oximetry in the delivery room.
What is Pulse Oximetry?
Pulse oximetry is a noninvasive method to measure the level of blood oxygen saturation (SpO2).40,41 It consists of a small light-emitting diode (LED) and a photodiode, which are placed opposite each other with the translucent site (eg, palm, wrist, sole, or finger in newborns) in between the 2 diodes. The LED transmits red light of wavelength 660 nm and infrared light of wavelength 905, 910, or 940 nm, which is received by the photodiode on the opposite side. The absorption of red and infrared light by oxyhemoglobin and deoxyhemoglobin present in the blood between the 2 diodes varies at these wavelengths, which is calculated as the R/IR ratio and converted into the SpO2 value by comparing with the look-up tables given by the manufacturer.
The signal varies with each myocardial contraction due to the surge of blood through the arteries, which increases the blood volume at the measuring site. This is displayed in the waveform on the monitor. By subtracting minimum absorption (due to the constant presence of skin, soft tissue, and venous blood at the site) from peak absorption (due to the surge in blood volume), a monitor can show only the absorption caused by arterial blood. Hence, presence of a pulse is important for functioning of the pulse oximeter.
The major drawbacks of conventional pulse oximetry are obtaining reliable data during movements of the limbs and low perfusion at the measuring site, the reason being difficulty in distinguishing pulsating arterial blood from moving venous blood. In 1995, Massimo introduced signal extraction technology (SET) that could accurately extract the arterial signal from the venous signals and thus overcame problems due to motion artifacts.
Oxygen in the blood is carried in 2 forms, 98% to 99% bound to hemoglobin (oxyhemoglobin) and the rest dissolved in the blood plasma (PO2). Together, oxygen content is represented in ml O2/100 ml of whole blood. Tissues utilize the dissolved form of oxygen. The oxygen hemoglobin dissociation sigmoid-shape curve explains the relationship between these 2 forms of oxygen. Oxygen bound to the hemoglobin is released into the blood plasma and absorbed into the tissues. Each hemoglobin molecule has the capacity to carry 4 oxygen molecules. Oxygen saturation is the ratio of the amount of oxygen bound to hemoglobin, to the oxygen-carrying capacity of hemoglobin, which is expressed in percentage. Various physiological factors in the newborn period affect the oxygen dissociation curve. Fetal hemoglobin has a high affinity for oxygen; as such, when fully saturated, there is a high percentage of oxyhemoglobin. This shifts the curve to the left.
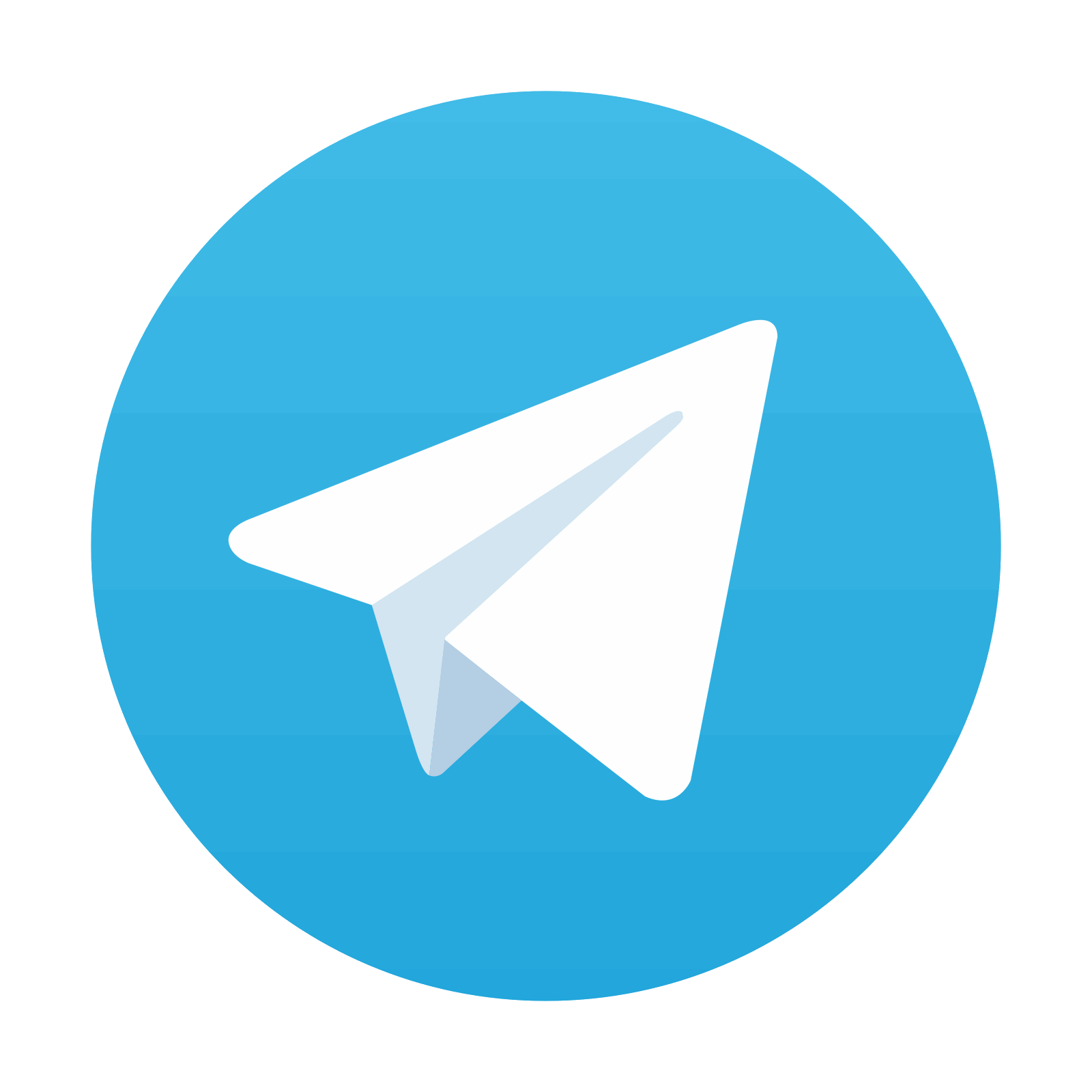
Stay updated, free articles. Join our Telegram channel

Full access? Get Clinical Tree
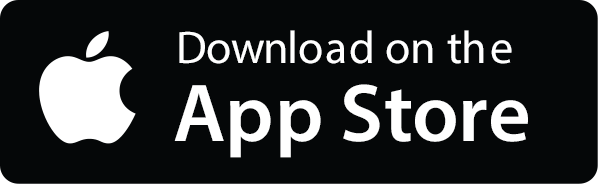
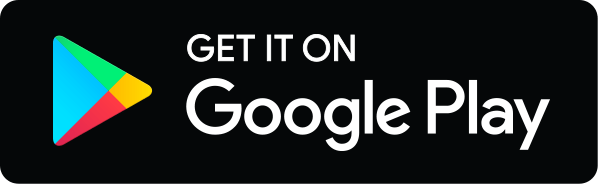