Cardiopulmonary and Right–Left Heart Interactions
Andrew N. Redington
The singular function of the right ventricle (RV), left ventricle (LV), and the lungs is discussed elsewhere. Nonetheless, particularly in the field of congenital heart disease, we are becoming increasingly aware of the importance of the interactions between them. There is continual cross talk between the two sides of the heart, and in turn, the ventricles are continually responding to subtle changes occurring within the thorax as a whole. In this chapter, these interactions, and their modification by congenital heart disease and its surgical repair, are discussed.
Comparison of Right and Left Heart Hemodynamics
The average cardiac output from the RV must, of course, essentially equal the cardiac output from the left heart. Nonetheless, the mechanism by which this is achieved is very different. The RV performs approximately one-quarter of the external mechanical work compared with its left ventricular (LV) counterpart. External mechanical work is a function of stroke volume and developed ventricular pressure, and is more accurately described as the area enclosed by the ventricular pressure–volume curve. Figure 26.1 shows a schematic comparison of left and right ventricular (RV) pressure–volume curves. Not only is the developed pressure substantially lower in the RV but its trapezoidal shape still further reduces the amount of work performed on the circulation to generate the cardiac output. The LV essentially works as a square wave pump, its stroke work being reasonably well represented as a direct product of its stroke volume and developed pressure (stroke work = stroke volume × peak left ventricular pressure – minimum left ventricular pressure). Because of its ability to eject blood into the pulmonary circulation during both pressure rise and pressure fall (1), the external work performed by the RV cannot be described using such a simple derivation. Furthermore, it can be seen that small changes in hemodynamics might impose a major change to global workload of the RV. Indeed, small changes in RV afterload can lead to major changes in workload and the shape of the pressure–volume characteristics to mirror the LV (2).
The trapezoidal shape of the RV pressure–volume relationship is exquisitely matched to the low hydraulic impedance imposed by the pulmonary vascular bed. Unlike the systemic vascular resistance, which reflects a dynamic balance between vasodilatory and vasoconstrictor influences, the pulmonary vascular bed appears to be maximally vasodilated. The low pulmonary vascular resistance requires a healthy endothelium and normal lung function for its integrity. In health, additional inhaled nitric oxide, for example, fails to lower the pulmonary vascular resistance, suggesting pulmonary endothelial vasodilatory capacity is at its maximum (3). This is a markedly different mechanism to that of the systemic vascular bed where a wide portfolio of vasodilatory substances can lower its resistance. The pulmonary vascular bed is also uniquely affected by external factors. The status of lung inflation, even the normal circulation, has major effects on the pulmonary vascular resistance and hemodynamic function. Normal respiration, ventilating around
functional residual capacity, minimizes the pulmonary vascular resistance. Underinflation of the lungs leads to an increased pulmonary vascular resistance, as a result of atelectasis and secondary alveolar hypoxemia, and overinflation of the lungs leads to an increase, secondary to alveolar stretch and direct vascular compression (Fig. 26.2) (4). Both should be avoided whenever RV afterload needs to be minimized.
functional residual capacity, minimizes the pulmonary vascular resistance. Underinflation of the lungs leads to an increased pulmonary vascular resistance, as a result of atelectasis and secondary alveolar hypoxemia, and overinflation of the lungs leads to an increase, secondary to alveolar stretch and direct vascular compression (Fig. 26.2) (4). Both should be avoided whenever RV afterload needs to be minimized.
Cardiopulmonary Interactions in the Normal Circulation
Descent of the diaphragm during normal inspiration leads to a modest fall in pleural pressure (3 to 5 cm of water) (5) and a concomitant rise in intra-abdominal pressure. These two changes lead to increased venous return and an increased RV stroke volume, in accord with the Frank–Starling mechanism. There is thus a waxing and waning of cardiac output of approximately 10% to 15% during the cardiac cycle (6).
The classic experiments of Cournand in the 1940s (7) were interpreted as confirmation that right heart filling and cardiac output were related to intrathoracic pressure. Positive pressure ventilation via a mask in conscious volunteers led to a fall in cardiac output of approximately 10% to 15%. This was initially thought to be entirely due to changes in systemic venous return (and reduced ventricular preload) imposed by a raised mean intrathoracic pressure. It has subsequently become clear that reduced preload, as a result of increased airway pressure, is not the whole story. In an elegant series of experiments in dogs, Henning (8) showed that restoration of preload during positive pressure ventilation failed to restore cardiac output to its baseline levels. The persistent reduction in RV stroke volume, despite normalized preload, suggested an adverse effect on intrinsic RV performance. It was hypothesized that the RV shows signs of reduced systolic function, even under the circumstances of a modest hemodynamic burden imposed by lung hyperinflation, as a result of increased afterload occurring via the mechanism described in Figure 26.2. No matter what the mechanism, however, the functional implications of these changes are not simply theoretic. In a study of children undergoing cardiac catheterization performed by Shekerdemian et al. (9), a negative pressure cuirass device was used to mimic normal ventilation to compare the effects of positive and negative pressure ventilation on cardiac output. In essentially normal children having undergone closure of a small arterial duct, for example, there is an approximate 16% fall in cardiac output, simply as a result of a modest rise in mean airway pressure (to ∼8 cm of water) secondary to positive pressure ventilation. These adverse hemodynamic effects of increased RV afterload (negative cardiopulmonary interactions) become even more important when the right heart circulation is affected by congenital heart disease.
Cardiopulmonary Interactions in Congenital Heart Disease
Given that normal right heart function is dependent on a low RV afterload, normal ventricular preload, and maintained RV systolic function, it would be surprising if congenital heart diseases did not have major effects on its performance. This is indeed the case.
Reduced Right Ventricular Contractile Performance
It has long been known that RV ischemia, in the setting of atherosclerotic coronary disease, is an extremely poorly tolerated hemodynamic burden (10). This, in part, is related to adverse right–left heart interactions (see section “The Effects of the Right Ventricle on the Left Ventricle”), but illustrates the importance of RV contractile performance, even in the presence of a relatively normal pulmonary vascular bed. A more common scenario in congenital heart disease is the adverse effect of cardiopulmonary bypass on right heart function. Brookes et al. (11) showed that even a brief period of cardiopulmonary bypass and cardioplegic arrest, during coronary bypass surgery, leads to a significant decline in RV systolic performance, as assessed by end-systolic elastance. This translates to an even greater dependence on cardiopulmonary interactions in children undergoing congenital heart surgery. Shekerdemian (9), in the study described above, showed that positive pressure ventilation had an even greater adverse effect in such patients. Compared with negative pressure ventilation, there was on average a 25% fall in cardiac output with positive pressure ventilation in children on the intensive care unit after simple right heart surgery (ventricular septal defect [VSD], atrial septal defect [ASD], etc.).
Cardiopulmonary Interactions and the Abnormal Right Heart
The potential for beneficial and adverse cardiopulmonary interactions is greater when the right heart is intrinsically abnormal because of congenital anomalies or is primarily affected by surgery. There are two circumstances in which these concepts are exemplified, those patients with abnormal RV diastolic function and those with exclusion of the RV from the venopulmonary circulation.
Abnormal Right Ventricular Diastolic Function
It is now known that restrictive RV physiology is a common sequel of surgery for hypoplasia of the right heart, for example, pulmonary atresia with intact ventricular septum (12), and after repair of tetralogy of Fallot. The characteristic physiology of these patients is the presence of antegrade diastolic pulmonary blood flow during atrial systole. This occurs when the resistance to RV filling exceeds the pulmonary vascular resistance. Atrial contraction ejects blood through the tricuspid valve, but there is little or no RV filling as the blood passes through the RV (which acts as a passive conduit) to the pulmonary artery (PA). Consequently, up to one-third of the antegrade pulmonary blood flow, and therefore cardiac output, is dependent on atrial systole. Furthermore, this flow is generated by only modest pressure transients (1 or 2 mm Hg) between the right atrium (RA) and the PA in diastole. Clearly a low pulmonary vascular resistance is crucial for this source of cardiac output to be maintained. An important element of the total pulmonary resistance, as discussed above, is the mean airway pressure. Indeed, antegrade diastolic flow is often entirely abrogated during positive pressure inspiration. Conversely, negative pressure ventilation may have a major beneficial effect on cardiac output. In postoperative tetralogy patients, positive pressure ventilation reduces cardiac output by >30% compared with that achieved during negative pressure ventilation with a cuirass device (13).
The influence of cardiopulmonary interaction is even more impressive when one considers right heart bypass procedures. Here, resting and exercise pulmonary blood flow is markedly dependent on the work of breathing. In our earlier Doppler studies, the phase relationship between ventilation and pulmonary blood flow was shown clearly in both the venopulmonary and atriopulmonary Fontan circulations (14,15). Subsequently, MRI studies have suggested that well over one-third of the cardiac output occurs as a direct result of the work of breathing (16). This may be even more important during exercise (17), particularly in those with total cavopulmonary anastomosis. Positive pressure ventilation in the Fontan circulation has long been known to adversely affect cardiac output. In early studies of the use of positive end-expiratory pressure, a linear relationship between positive end-expiratory pressure and cardiac output was demonstrated (18). Unsurprisingly, negative pressure ventilation under these circumstances can lead to a marked increase in cardiac output compared with positive pressure ventilation. In a separate series of studies, Shekerdemian et al. (19) showed the profound effects of positive and negative pressure ventilation on the pattern of pulmonary blood flow in the Fontan circuit (Fig. 26.3) as well as on total cardiac output measured by respiratory mass spectrometry.
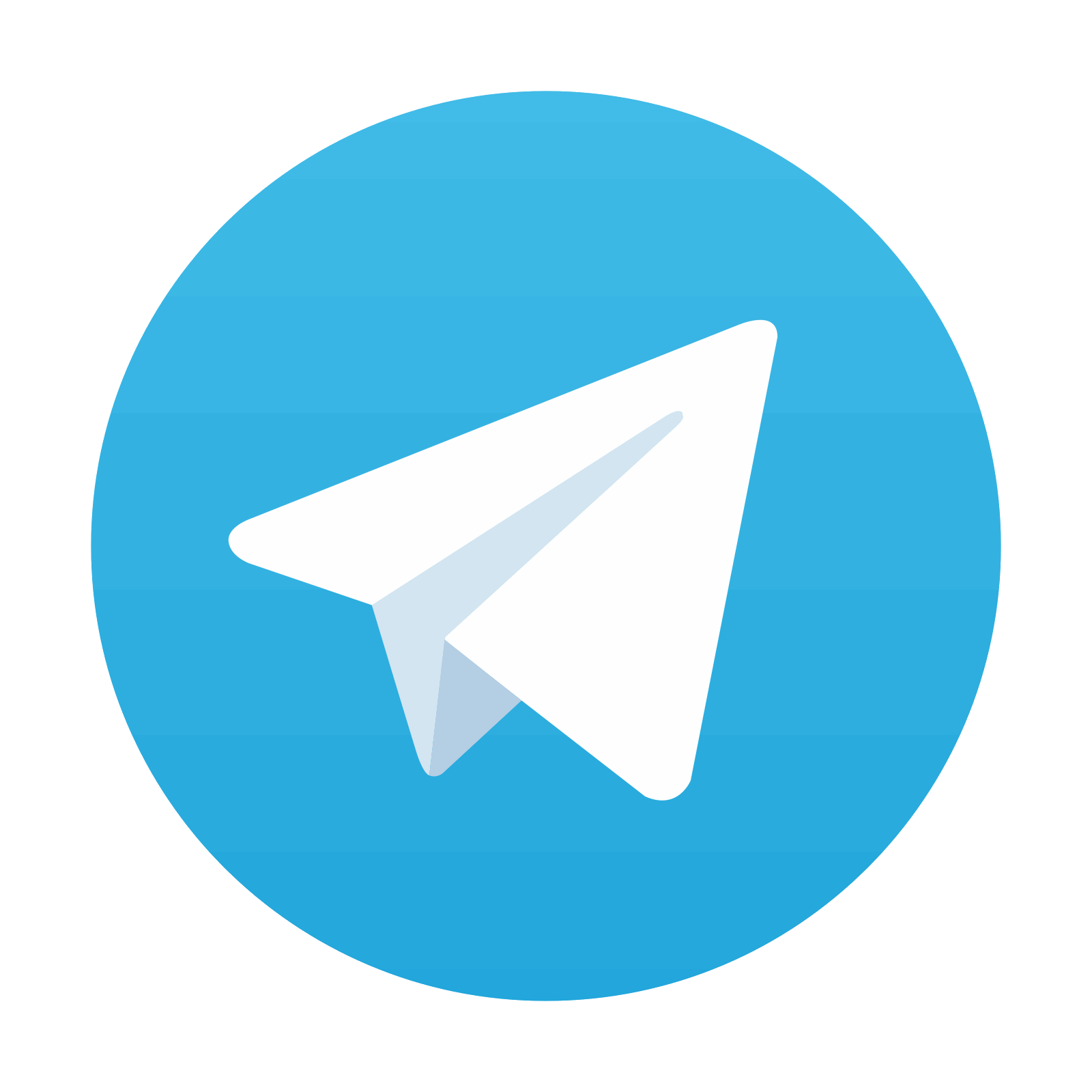
Stay updated, free articles. Join our Telegram channel

Full access? Get Clinical Tree
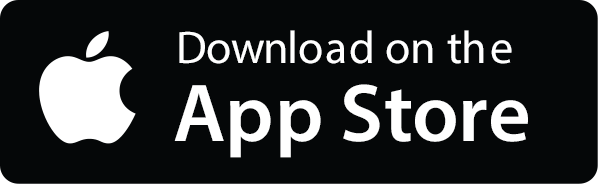
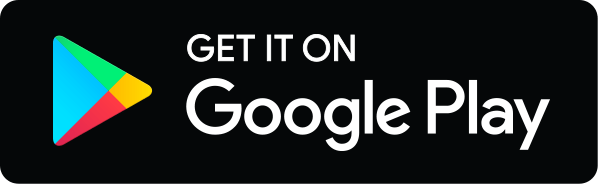