Cardiac Anatomy and Examination of Cardiac Specimens
Joseph J. Maleszewski
William D. Edwards
A fundamental understanding of cardiac anatomy forms the cornerstone of diagnostic pediatric cardiology and is a prerequisite for the proper interpretation of clinical cardiovascular imaging. In this chapter, cardiac anatomy is presented segmentally, with an emphasis on comparisons between analogous right-sided and left-sided structures. Although standard and commonly accepted anatomic terminology is used, anglicized forms are also provided in parentheses—for example, crista terminalis (terminal crest).
Mediastinum
General Features
In keeping with their embryonic origins as midline structures, the heart and great vessels occupy the midthorax, within the mediastinum. The anatomic borders of the mediastinum are as follows:
Anteriorly, the sternum and its adjacent ribs
Posteriorly, the vertebral column and its adjacent ribs
Laterally, the medial aspects of the parietal pleuras (pleurae)
Superiorly, the plane of the first rib
Inferiorly, the diaphragm
The mediastinum, in turn, is divided into four regions (Fig. 6.1). The heart, aortic arch, and descending thoracic aorta are located in the middle, superior, and posterior regions, respectively. Also located within the mediastinum are the esophagus, trachea, right and left main bronchi, thymus, lymph nodes, autonomic nerves, thoracic duct, and small vessels (including bronchial, esophageal, azygos, and hemiazygos).
Cardiac Size
The size of the heart relative to the thoracic cage varies with age. Radiographically, the normal cardiothoracic ratio is 60% or less for newborns and 50% or less in children and adults (Fig. 6.2). However, these ratios are applicable only for full respiratory inspiration, a condition that may be difficult to attain in newborns and infants. Accurate assessment of the great vessels by chest radiography also may be hampered by the overlying thymus.
Cardiac size also is proportional to body size and correlates better with body surface area and weight than with height. In well-conditioned athletes with physiologic cardiac hypertrophy heart weights may approach or slightly exceed the upper limits of normal. Heart weight varies with gender as well and, for the same body size, is greater in girls than in boys during infancy and childhood. By the time a body weight of 25 kg is achieved, however, heart weights are similar between genders, and beyond 35 kg body weight, heart weights in boys exceed those in girls by about 10% (1). This trend continues throughout adult life and increases with body size, from 15% at 70 kg, to 20% at 100 kg, to 25% at 150 kg (2).
In general, the normal human heart is roughly the size of one’s fist. In this regard, it is important to emphasize that a patient’s heart size should be similar in size to the patient’s fist, not the examiner’s. This obvious fact can easily be forgotten when one is viewing cardiac images and not taking into account the size of the patient.
![]() Figure 6.1 Mediastinum, shown schematically. Viewed from a right lateral perspective, the mediastinum has four divisions. |
Cardiac Position
Within the mediastinum, the cardiac apex is normally directed leftward, anteriorly, and inferiorly, and this constitutes levocardia. In newborns, the apical direction is more horizontal than in children or adults.
However, once the heart is removed from the chest, whether literally at autopsy or technically by projecting an image onto a video monitor, the extracardiac reference points are lost, and orientation becomes a matter of convenience. Traditionally, photographs of cardiac specimens have been oriented with the apex down, and echocardiographic four-chamber images of the heart are often projected similarly. As a result, confusion has arisen concerning the true anatomic positions of the cardiac chambers and valves.
Pericardium
General Features
The pericardium both covers the heart as the epicardium and surrounds it as the parietal pericardium, much like a fluid-filled balloon covers a fist that is pressed into it. Between the two layers, within the pericardial sac, serous pericardial fluid (≤25 mL in adults) serves to lubricate the heart and allow its relatively friction-free movement within the chest. In addition, the parietal pericardium limits the diastolic dimensions of the heart.
Parietal Pericardium
The parietal pericardium represents a tough, flask-shaped sac that surrounds the heart and attaches along the great vessels, such that the ascending aorta and main pulmonary artery are intrapericardial (Fig. 6.3A). Similarly, the terminal 2 to 4 cm of the superior vena cava are also located within the pericardial sac, as are shorter lengths of the pulmonary veins and the inferior vena cava.
For patients with total anomalous pulmonary venous connection, the confluence of pulmonary veins is located within the pericardial sac behind the heart. In contrast, the right and left pulmonary arteries and the ductus arteriosus are extrapericardial structures, and surgical procedures restricted to these vessels do not require a pericardial incision.
The parietal pericardium consists of an outer fibrous layer and an inner serous layer of mesothelial cells. The fibrous layer is densely collagenous and is ≤1 mm thick in adults. Its outer surface also normally contains variable amounts of adipose tissue, especially near the diaphragm, that can cause apparent thickening of the pericardium, and contributes to the cardiac silhouette radiographically.
Because the fibrous pericardium contains little elastic tissue, it cannot distend acutely. Consequently, the rapid accumulation of as little as 200 mL of pericardial fluid in adults generally produces hemodynamic features of cardiac tamponade. However, in the setting of chronic enlargement of the heart, as occurs with normal body growth or with cardiac dilation, stretching and growth of the
parietal pericardium do take place to accommodate the increasing cardiac volume. Such growth is capable to accommodating >1 L of chronic pericardial effusion.
parietal pericardium do take place to accommodate the increasing cardiac volume. Such growth is capable to accommodating >1 L of chronic pericardial effusion.
![]() Figure 6.3 Parietal pericardium. A: With the anterior aspect of the parietal pericardium removed, the intrapericardial position of the great vessels is apparent. B: With most of the parietal pericardium excised, the pericardial reflection can be identified (arrows), as can the ligament of Marshall (arrowheads) and the transverse sinus (asterisk) (left lateral view). (See Appendix 6.1 for abbreviations.) |
Visceral Pericardium (Epicardium)
The visceral pericardium, or epicardium, covers the heart and the intrapericardial portions of the great vessels. It consists of a delicate lining of mesothelial cells and the subjacent adipose tissue, coronary vessels, and nerves along the surface of the heart. Adipose tissue tends to accumulate within the atrioventricular (AV), interventricular, and interatrial grooves (sulci) and along the acute margin of the right ventricle and the coronary branches. Prominent tags of fat cover the origins of the coronary arteries between the aorta and the atrial appendages. With increasing age, epicardial fat increases in amount and may infiltrate into the atrial septum, particularly within the limbus of the fossa ovalis.
Because the heart must be compliant enough to enlarge during ventricular diastole and to contract during systole, the normal visceral pericardium has no dense fibrous component. Even so, it does have appreciable mechanical strength, as evidenced by the fact that following coronary interventions complicated by arterial perforation, the overlying epicardium readily withstands coronary blood pressure and thereby deters rupture into the pericardial sac.
Pericardial Reflection
The junction between the parietal and visceral layers occurs along the great vessels and is known as the pericardial reflection. That portion involving the great veins forms the oblique sinus, a cul-de-sac (shaped like an inverted U) along the posterior aspect of the left atrium. Between the great arteries anterosuperiorly and the atrial walls, posteroinferiorly, is a tunnel-shaped structure, the transverse sinus (Fig. 6.3B). Nearby, the ligament of Marshall represents the embryonic remnant of a left superior vena cava.
Intraoperatively, in the setting of pulmonary atresia, if a remnant of the hypoplastic or atretic main pulmonary artery exists, it will be found along the ascending aorta, anterosuperior to the transverse sinus. Conversely, a persistent left superior vena cava will abut the left pulmonary artery posterior to the transverse sinus.
Following operative procedures that require an anterior pericardiotomy, the development of fibrinous pericarditis is the rule and may be accompanied by a friction rub. As healing takes place, fibrin is replaced by fibrovascular granulation tissue, from which oozing of blood may occur as small vessels are eroded by repeated contact between the parietal and visceral layers with each heartbeat. For this reason, the pericardium is generally left open postoperatively so that accumulations of blood or fluid can be drained into one of the pleural cavities and removed through a chest tube.
Within a few days, however, the raw and inflamed surfaces generally begin to adhere to the overlying sternum, effectively closing the pericardium. When this occurs, oozing of blood from the pericardial surfaces can result in the insidious development of postoperative cardiac tamponade. Furthermore, in supine patients, localized accumulations of blood within the oblique sinus can produce isolated left atrial tamponade, which can be readily detected by bedside echocardiography.
Over time, organization of fibrinous exudates often results in the development of diffuse fibrous adhesions between the parietal pericardium and the epicardial surface, although progression to constriction is rare. However, fibrous adhesions may increase the risk of subsequent cardiac operations by obscuring the locations of epicardial coronary arteries or, when dissected free, by causing appreciable intraoperative bleeding while the patient is heparinized.
External Topography
General Features
The AV groove (sulcus) defines the plane of the base of the heart which contains the four major cardiac valves. The anterior and inferior interventricular grooves indicate the plane of the underlying ventricular septum. Externally, the two ventricles are similar in size and the atria are appreciably smaller than the ventricles, even though all four internal chamber volumes are similar. Along the surface of the heart, the right and circumflex coronary arteries travel in the right and left AV grooves, respectively, and the left anterior and posterior descending coronary arteries course along the anterior and inferior interventricular grooves, respectively. Thus, by external inspection alone, surgeons and pathologists can assess the location of the coronary arteries and the presence of hypoplastic or dilated chambers.
Base–Apex Characteristics
The ventricles, being roughly conical, have a base (at the base of the heart) and an apex. The base–apex direction (or axis) for both ventricles is leftward, anterior, and inferior, and the two directions are roughly parallel. However, in crisscross hearts, the ventricular apical directions cross and are often perpendicular.
Because the left base–apex length is normally greater than that of the right, the left ventricular apex generally forms the true apex of the heart. However, the right ventricle may form the cardiac apex when the left ventricle is hypoplastic or when the right ventricle is dilated. Rarely, the interventricular groove is quite deep apically and results in a heart with a bifid apex.
The cardiac apex is normally located along the left midclavicular line at the fourth or fifth intercostal space. Clinically, the point of maximal impulse usually corresponds to the anteroseptal region of the left ventricle rather than to the true cardiac apex.
External Landmarks
The junction between the anterior and inferior free walls of the right ventricle forms a sharp angle known as the acute margin, the basal aspect of which delineates the right shoulder of the heart. Analogously, the rounded lateral wall of the left ventricle forms an ill-defined obtuse margin, and its basal aspect represents the left shoulder of the heart. Thus, coronary vessels supplying this region are known as obtuse marginal branches of the circumflex artery. Along the inferior (diaphragmatic) aspect of the heart, the AV, interventricular, and interatrial grooves form a cross-shaped intersection called the crux cordis (crux of the heart).
Chambers and Great Vessels
To properly interpret the various cardiac imaging modalities, one must understand not only the normal size and shape of the cardiac chambers and great vessels but also their relative positions three-dimensionally (Fig. 6.4). In this regard, only the right atrium is anatomically named correctly. It is truly a right lateral chamber, whereas the left atrium lies in the midline posteriorly and is not a left-sided structure. The right ventricle is a right anterior chamber, and the left ventricle is a left posterior structure. Although not striking, the atria are located slightly superiorly relative to the ventricles. Positionally, the aorta arises posteriorly, inferiorly, and to the right of the main pulmonary artery. In patients with congenitally malformed hearts, the relative sizes and positions of the cardiac chambers and great vessels may vary considerably from normal.
Great Veins
Superior Vena Cava
The internal jugular and subclavian veins merge to form brachiocephalic (or innominate) veins bilaterally (Fig. 6.5). Their junctions
are usually guarded by venous valves (3). The brachiocephalic veins enter the mediastinum at the level of the first rib, posterior to the sternoclavicular joint. The left brachiocephalic or innominate vein is two to three times the length of its right-sided counterpart and lies along the anterosuperior aspect of the aortic arch and its brachiocephalic branches. Each innominate vein receives internal mammary (thoracic) and pericardiophrenic veins, and the left also receives the inferior thyroidal vein.
are usually guarded by venous valves (3). The brachiocephalic veins enter the mediastinum at the level of the first rib, posterior to the sternoclavicular joint. The left brachiocephalic or innominate vein is two to three times the length of its right-sided counterpart and lies along the anterosuperior aspect of the aortic arch and its brachiocephalic branches. Each innominate vein receives internal mammary (thoracic) and pericardiophrenic veins, and the left also receives the inferior thyroidal vein.
![]() Figure 6.4 External cardiac anatomy. The heart and great vessels are shown from the anterior (A), posterior (B), right lateral (C), left lateral (D), superior (E), inferior (F), right anterior oblique (G), and left anterior oblique (H) anatomic perspectives, as indicated for each view. (See Appendix 1.1 for abbreviations.) External cardiac anatomy. The heart and great vessels are shown from the anterior (A), posterior (B), right lateral (C), left lateral (D), superior (E), inferior (F), right anterior oblique (G), and left anterior oblique (H) anatomic perspectives, as indicated for each view. (See Appendix 6.1 for abbreviations.) |
![]() Figure 6.5 Systemic veins, shown schematically. A: The systemic veins include the superior and inferior venae cavae and their tributaries. B: The portal circulation drains the abdominal digestive system and the spleen. (See Appendix 6.1 for abbreviations.) |
Both brachiocephalic veins merge to form the superior vena cava, which lies just anteriorly to the right pulmonary artery and against the posterolateral aspect of the ascending aorta. The azygos vein arches over the right bronchus and empties into the superior vena cava posteriorly. The superior vena cava, as a right lateral structure, contributes to the right superior border of the radiographic frontal cardiac silhouette (Fig. 6.6). Approximately one-third to one-half of its length is intrapericardial as it approaches the right atrium.
The right internal jugular vein, right brachiocephalic vein, and superior vena cava provide a short and relatively straight intravascular route to the right atrium and tricuspid orifice that may be used for obtaining endomyocardial biopsy specimens from the right ventricle. Subclavian veins often are used for the placement of transvenous pacemaker leads, and both the subclavian and internal jugular veins are used for the insertion of pressure-monitoring catheters. Indwelling catheters and pacemaker leads can become coated with shallow thrombus, particularly at sites of contact with vascular walls, which may become sources of embolization or infection.
Inferior Vena Cava
The inferior vena cava receives systemic venous drainage from the legs, retroperitoneal viscera, and the portal circulation (Fig. 6.5). Because the veins from the abdominal digestive system drain through the liver, ingested substances are metabolized before they gain access to the remainder of the body. The suprahepatic portion of the inferior vena cava is only a few centimeters in length and, after traversing the diaphragm, joins the inferior surface of the right atrium.
The ostium of the inferior vena cava is guarded by a diminutive crescent-shaped flap of tissue, the eustachian valve. Although generally small, this valve of the inferior vena cava can become so large that it produces a double-chambered right atrium (cor triatriatum dexter).
Interestingly, the vertebral venous plexus does not directly join the inferior vena cava. Rather, it drains into the intracranial, intercostal, lumbar, and lateral sacral veins, as well as into the portal system via the rectal venous plexus. Accordingly, infections or metastases may spread to the vertebral bodies or central nervous system through this vascular network.
Coronary Sinus
The coronary sinus travels in the left AV groove and receives not only the great cardiac vein but also the posterior, middle, and small cardiac veins. It empties into the right atrium near the atrial septum and the orifice of the inferior vena cava. During electrophysiologic studies in patients with the Wolff–Parkinson–White preexcitation syndrome and left-sided bypass tracts, a multielectrode catheter can be positioned within the coronary sinus and great cardiac vein, adjacent to the mitral valve ring, to localize the aberrant conduction pathways. During cardiac operations, cardioplegic solution may be retrogradely administered into the coronary sinus.
The coronary sinus ostium is guarded by a crescent-shaped valve, the thebesian valve. A commissure exists between the valves of the coronary sinus and the inferior vena cava. From this commissure a small cord, the tendon of Todaro, travels just beneath the endocardium and inserts into the membranous septum. Rarely, an unroofed coronary sinus drains directly into the left atrium, or the coronary sinus ostium is atretic.
The valves of the inferior vena cava and coronary sinus are both derived from the embryonic right venous valve. When either is enlarged and fenestrated, the term Chiari net (or network) may be applied.
Pulmonary Veins
Superior (upper) and inferior (lower) pulmonary veins from each lung join the posterolateral aspects of the left atrium. Owing to the midline nature of the left atrium, the right-sided veins are
similar in length to their left-sided counterparts. As a variation of normal, a middle lobe vein from the right lung may enter the left atrium separately rather than first joining the upper lobe vein. In other cases, the upper and lower pulmonary veins, particularly from the left lung, can merge and join the left atrium as a single vein.
similar in length to their left-sided counterparts. As a variation of normal, a middle lobe vein from the right lung may enter the left atrium separately rather than first joining the upper lobe vein. In other cases, the upper and lower pulmonary veins, particularly from the left lung, can merge and join the left atrium as a single vein.
![]() Figure 6.6 Heart and great vessels. A: The borders of the frontal cardiac silhouette are demonstrated on a chest radiogram. B: An anterior view of a cardiac specimen is shown, for comparison with A. (See Appendix 6.1 for abbreviations.) |
The right and left lower pulmonary veins each travel along the inferior aspect of the corresponding main bronchus. In contrast, the two upper veins each course anteriorly to their respective bronchus and, at the pulmonary hilum, lie anteriorly to the right intermediate and left main pulmonary arteries. Thus, because the upper pulmonary veins travel anteriorly and the pulmonary arteries travel posteriorly (moving from the heart to the hilus), the veins are posterior to the arteries at the level of the left atrium but lie anteriorly to the arteries at the level of the pulmonary hilum.
Interestingly, the media of the pulmonary veins, within 1 to 3 cm of the left atrium, contain myocardial cells rather than smooth muscle cells. Consequently, these regions can function as sphincters during atrial systole thereby minimizing retrograde blood flow back into the lungs. These venous myocytes can also be the nidus for atrial fibrillation. Because the pulmonary veins are normally thin walled and distended under low pressure, they are prone to extrinsic compression either by a native structure, such as thrombus or neoplasm, or by synthetic materials, such as a conduit or surgical hemostatic packing material.
Atria
General Features
The right and left atria serve as receiving chambers for blood returning from the systemic and pulmonary venous systems, respectively. They also have an endocrine function, particularly the right atrium. In the setting of right atrial dilation or congestive heart failure, atrial natriuretic peptide is released from secretory granules within myocytes as part of the cardiorenal system for sodium and body fluid homeostasis.
Right Atrium
The right atrium is a right lateral chamber that, along with the venae cavae, forms the right lateral border of the radiographic frontal cardiac silhouette (Fig. 6.6). It receives blood from the two venae cavae, coronary sinus, and numerous small thebesian veins, and it expels blood across the tricuspid valve and into the right ventricle. Structurally, the right atrium consists of a free wall and septal components.
Free Wall
Internally, the free wall has a smooth posterior region and a more muscular anterior region (Fig. 6.7). The posterior aspect receives the two venae cavae and has a veinlike appearance, in keeping with
its embryologic origin from the sinus venosus. In contrast, the anterior aspect exhibits a muscular wall and a large pyramidal appendage. A prominent C-shaped ridge of muscle, the crista terminalis, serves to separate the two regions and forms one of the tracts for internodal conduction.
its embryologic origin from the sinus venosus. In contrast, the anterior aspect exhibits a muscular wall and a large pyramidal appendage. A prominent C-shaped ridge of muscle, the crista terminalis, serves to separate the two regions and forms one of the tracts for internodal conduction.
![]() Figure 6.7 Comparison of right and left atria. A: Opened right atrium. Two arrow-shaped probes show that the superior vena cava is directed toward the tricuspid orifice and the inferior vena cava is directed toward the fossa ovalis. B, C: Atrial septum. A white probe in the patent foramen ovale passes between the limbus and valve of the fossa ovalis in the right atrium (B) and exits through the ostium secundum in the left atrium (C). D, E: Atrial free walls. The right atrial wall (D, viewed from a left lateral perspective) contains a crista terminalis and pectinate muscles, whereas the left atrial wall (E, viewed from an anterior perspective) contains neither of these structures. F: The interatrial and atrioventricular septa are demonstrated in a four-chamber slice of the heart. (See Appendix 6.1 for abbreviations.) |
Numerous pectinate muscles arise from the terminal crest and travel as parallel ridges along the anterior aspect of the free wall. Pectinatus is Latin for comb, and the crista terminalis and pectinate muscles may be likened to the backbone and teeth of a comb, respectively. An irregular arrangement of pectinate muscles is also found within the atrial appendage and, as a result, atrial pacemaker leads can readily be lodged in this area. The right atrial appendage rests against the ascending aorta and overlies the proximal right coronary artery.
When right atrial enlargement is associated with stasis to blood flow, thrombus may form between the pectinate muscles, particularly within the appendage. Transvenous pacemaker leads and intracardiac catheters often produce linear contact lesions at the cavoatrial junction, and these usually become lined by shallow mural thrombi.
It is important to note that the atrial wall between the ridges of pectinate muscles is generally <1 mm thick and can be perforated by catheters and pacemaker leads. Although the posterior half of the free wall (derived from the sinus venosus) is also only about 1 mm thick, it has a thicker endocardium and is therefore less prone to perforation. In adolescents and adults, the pectinate muscles are 2 to 4 mm thick and the crista terminalis may achieve a thickness of 3 to 6 mm.
Septum
When viewed from the right, the septum has an interatrial component (between the right and left atria) and an AV component (between the right atrium and left ventricle). The interatrial portion is relatively small, and its most prominent feature is the fossa ovalis (4). This consists of a horseshoe-shaped muscular rim—the limbus, which forms a pathway for internodal conduction—and a central sheet of thin fibrous tissue—the valve of the fossa ovalis (Fig. 6.7). In adolescents and adults, the limbus averages 4 to 8 mm in thickness, and the valve is about 1 mm thick. Embryologically, the valve of the fossa ovalis represents the first septum that develops (septum primum), and the limbus represents the second septum that forms (septum secundum).
During fetal and neonatal life, the valve of the fossa ovalis represents a paper-thin, delicate, translucent membrane. As such, it is readily torn (or stretched) during balloon atrial septostomy procedures. With increasing age, however, the progressive deposition of collagen and elastin produces a thicker, tougher, opaque valve (5). As a result, transseptal procedures may be more difficult in older children, adolescents, and adults.
In contrast to the fossa ovalis, the foramen ovale represents a potential passageway between the two atria. It courses between the anterosuperior aspect of the limbus and the valve of the fossa ovalis and then through a natural valvular perforation, the ostium secundum and into the left atrium (Fig. 6.7). Although the foramen ovale is patent throughout fetal life, it functionally closes soon after birth, as left atrial pressure begins to exceed that in the right atrium, and the valve of the fossa ovalis becomes pressed against the limbus, thereby effectively closing the foramen.
In approximately two-thirds of individuals, the foramen ovale closes permanently during the first year of life, as fibrous tissue seals the valve to the limbus of the fossa ovalis. Thus, in about one-third of infants, children, and adolescents, this flap valve is not sealed (patent foramen ovale) and closes only when the pressure in the left atrium exceeds that in the right atrium. During the Valsalva maneuver, for example, a small right-to-left shunt can be detected echocardiographically in persons with a patent foramen ovale. In adolescents and adults, the foramen ovale ranges from 2 to 10 mm in maximal potential diameter, with a mean size of 5 to 6 mm (6).
In the setting of pronounced atrial dilation, the atrial septum can be stretched to such an extent that the limbus no longer covers the ostium secundum, resulting in a valvular-incompetent patent foramen ovale—an acquired atrial septal defect. In contrast, fenestrations of the valve are the most common cause of congenital atrial septal defects. Excessive valve tissue may undulate during the cardiac cycle and form an aneurysm of the fossa ovalis.
Because the tricuspid valve annulus attaches to the septum lower (more apically) than the mitral annulus, septal myocardium is interposed between the right atrium and the left ventricle. This constitutes the AV septum (Fig. 6.7). Although this is primarily a muscular septum, averaging 10 mm thick in adults, it also contains a membranous portion that is only about 1 mm thick. The AV portion of the membranous septum is located at the anteroseptal tricuspid commissure (when viewed from the right side of the heart) and beneath the right posterior aortic commissure (as seen from the left side).
The AV septum corresponds to the triangle of Koch, an important anatomic surgical landmark because it contains the AV node and the proximal (penetrating) portion of the AV (His) bundle. Thus, during tricuspid annuloplasty procedures, care must be taken to avoid injury to the conduction system. When defects occur in the muscular AV septum, the mitral annulus usually drops to the same level as the tricuspid annulus, so that the defect becomes primarily interatrial, and the AV conduction tissues are displaced inferiorly.
Finally, a medial portion of the free wall lies against the right aortic sinus which bulges somewhat into the atrial cavity as the torus aorticus (aortic bulge). This protuberance is bordered by the limbus of the fossa ovalis, the ostium of the appendage, the tricuspid annulus, and the AV septum. During transseptal procedures, care must be taken to stay within the confines of the valve of the fossa ovalis to avoid perforation along the aortic protuberance which could result in trauma to the adjacent aortic root or coronary arteries.
Because of hemodynamic streaming within the right atrium during intrauterine life, poorly oxygenated blood from the superior vena cava is directed toward the tricuspid orifice, whereas well-oxygenated placental blood within the inferior vena cava is directed by the eustachian valve toward the foramen ovale and into the left atrium. Consequently, the most oxygenated blood in the fetal circulation travels, via the left side of the heart, to the coronary arteries, upper extremities, and the rapidly developing central nervous system. Throughout postnatal life, this orientation of the venae cavae is maintained (Fig. 6.7). As a result, transseptal procedures are more easily performed via the inferior vena cava, in contrast to right ventricular biopsies, which are more readily performed by a superior vena caval approach.
Left Atrium
The left atrium is a midline posterior chamber that receives pulmonary venous blood and expels it across the mitral valve and into the left ventricle. By virtue of its posterior location, the body of the left atrium does not contribute to the borders of the radiographic frontal cardiac silhouette. However, the left atrial appendage, when enlarged, may produce a bulge along the left cardiac border, between the left ventricle and the left pulmonary artery.
Interposed between the left atrium and the vertebral bodies are the esophagus, to the right, and the descending thoracic aorta, to the left. Furthermore, the bifurcated pulmonary artery and left bronchus travel along the superior aspect of the left atrium, and the left and posterior aortic sinuses may indent the atrial wall as the aortic protuberance (torus aorticus). During transesophageal echocardiography, the transducer is placed close to the left atrium and provides excellent visualization of the atria, AV valves, and great vessels.
In the setting of left atrial dilation, the left bronchus is pushed upward, as can be seen radiographically, and the esophagus is displaced rightward. When a left superior vena cava persists, the coronary sinus into which it drains is generally quite dilated, in some cases indenting the left atrial wall, and should not be mistaken echocardiographically for the descending thoracic aorta. As on the right side, the left atrium consists of a free wall and a septum.
Free Wall
The free wall includes a dome-shaped body which receives the pulmonary veins and a fingerlike appendage. These two regions are separated externally by the left atrial coronary vein and ligament of Marshall and internally by the ostium of the appendage. The left atrial body, although 1 to 3 mm thick and infiltrated by cardiac myocytes, is derived embryologically from the common pulmonary vein and internally maintains a smooth veinlike appearance. The endocardium is opaque and gray-white, owing to the deposition of collagen and elastin, and is thicker and less compliant than that in the other three chambers.
The left atrial appendage rests along the left AV groove and covers the proximal circumflex coronary artery and, in some individuals, the left main coronary artery. The appendage contains numerous small pectinate muscles, has a variable number of lobes or blind-ended pouches, is tortuous, and may fold on itself. The morphology of the left atrial appendage is variable, but four basic types have been described: Windsock, cactus, cauliflower, and chicken wing. Outside the appendage, the body of the left atrium contains no pectinate muscles, and there is no crista terminalis.
As the left upper pulmonary vein joins the left atrium, an infolded ridge often forms where the ostium of the pulmonary vein is contiguous with that of the atrial appendage. This should not be mistaken as a partial form of cor triatriatum (triatrial heart).
Septum
When viewed from the left, the septum is entirely interatrial. Along its anterosuperior border, the valve of the fossa ovalis contains one or more fenestrations, representing the embryologic counterpart of the ostium secundum. If a probe passed through the fenestrations enters the right atrium, the foramen ovale is considered patent. Neither the limbus of the fossa ovalis nor the AV septum is visible from within the left atrium. Several small thebesian veins drain directly into the left atrial cavity, particularly along the septum.
Comparison of the Atria
With regard to the atrial septum, the limbus of the fossa ovalis is a feature of the right atrium, and the ostium secundum is characteristic of the left atrium (Fig. 6.7). The free wall of the right atrium contains the crista terminalis and pectinate muscles, whereas that of the left atrium does not (Table 6.1). Moreover, the right atrial
appendage is large and pyramidal, whereas the left atrial appendage is smaller and fingerlike. Although the superior vena cava and the pulmonary veins can anomalously join the contralateral atrium, the inferior vena cava almost invariably joins the morphologic right atrium.
appendage is large and pyramidal, whereas the left atrial appendage is smaller and fingerlike. Although the superior vena cava and the pulmonary veins can anomalously join the contralateral atrium, the inferior vena cava almost invariably joins the morphologic right atrium.
TABLE 6.1 Comparison of Right-Sided and Left-Sided Anatomic Fractures of Cardiac Segments | ||||||||||||||||||||||||||||||||||||||||||||||||||
---|---|---|---|---|---|---|---|---|---|---|---|---|---|---|---|---|---|---|---|---|---|---|---|---|---|---|---|---|---|---|---|---|---|---|---|---|---|---|---|---|---|---|---|---|---|---|---|---|---|---|
|
Thus, the distinguishing features of a morphologic right atrium are the limbus of the fossa ovalis, connection of the inferior vena cava, and a large pyramidal appendage. The limbus can be detected with four-chamber imaging, and the course of the inferior vena cava and the morphology of the atrial appendage can be assessed by either invasive or noninvasive imaging. Identification of the crista terminalis and pectinate muscles is possible by direct inspection at operation or autopsy but not consistently by imaging procedures.
Atrioventricular Valves
General Features
The AV valves serve to maintain unidirectional blood flow and to electrically separate the atria and ventricles. Each valve has five components. The annulus, leaflets, and commissures form the valvular apparatus, and the chordae tendineae (tendinous cords) and papillary muscles form the tensor apparatus.
The annulus of each valve is somewhat saddle shaped rather than being truly planar and represents an ill-defined ring of fibrous tissue from which the leaflets arise. Although the mitral annulus is a continuous ring of collagen, the tricuspid annulus is not and exhibits loose connective tissue at the points of annular discontinuity. Consequently, ventricular dilation leads more readily to annular dilation of the tricuspid valve than of the mitral valve. During the first two decades of life, valvular growth correlates better with age than with body height, weight, or surface area (1).
Leaflets represent delicate flaps of connective tissue. Owing to direct cordal insertions along their leading edges, the free edges have a serrated appearance. Tendinous cords also insert along the ventricular aspect of each leaflet (the valve pocket or undersurface) and thereby support the leaflet during ventricular systole. On the atrial aspect, the closing edge represents an ill-defined junction between the thinner body (or clear zone) and the thicker contact region (or rough zone) of the leaflet. During valve closure, apposing leaflets contact one another along the surfaces between the free and closing edges (Fig. 6.8). In about 50% of fetuses and infants, blood cysts occur as small (<3 mm) purple nodules along the contact
surfaces of the mitral and tricuspid valves and generally disappear within 1 year (7).
surfaces of the mitral and tricuspid valves and generally disappear within 1 year (7).
![]() Figure 6.8 Comparison of right and left atrioventricular valves. A: The tricuspid valve normally has three leaflets. The membranous septum is located along its annulus (dashed line), at the anteroseptal commissure (arrow). B: The mitral valve has two leaflets, with papillary muscles beneath each commissure. C, D: In short-axis views, the tricuspid orifice is shaped like a reversed D at the annular level (C) and like a triangle at the midleaflet level (D). In contrast, the mitral orifice is elliptical at both levels. As shown in D, the anterior leaflet (arrow) of each valve is a midcavitary structure that divides its ventricle into inflow and outflow regions. (See Appendix 6.1 for abbreviations.) |
Microscopically, each leaflet exhibits two major layers. A fibrous layer (fibrosa) forms the strong structural backbone of the valve and is continuous from the annulus proximally to the sites of cordal insertion distally. In contrast, the spongy layer (spongiosa) acts as a shock absorber and becomes prominent along the contact regions of each leaflet. Because the leaflets are thin and compliant and are attached only at the annulus and papillary muscles, rapid opening and closure of the valve is possible.
A commissure represents the site along a valve annulus where two leaflets meet. Commissures always have an underlying papillary muscle and a fanlike array of tendinous cords that attach to both leaflets, in contrast to congenital clefts, which have neither. Although each leaflet has two major commissures, it also may be further divided into several regions, or scallops, by minor commissures, each of which has a small underlying papillary muscle.
The tendinous cords act as strong fibrous guy-wires that anchor and support the leaflets. Accordingly, they restrict excessive valvular excursion during ventricular systole and prevent valvular prolapse into the atrium. Because a cord generally branches several times, more than 150 cords normally insert into the free edge or ventricular aspect of each valve, which tend to distribute the systolic force of ventricular blood evenly along the undersurface of each leaflet (8,9). If the tendinous cords are malformed, weakened, or insufficient in number, a portion of the leaflet can begin to bulge and prolapse, leading to valvular regurgitation. This commonly occurs not only with myxomatous valves, but with common AV valves and at least one of the two AV valves in a double-inlet left ventricle (10).
Papillary muscles can be single, multiheaded, or a fused group. By being positioned directly beneath a commissure and by receiving tendinous cords from two adjacent leaflets, a papillary muscle tends to pull its two leaflets toward each other during ventricular systole, thereby facilitating valve closure. Ventricular contraction also contributes to valve closure by decreasing the annular dimension and shortening the base–apex length of the chamber. Thus, papillary muscle ischemia or ventricular dilation may produce valvular regurgitation, as can be seen with the tricuspid valve after severe birth asphyxia or in the setting of persistent pulmonary hypertension of the newborn.
Tricuspid Valve
Because of the normal rightward bowing of the ventricular septum, the tricuspid annulus is shaped like a reversed D when viewed from its ventricular aspect (Fig. 6.8). However, at midleaflet level, the orifice becomes triangular. Annular dimensions vary with the cardiac cycle, decreasing by about 20% in circumference and 33% in area during systole, owing to contraction of the right ventricular myocardium (11). The plane of the tricuspid annulus faces toward the right ventricular apex.
While the septal and posterior leaflets lie against the ventricular septum and inferior wall of the right ventricle, respectively, the anterior leaflet forms a prominent intracavitary curtain that partially separates the inflow and outflow tracts. The anterior leaflet is the most mobile of the three, and the septal leaflet, by virtue of its numerous direct cordal attachments along the ventricular septum, is the least mobile. The relative sizes of the three leaflets vary appreciably from person to person (9).
Among the three papillary muscles, the anterior muscle is the largest and most well formed. It originates from the acute margin of the right ventricle, may be single or bifid, and provides cordal insertions to the anterior and posterior leaflets. The posterior papillary muscle arises from the inferior wall near the septum, and the posterior and septal leaflets receive cords not only from this small muscle but also from accessory papillary muscles and trabeculations. The medial papillary muscle (also called the papillary muscle of the conus or the muscle of Lancisi) emanates along the superior aspect of the septal band at the level of the membranous septum and has cordal attachments to the septal and anterior leaflets. Although prominent in infants and children, it commonly merges with the septal band and becomes small or absent by adulthood.
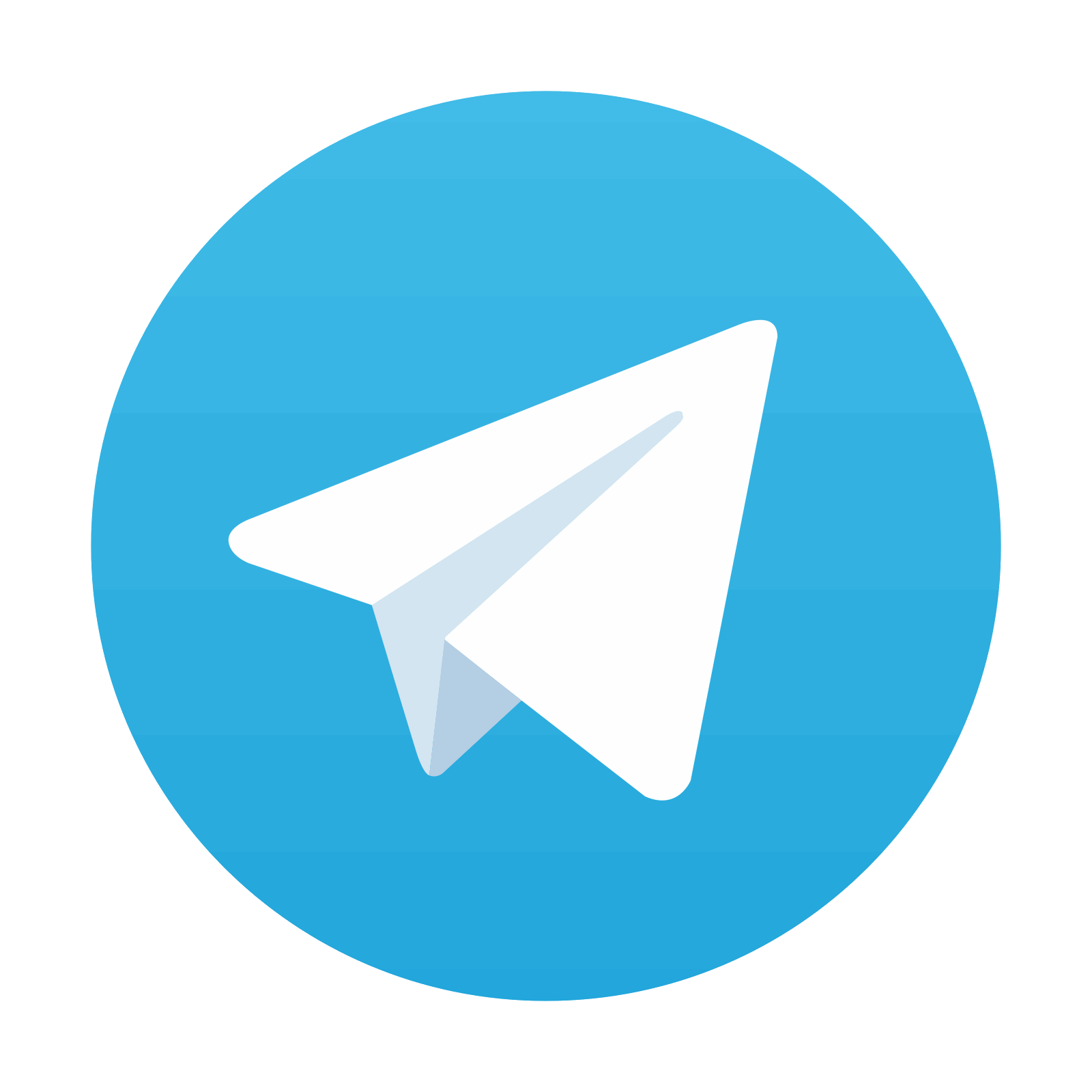
Stay updated, free articles. Join our Telegram channel

Full access? Get Clinical Tree
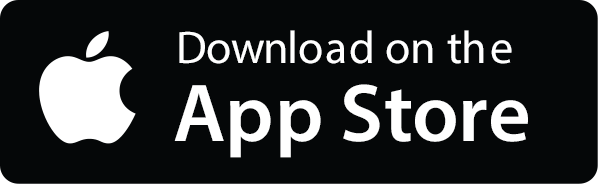
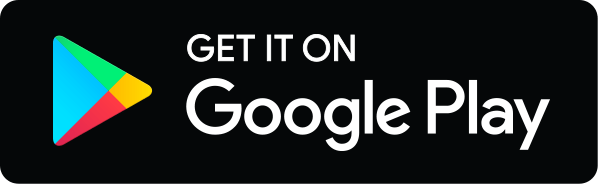