Abstract
Improved survival of very immature infants has contributed to an increase in the number of those who develop bronchopulmonary dysplasia (BPD). BPD is a chronic lung disease that occurs in roughly 10,000–15,000 infants per year in the United States alone. This has important implications for the utilization of health resources, as follow-up studies have demonstrated that BPD infants require frequent readmission to the hospital in the first 2 years after birth for respiratory infections, asthma, and related problems; they also have persistent lung function abnormalities as adolescents and young adults. BPD most commonly occurs in prematurely born infants who have required mechanical ventilation and oxygen therapy for acute respiratory distress. This chapter reviews our current understanding of the etiology, pathophysiology, and treatment of BPD.
Keywords
prematurity, pulmonary hypertension, mechanical ventilation, chronic lung disease
Introduction
Improved survival of very immature infants has contributed to an increase in the number of infants who develop bronchopulmonary dysplasia (BPD). BPD is a chronic lung disease that occurs in roughly 10,000–15,000 infants per year in the United States alone. This has important implications for the utilization of health resources, as follow-up studies have demonstrated that BPD infants require frequent readmission to the hospital in the first 2 years after birth for respiratory infections, asthma, and related problems; they also have persistent lung function abnormalities as adolescents and young adults. BPD most commonly occurs in prematurely born infants who have required mechanical ventilation (MV) and oxygen therapy for acute respiratory distress, but it also can occur in immature infants who have had minimal initial lung disease. Although BPD is most commonly associated with premature birth, it can occur in term or near-term infants due to severe acute lung injury, as reflected by a need for high mechanical ventilator support or extracorporeal membrane oxygenation (ECMO) therapy.
Over the past 40 years, the introduction of prenatal steroid use, surfactant therapy, new ventilator strategies, aggressive use of early continuous positive airway pressure (CPAP), changes in approach to managing patent ductus arteriosus (PDA), improved nutrition and other treatments have resulted in dramatic changes in the clinical course and outcomes of premature newborns with respiratory distress syndrome (RDS). Whereas the overall incidence of BPD has not declined over the past decade, its severity has been clearly modulated by changes in clinical practice. There is now growing recognition that infants with chronic lung disease after premature birth have a different clinical course and pathology than had been traditionally observed in infants who were dying with BPD during the presurfactant era ( Fig. 20.1 ). The classic progressive stages with prominent fibroproliferation that first characterized BPD are often absent, and the disease has changed to being predominantly defined by a disruption of distal lung growth; this has been termed “the new BPD.” In contrast to classic BPD, the new BPD develops in preterm newborns who may have required minimal or even no ventilatory support and relatively low inspired oxygen concentrations during the early postnatal days ( Fig. 20.2 ). At autopsy, the lung histology of infants who die with the new BPD displays more uniform and milder regions of injury, but impaired alveolar and vascular growth remain prominent ( Table 20.1 ). The implications of how these changes in BPD alter long-term pulmonary outcomes remain unknown. The new BPD is likely the result of disrupted antenatal and postnatal lung growth that leads to persistent abnormalities of lung architecture. It is unclear whether such infants subsequently experience sufficient catch-up lung growth to achieve and sustain improved lung function over time. To date, there are limited safe and effective preventative therapies for BPD, but there are promising new therapies directed either at reducing lung injury or improving lung growth.



Overall, BPD may perhaps be best considered as a “syndrome” rather than a single disease because etiologies, clinical course and respiratory outcomes are diverse and modulated by therapeutic interventions. The changing nature of BPD suggests that a standard binomial definition will not adequately predict long-term pulmonary outcomes. Importantly, there is growing recognition that prematurity itself, even in the absence of BPD and even in late preterm infants, is associated with significant late respiratory morbidity. Additionally, there is a growing appreciation that interventions designed to prevent BPD should focus on late pulmonary and neurodevelopmental outcomes that have the most impact on the health and welfare of prematurely born children and their families rather than short-term outcomes (e.g., supplemental oxygen requirement at 36 weeks corrected age). Such a change in thinking about BPD requires the development of multidisciplinary teams of health care professionals and clinician-scientists, an appreciation of the magnitude, nature and chronic manifestations of prematurity and BPD and novel programs that provide continuity of long-term care. This chapter reviews the epidemiology, pathophysiology, and long-term outcomes of infants with BPD.
Definition
Despite extensive studies of premature infants with chronic lung disease, the definition of BPD remains problematic. Northway’s initial diagnosis incorporated a clinical assessment, radiologic findings, and histopathology. Today current definitions of BPD diagnose the disease based on the treatment: oxygen requirement at 28 days or 36 weeks corrected gestational age. Ideally a diagnosis of BPD would act as an accurate surrogate to predict long-term pulmonary morbidity, would be a meaningful outcome measure for evaluating prevention and therapeutic interventions and would be consistently defined between units so it could be used to compare performance and identify best practices, identifying quality improvement measures and targeting future investigation. Unfortunately the current definition falls short on all counts. Owing to inconsistencies in how different institutes were defining BPD, a National Institutes of Health–sponsored conference developed a new definition of BPD that incorporates several components of previous definitions and adds a BPD severity categorization according to the level of respiratory support required at 36 weeks corrected gestational age or at the time of discgharge ( Table 20.2 ). The potential advantage of this approach is that BPD is defined as a spectrum of disease with early markers that may be predictive of long-term pulmonary morbidity. However, even with the improved current definition, sensitivity and specificity are lacking in predicting long-term pulmonary adverse outcomes. In one study evaluating pulmonary outcomes with different definitions of BPD, even with the improved definition, 20%–27% of very low birth weight (VLBW) neonates without a diagnosis of BPD still had long-term pulmonary morbidity requiring respiratory medications or rehospitalization for respiratory etiology. For the VLWB neonates with a diagnosis of severe BPD, up to 50% did not have any long-term pulmonary morbidities ( Fig. 20.3 ).
Gestational Age | <32 Weeks | >32 Weeks |
---|---|---|
Time point of assessment | 36 weeks PMA or discharge, whichever comes first | >28 days but <56 days postnatal age or discharge, whichever comes first |
Treatment with oxygen >21% for at least 28 days | ||
Mild BPD | Breathing room air at 36 weeks PMA or discharge, whichever comes first | Breathing room air by 56 days postnatal or discharge, whichever comes first |
Moderate BPD | Need for <30% O 2 at 36 weeks PMA or discharge, whichever comes first | Need for <30% O 2 to 56 days postnatal age or discharge, whichever comes first |
Severe BPD | Need for >30% O 2 ± PPV or CPAP at 36 weeks PMA or discharge, whichever comes first | Need for >30% O 2 ± PPV or CPAP at 56 days postnatal age or discharge, whichever comes first |

An additional problem in defining BPD is the wide center-to-center variability in diagnosing the need for supplemental oxygen. A survey from the Vermont Oxford Network revealed striking variations in thresholds for instituting supplementary oxygen based on pulse oximetry, ranging from less than 84% to less than 96%, with only 41% of the respondents using the same criterion (<90%). This alone has a marked impact on the reported incidence of BPD. For a given study population, the incidence of BPD decreased from 37% to 24% if the need for supplemental oxygen was defined by accepting oxygen saturations above 92% or 88% while breathing room air, respectively. Use of an oxygen reduction test may better help to diagnose ongoing supplemental oxygen requirements. Despite efforts to improve the diagnosis of BPD, the emerging practice trend of using of noninvasive positive pressure through heated high flow leaves up to 2.1% of infants unclassifiable with the current National Institute of Child Health and Human Development (NICHD) definition. Further work is needed to identify early physiologic, structural, and genetic or biochemical markers of BPD that are predictive of critical long-term endpoints, such as the presence of late respiratory disease evidenced by recurrent hospitalizations, reactive airways disease, the need for prolonged oxygen, the need for respiratory medications or exercise intolerance during childhood.
Epidemiology
Pulmonary immaturity is the primary risk factor for BPD owing to incomplete structural and biochemical development, including inadequate surfactant, antioxidant, and antiprotease activities. In the early 1960s, oxygen and MV were selectively used for premature infants with acute respiratory failure due to apnea and hyaline membrane disease. As these therapies were applied more widely, there was a growing recognition of premature infants who survived but developed chronic pulmonary disease with hypoxemia and chest radiographic abnormalities. In 1964, Shepard and colleagues reported that 50% of premature neonates who received oxygen and MV developed chronic lung disease. In 1967, Northway and coworkers provided a comprehensive characterization of the clinical, radiologic and pathologic features of chronic lung disease in infants who had received high concentrations of oxygen and MV from birth. On average, these premature infants were born at 34 weeks’ gestation and weighed 2200 g, yet their mortality was 67%, and the surviving infants had persistent respiratory distress and abnormal chest radiographs beyond the first 4 weeks after birth. In the latter study, the term bronchopulmonary dysplasia was first applied, referring to the striking disruption of airway structure. This seminal study identified the interactive roles of three key pathogenic factors: lung immaturity, acute lung injury, and inadequate repair of the initial lung injury. This basic concept still provides an important paradigm for our current understanding of BPD.
Currently most infants who develop BPD are born with extreme prematurity, and 97% have birth weights below 1250 g. The risk of BPD increases with decreasing gestational age, with an incidence of 85% for infants born at 22 weeks and 23% for those born at 28 weeks ( Fig. 20.4 ). It is also inversely associated with birth weight. The incidence has been reported to be as high as 85% in neonates between 500 and 699 g, but only 5% in infants with birth weights over 1500 g. Although the overall incidence of BPD is reported at about 20% of ventilated newborns, marked variability exists between centers. This likely reflects regional differences in the clinical definitions of BPD, the number of infants born at the hospital versus born at outside facilities and transferred in, the proportion of newborns with extreme prematurity, and specific patient management. In the most immature infants, even minimal exposure to oxygen and MV may be sufficient to contribute to BPD. Two-thirds of infants who develop BPD have only mild respiratory distress at birth ( Fig. 20.5 ). Other observers have recognized this change in patterns of respiratory diseases and have emphasized the importance of enhancing our understanding of the mechanisms of progressive respiratory deterioration and the lack of clinical improvement during the first week of life.


Despite advances in neonatal care, the incidence of BPD has not decreased; some reports suggest that it is actually increasing. Whether this increase in incidence is a result of improved survival of the most immature and vulnerable infants is unclear. Whatever the case may be, it is a strong impetus to identify improved strategies for prevention and treatment.
Etiology
Implications regarding the impact of extremely premature birth on lung growth and structure are best appreciated in the context of the stages of lung development ( Fig. 20.6 ). The fetal lung at 24 weeks’ gestation is generally at the late canalicular or early saccular stage of growth, which is characterized by immature airway structure, undifferentiated epithelial cells, a paucity of capillaries, and reduced surface area. Thus premature birth and injury at this early stage of development have profound implications for the risk of BPD, which provides additional challenges beyond those of premature birth at later stages of lung development.

Epidemiologic studies of BPD have identified many factors that modify the risk. Endogenous factors linked with BPD include gestational immaturity, lower birth weight, male sex, white or nonblack race, family history of asthma, and being small for gestational age. Epidemiologic studies of BPD have yielded a number of predictive models. Although none is routinely applied in caring for infants at risk of BPD, such models might serve as valuable tools for providing risk adjustment for clinical trials and other clinical research studies.
Genetic Susceptibility
The risk for developing BPD is now recognized as being markedly influenced by complex interactions between genetic and environmental risk factors. Variability in the incidence and severity of BPD among premature infants with similar environmental risk factors suggests that genetic susceptibility plays a critical role in the pathogenesis of BPD. Parker and colleagues first reported that genetic factors increase the risk for BPD in preterm twin pairs independent of birth weight, gestational age, gender, RDS severity, PDA, infection, antenatal steroids and other factors. Lavoie and colleagues found that genetic effects accounted for about 80% of the observed variance in BPD susceptibility. Initial genomewide association studies implicated SPOCK2 (SPARC/osteonectin, cwcv and kazal-like domains proteoglycan 2 gene) as significant in the development of BPD ; however, two subsequent genome wide association studies (GWAS) did not confirm this or find other genes to be significant. Exome sequencing from newborn blood spot samples has identified numerous mutated genes in infants with BPD and could provide further insight into genetic risk moving forward. Numerous genes are required for normal lung growth and development, and they are likely to contain sequence variations that modulate the risk for BPD. Published studies have identified several potential candidate genes, especially regarding surfactant proteins and cytokines. However, many studies report small sample sizes, and the findings from most of the studies have not been replicated in subsequent cohorts. The current challenge is to specifically identify the candidate genes that contribute to the development of BPD and their interaction with the specific environmental stimuli that adversely affect lung injury, repair, and structure after preterm birth.
Prenatal Factors
Recent studies have strongly implicated prenatal factors, including maternal smoking, preeclampsia, placental abnormalities, chorioamnionitis, and intrauterine growth restriction as key factors in the risk for BPD after preterm birth. The interactions of prenatal and postnatal factors, such exposure to hyperoxia in growth-restricted infants or after exposure to endotoxin, may be critical factors as well. The question of how these epidemiologic observations are mechanistically linked with premature birth and poor respiratory outcomes is currently under intense laboratory and clinical investigation. Additional perinatal risk factors for BPD include absence of maternal glucocorticoid treatment and perinatal asphyxia.
Postnatal Factors
BPD risk has also been linked with early neonatal influences, including lower Apgar scores, RDS, PDA, higher weight-adjusted fluid intake, earlier use of parenteral lipid, light-exposed parenteral nutrition, and duration of oxygen therapy. Among at-risk infants, the duration and approach to MV (including the use of high inspired oxygen, high peak inspiratory pressure, lower positive end-expiratory pressure, and higher ventilation rate) are also associated with BPD—relationships that could be causal or simply reflect the underlying severity of acute respiratory disease. Increased BPD risk has been associated with hypocarbia (indicated by highest PCO 2 <40 at 48 or 96 hours), suggesting that aggressive ventilation can contribute to lung injury and BPD. Colonization or infection with Ureaplasma urealyticum, postnatal cytomegalovirus infection and postnatal sepsis also appear to predispose to BPD.
Pathogenesis
Preterm infants are especially susceptible to injury caused by mechanical forces, oxidative stress, and inflammation due to the extreme structural and biochemical immaturity of the preterm lung. As Northway first observed, BPD has diverse, multifactorial etiologies, including hyperoxia, ventilator-induced lung injury (VILI), inflammation, and infection ( Fig. 20.7 ). Animal studies suggest that lung injury due to each of these adverse stimuli is at least partly mediated through increased oxidative stress, which further augments inflammation, promotes lung injury, and impairs growth factor–signaling pathways.

Oxygen Toxicity
Oxygen toxicity due to high levels of supplemental oxygen markedly increases the production of reactive oxygen species (ROS), which overwhelm host antioxidant defense mechanisms in the immature lung and thus cause adverse molecular, biochemical, histologic, and anatomic effects. Prematurely born infants are especially vulnerable to oxidative stress because their lungs are relatively deficient in antioxidant enzyme systems (e.g., superoxide dismutase, catalase and others) at birth. Early animal studies clearly demonstrated that high levels of supplemental oxygen promote lung inflammation, impair alveolar and vascular growth, and increase lung fibroproliferation. Experimentally, even relatively mild levels of hyperoxia may be sufficient to induce oxidative stress and impair growth of the immature lung.
A potential method for preventing the development of BPD appears to be prophylactic supplementation of human recombinant antioxidant enzymes. In a multicenter randomized controlled trial (RCT), intratracheal treatment of premature infants at birth with recombinant human CuZn superoxide dismutase (rhSOD) was associated with fewer episodes of respiratory illness (i.e., wheezing, asthma, pulmonary infections) and less need for treatment with bronchodilators or corticosteroids at 1 year corrected age. This suggests that rhSOD may prevent long-term pulmonary injury from ROS in high-risk premature infants. This trial failed to demonstrate a decrease in the diagnosis of BPD, highlighting the challenges posed by the current way in which BPD is defined. Further studies evaluating recombinant antioxidants enzymes have not yet been done.
Ventilator-Induced Lung Injury
MV can induce injury through “volutrauma,” in which phasic stretch or overdistention of the lung can induce lung inflammation, permeability edema, and subsequent structural changes that mimic human BPD, even in the absence of high levels of supplemental oxygen. Aggressive MV with hypocarbia has been associated with the development of BPD, as reports have shown an inverse relationship between low PaCO 2 levels and BPD development. High tidal volumes should be avoided during MV and even during resuscitation in the delivery room. In an experimental study demonstrating a relationship between the size of manual inflations and lung damage in lambs, adverse effects were demonstrated even with inflations of 8 mL/kg. Although small tidal volumes may reduce the risk for VILI in preterm infants, failure to recruit and maintain adequate functional residual capacity (FRC), even with low tidal volumes, is injurious in experimental models.
Inflammation
Experimental and clinical studies have shown that inflammation clearly plays a central role in the pathobiology of BPD. Oxygen toxicity, volutrauma, and infection can induce early and sustained inflammatory responses that promote the recruitment and activation of neutrophils, which persist in infants who develop BPD. Levels of multiple proinflammatory cytokines (e.g., interleukins [ILs] such as IL-1β, IL-6 and soluble intracellular adhesion molecule 1 [ICAM-1]) and growth factors (e.g., transforming growth factor-β [TGR-β], vascular endothelial growth factor, and others) are present and altered in lung lavage and blood samples from premature infants who subsequently develop BPD versus controls. IL-1β induces the release of inflammatory mediators, activating inflammatory cells and upregulating adhesion molecules on endothelial cells. ICAM-1 is a glycoprotein that promotes cell-to-cell contact. Direct contact between activated cells leads to further production of proinflammatory cytokines, such as IL-8, which induces neutrophil chemotaxis, inhibits surfactant synthesis and stimulates elastase release. The levels of collagenase and phospholipase A2 are also increased, and oxidative modification results in the inactivation of alpha-1-antiprotease, which further tips the protease-antiprotease imbalance to favor injury. Lung inflammation is associated with loss of endothelial basement membrane and interstitial sulfated glycosaminoglycans, which are important in inhibiting fibrosis. Tumor necrosis factor alpha (TNF-α) and IL-6 induce fibroblast and collagen production. Increased TGF-β and impaired vascular endothelial growth factor (VEGF) signaling have been strongly linked with the risk for BPD. Leukotrienes are present at high levels in the lungs of infants developing BPD and remain elevated even at 6 months of age.
Evidence that a systemic inflammatory response contributes to the pathogenesis of BPD led investigators to explore the contributions of antenatal, perinatal, and postnatal inflammation to lung injury. Observational studies have suggested an association between amniotic fluid markers and placental and umbilical cord pathology of chorioamnionitis with BPD. The combined effects of antenatal infection or inflammation and clinical factors might contribute to the occurrence of BPD via a number of mechanisms: direct injury of pulmonary parenchyma, disruption of the developmental milieu, impaired angiogenesis, or activation (priming) of immune cells in the lung, thus provoking an exaggerated inflammatory injury in response to a variety of prenatal, perinatal, and postnatal insults. BPD may be increased in infants whose mothers had chorioamnionitis. This is because intraamniotic endotoxin exposure can disrupt alveolar and vascular development and thus lead to a decreased alveolar number and pulmonary hypertension (PH). In a case-control study of 386 infants born at or below 1500 g (after adjusting for other BPD risk factors), chorioamnionitis alone appeared to be associated with reduced risk of BPD; however, chorioamnionitis followed by 7 days of MV or postnatal sepsis had a synergistic effect that substantially increased the risk of BPD. These data suggest that chorioamnionitis might make the lung more susceptible to postnatal injury from a “second hit” of hyperoxia or ventilator-induced injury. Alternatively, these findings also suggest variable effects of chorioamnionitis on lung maturation versus arrested development, in which greater prenatal injury is reflected by an increased need for more ventilator and oxygen support.
Pathophysiology
Respiratory Function
Nonuniform damage to the airways and distal lungs results in variable time constants for different areas of the lungs. Inspired gas may be distributed to relatively poorly perfused lung, thereby worsening ventilation-perfusion matching. Decreased lung compliance appears to correlate strongly with morphologic and radiographic changes in the lung. Dynamic lung compliance is markedly reduced in infants with established BPD, even in those who no longer require oxygen therapy. The reduction in compliance is due to small airway narrowing, interstitial fibrosis, edema, and atelectasis. Established BPD is primarily characterized by reduced surface area and heterogeneous lung units in which regional variations in airway resistance and tissue compliance lead to highly variable time constants throughout the lung. As a result, MV of severe BPD, especially beyond the first few months of life, requires strikingly different ventilator strategies than commonly used earlier in the disease course ( Fig. 20.8 ). Such strategies generally favor longer inspiratory times, larger tidal volumes, higher positive end-expiratory pressure (PEEP), and lower rates to allow more effective gas exchange and respiratory function.

Although the new BPD has been characterized as an arrest of distal lung and vascular growth, most of these observations were based on lung histology; evidence that provided direct physiologic data to support this finding was lacking. Tepper and colleagues have demonstrated the important finding of reduced lung surface area in infants with BPD by utilizing novel methods of assessing diffusion capacity. Their recent work provides in vivo evidence for impaired diffusion capacity secondary to both decreased alveolar surface area and decreased pulmonary capillary bed.
Pulmonary Circulation
Acute lung injury also impairs growth, structure, and function of the developing pulmonary circulation after premature birth. Endothelial cells are particularly susceptible to oxidant injury due to hyperoxia or inflammation. The media of small pulmonary arteries may also undergo striking changes, including smooth muscle cell proliferation, precocious maturation of immature pericytes into mature smooth muscle cells, and incorporation of fibroblasts into the vessel wall and surrounding adventitia. Structural changes in the lung vasculature contribute to high pulmonary vascular resistance (PVR) due to narrowing of the vessel diameter and decreased vascular compliance. Decreased angiogenesis may limit vascular surface area, causing further elevations of PVR, especially in response to high cardiac output with exercise or stress. The pulmonary circulation in BPD patients is further characterized by abnormal vasoreactivity, which also increases PVR ( Fig. 20.9 ). Abnormal pulmonary vasoreactivity is evidenced by a marked vasoconstrictor response to acute hypoxia. Cardiac catheterization studies have shown that even mild hypoxia causes marked elevations in pulmonary arterial pressure, even in infants with modest basal levels of PH. Maintaining oxygen saturation levels above 92%–94% effectively lowers pulmonary arterial pressure. Strategies to lower pulmonary arterial pressure or limit lung injury to the pulmonary vasculature may limit the subsequent development of PH in BPD.

Early injury to the lung circulation leads to the rapid development of PH, which contributes significantly to the morbidity and mortality of severe BPD. Persistent echocardiographic evidence of PH beyond the first few months has been associated with up to 40% mortality in infants with BPD. High mortality rates have been reported in infants with BPD and severe PH, especially in those who require prolonged ventilator support ( Fig. 20.10 ). Although PH is a marker of more advanced BPD, elevated PVR also causes poor right ventricular function, impaired cardiac output, limited oxygen delivery, increased pulmonary edema, and perhaps a higher risk for sudden death.

In addition to the adverse effects of PH on the clinical course of infants with BPD, the lung circulation is further characterized by persistence of abnormal or “dysmorphic” growth, including a relative paucity of small pulmonary arteries with an altered pattern of distribution within the interstitium of the distal lung. In infants with severe BPD, decreased vascular growth occurs in conjunction with marked reductions in the formation of alveoli, suggesting that the new BPD is primarily characterized by growth arrest of the developing lung. This reduction of alveolar-capillary surface area impairs gas exchange, thereby increasing the need for prolonged supplemental oxygen and ventilator therapy. This causes marked hypoxemia with acute respiratory infections and late exercise intolerance and further increases the risk for developing severe PH. Experimental studies have further shown that early injury to the developing lung can impair angiogenesis, which contributes to decreased alveolarization and simplification of distal lung airspace (the “vascular hypothesis”). Thus, abnormalities of the lung circulation in BPD are related not only to the presence or absence of PH but also, more broadly, to pulmonary vascular disease after premature birth. This becomes manifest by decreased vascular growth and structure, thus also contributing to the pathogenesis and abnormal cardiopulmonary physiology of BPD.
Clinical studies have shown that the metabolic function of the pulmonary circulation is also impaired, as reflected by the lung’s reduced clearance of circulating norepinephrine (NE). Normally, 20%–40% of circulating NE is cleared during a single passage through the lung, but infants with severe BPD have an increase, suggesting a net production of NE across the pulmonary circulation. It is unknown whether impaired metabolic function of the lung contributes to the pathophysiology of BPD or is simply a marker of severe pulmonary vascular disease. It has been speculated that high catecholamine levels may lead to left ventricular hypertrophy (LVH) or systemic hypertension, known complications of BPD.
In addition to pulmonary vascular disease and right ventricular hypertrophy, other cardiovascular abnormalities associated with BPD include LVH, systemic hypertension, and the development of prominent systemic-to-pulmonary collateral vessels. Infants with severe BPD can develop LVH in the absence of right ventricular hypertrophy. Left ventricular diastolic dysfunction can contribute to lung edema, diuretic dependency, and PH in some infants with BPD.
Prominent bronchial or other systemic-to-pulmonary collateral vessels were noted in early morphometric studies of infants with BPD and can readily be identified in many infants during cardiac catheterization. Although these collateral vessels are generally small, large collaterals may contribute to significant shunting of blood flow to the lung, resulting in edema and the need for higher levels of supplemental oxygen. Collateral vessels have been associated with high mortality in some patients with both severe BPD and PH. Some infants have improved after embolization of large collateral vessels, as reflected by a reduced need for supplemental oxygen, ventilator support, and diuretics. The contribution of collateral vessels to the pathophysiology of BPD, however, is poorly understood.
Clinical Findings
Physical Findings
Multiple abnormalities of lung structure and function contribute to late respiratory disease in BPD (see Fig. 20.5 ). Depending on the severity of BPD, findings on physical examination are variable and some infants may not have any abnormal findings. Chronic respiratory signs in children with BPD include tachypnea with shallow breathing, retractions, head bobbing, and a paradoxical breathing pattern. Auscultation may reveal coarse rhonchi, crackles, and wheezes. Some infants may demonstrate systemic hypertension during the first year of life. This systemic hypertension in BPD may be mild, transient, or striking. It usually responds to medication. The etiology remains obscure, but further evaluation of some affected infants reveals significant renovascular or urinary tract disease.
Imaging
Chest radiography is easily and frequently obtained in infants with BPD; however, its utility in diagnosis is limited owing to variable findings and the fact that many infants may have minimal radiologic abnormalities. Some patients may show lung fields with diffuse haziness. Patients with severe disease may demonstrate areas of hyperinflation and air trapping alternating with areas of fibrosis and atelectasis. Pulmonary edema may be present, especially during acute exacerbations.
Computed tomography (CT) of the chest has been used to identify structural abnormalities in patients with BPD, demonstrating increased airspace area reflecting alveolar simplification, hyperexpansion, emphysema, fibrosis and interstitial abnormalities, and mosaic attenuation. Several different scoring systems have been developed correlating with the severity of BPD. Longitudinal assessment by chest CT is not routinely used owing to concerns of exposing patients to large doses of ionizing radiation. New low-dose high-resolution CT protocols decrease this exposure, with dosing equivalent to 10–15 chest x-rays. Nevertheless, the risk of serial radiation exposure limits the utility of this imaging modality.
Magnetic resonance imaging (MRI) of the lung is an alternative imaging modality without the risk of ionizing radiation; however, this is complicated, in that lungs have low proton density and alveolar spaces contribute to rapid signal decay, making imaging technically challenging. MRI with a hyperpolarized noble gas such as helium or xenon can improve sensitivity in identifying airway abnormalities. A proof-of-concept study demonstrated the safe performance of a nonsedated hyperpolarized-gas MRI in a healthy infant, and this technique may facilitate longitudinal assessment of patients with BPD in the future.
Pulmonary Function Tests
Increased airway resistance can be demonstrated even during the first week after birth in preterm neonates at risk for BPD. Infants with BPD at 28 days of age have an increased total respiratory and expiratory resistance with severe flow limitation, especially at low lung volumes. The presence of tracheomalacia may also result in airflow limitation, which is worsened by bronchodilator therapy. In the early stages of BPD, the functional lung volume is often reduced due to atelectasis, but during later stages there is gas trapping with hyperinflation. The use of pulmonary function testing to follow the progression of BPD and the response to therapeutic interventions has increased but is still not commonly applied in the clinical setting.
Laboratory Findings
There is no specific laboratory test for the diagnosis of BPD. Patients with BPD may demonstrate hypercarbia and elevated bicarbonate levels in compensation for respiratory acidosis. Although not routinely used in clinical practice, changes in cytokine profiles have been associated with the development of BPD. Increased concentrations of IL-1β, IL-6, IL-8, IL-10, and interferon-γ and decreased concentrations of IL-17, RANTES (regulated on activation, normal T cell expressed and secreted), and TNF-β have been observed. A recent study identified markers of urinary inflammation and oxidative stress that are associated with an increased risk of developing BPD. Increased 8-hydroxydeoxyguanosine levels, a marker of oxidative stress–derived DNA damage, were associated with increased BPD. Greenough and colleagues have shown increased exhaled nitric oxide on day of life (DOL) 28 is associated with increased risk of BPD. Research continues to seek improved markers to predict or identify infants at risk for long-term pulmonary morbidities.
Echocardiographic Findings
Routine echocardiograph monitoring is recommended at 36 weeks’ corrected gestational age to assess for evidence of PH. Cardiac catheterization remains the gold standard for diagnosing PH. However, owing to its invasive nature, it is typically performed only in patients with severe disease. Tricuspid regurgitant jet velocity (TRJV) is used to estimate right ventricular systemic pressure (RVSP); however, other findings used to diagnose PH include right ventricular hypertrophy, right atrial enlargement, and interventricular septal flattening.
Prevention
As described earlier, diverse mechanisms contribute to the pathogenesis of BPD, which perhaps makes it less surprising that there is no single therapy or prevention strategy except for the prevention of premature birth. The use of antenatal steroids in mothers at high risk of delivering a premature infant has reduced the incidence of neonatal death and RDS by 50% but failed to decrease the incidence of BPD, even in combination with postnatal surfactant. Thus far, there has been no clear advantage to one antenatal steroid dosing regimen. Similarly, antenatal thyrotrophin-releasing hormone therapy did not reduce BPD in randomized trials.
Postnatally prevention of BPD begins in the delivery room. Emerging evidence suggests that avoiding or limiting MV leads to a decreased incidence of BPD. Rapid establishment of FRC has become an early goal for all VLBW infants in attempts to avoid MV. Use of a T-piece resuscitator as opposed to a self-inflating bag has been shown to decrease intubation rates. Sustained lung inflation immediately after birth is being investigated as a strategy to establish FRC and avoid lung injury. One RCT demonstrated a decreased need for MV at 72 hours; yet there was no difference in BPD at 36 weeks and an increase in pneumothorax. Results of a larger RCT are pending.
There has been a practice trend supporting early and aggressive use of noninvasive ventilation to avoid MV in hopes of decreasing BPD. Several RCTs have compared the use of early nasal continuous positive airway pressure (nCPAP) with intubation. A meta-analysis of these results reveals a small but significant decrease in the incidence of BPD or death. Additionally, early nCPAP reduced the need for subsequent intubation and was associated with a reduced rate of steroid prescription and shorter duration of ventilation.
Despite efforts to avoid intubation, 85% of infants born between 22 and 28 weeks are exposed to MV and CPAP failure rates remains high, between 40% and 60%. Although no optimal ventilation mode has emerged, it is clear from physiologic studies that tidal volumes and inspired oxygen concentrations should be reduced as low as possible to avoid hypocarbia, volutrauma, and oxygen toxicity and that lung recruitment strategies should be employed. The association of volutrauma with the development of BPD has led to the use of strategies such as permissive hypercapnia to minimize lung injury. Despite the physiologic plausibility of permissive hypercapnia as a prevention strategy for BPD, the recent permissive hypercapnia in extremely low birthweight infants (PHELBI) trial did not show a difference in BPD or death between groups targeting high compared to low carbon dioxide, suggesting that more research is needed to better understand optimal early ventilator strategies. Meta-analysis of randomized trials has demonstrated that patient-triggered ventilation does not reduce BPD; if started in the recovery phase of RDS, however, it significantly shortens weaning from MV. The results of randomized trials of high-frequency oscillatory ventilation (HFOV) or high-frequency jet ventilation (HFJV) have been inconsistent. Two large studies that incorporated prenatal steroid and surfactant replacement therapy yielded different results. In one study (which restricted entry to VLBW infants with moderate to severe hypoxemic respiratory failure following surfactant administration), there was a significant benefit from HFOV of higher survival without BPD. No substantial benefit or adverse effects of HFOV were found in the other study, however, which randomized premature infants (younger than 29 weeks) within 1 hour of birth regardless of the degree of lung disease. An explanation for those conflicting results may be that in the current era, in which modified conventional ventilation strategies are used, pulmonary benefit from HFOV may be demonstrable only in infants with moderate to severe disease. Clearly the strategy applied for either conventional or HFOV is more important than the device itself. HFOV is frequently used as “rescue” therapy in premature newborns with severe respiratory failure despite treatment with exogenous surfactant. Whether such an approach reduces BPD or improves long-term outcomes requires appropriate testing.
Exogenous surfactant therapy to treat RDS has changed the face of neonatology in the last century. Surfactant reduces the combined outcome of death and BPD but not BPD alone. Arguably, this may be due to the increased survival of very immature infants at high risk of BPD. The “intubate, surfactant, extubate” (INSURE) technique was originally described in 1994, combining early surfactant administration with rapid extubation to minimize duration of MV. This technique has been evaluated as both an early and a prophylactic intervention (within the first hour of life or in the delivery room) as well as a rescue technique once infants develop symptoms of RDS. A meta-analysis of early INSURE showed improved outcomes including decreased oxygen requirement at 28 days and less exposure to MV; however, there was no decrease in BPD or mortality. Studies of late or rescue INSURE have had a small number of participants and more studies are needed to determine if this technique should be used broadly. Several groups are currently investigating techniques to deliver surfactant to spontaneously breathing infants while avoiding intubation altogether. Ongoing multicenter RCTs are currently recruiting and hopefully will provide greater insight in the coming years.
The use of late surfactant for preterm infants on prolonged MV and thus at high risk for BPD has been evaluated in two recent RCTs. Hascoet et al. showed that late surfactant administration did not decrease the incidence of BPD; however, it did decrease long-term pulmonary morbidities including rehospitalization for respiratory illnesses and need for chest physiotherapy. Ballard et al. and the TOLSURF (Trail Of Late SURFactant) study group recently published initial data showing no difference in BPD between infants treated with late surfactant or placebo; however, long-term pulmonary data are still being collected.
Several nutritional and fluid balance strategies have been considered to decrease BPD. Although fluid restriction in the first week after birth does not reduce BPD, it is important to avoid fluid overload, administration of colloid and sodium supplementation. A retrospective evaluation of 400- to 1000-g infants demonstrated that a higher fluid intake and less weight loss in the first week of life was associated with a higher incidence of BPD. Aggressive treatment of symptomatic PDA may reduce the severity of BPD, but this remains controversial.
Appropriate nutrition, both in utero as well as postnatally, is critical in helping to promote normal lung growth, maturation, and repair. Infants born with in utero growth restriction (IUGR) have an increased risk of developing BPD. Rapid weight gain and crossing of centiles, however, may be undesirable, particularly in infants that are small for gestational age. Long-chain polyunsaturated fatty acids (LCPUFAs) are important for cell membrane function as well as for their antiinflammatory role, and animal data support that docosahexaenoic acid (DHA) in particular is critical for alveolarization. Cohort studies suggest that low levels of DHA are associated with increased BPD, and maternal DHA supplementation while breastfeeding is associated with decreased BPD. Additionally, a small clinical trial comparing soy-based versus fish oil–based lipid suggests that soy-based lipids are associated with an increased risk of BPD. Meta-analysis of seven randomized trials has demonstrated that systemic supplementation with vitamin A in sufficient quantities to establish normal serum retinol concentrations reduces oxygen dependence at 36 weeks postmenstrual age (PMA). However, follow-up studies showed no improvement in long-term respiratory outcomes in vitamin A–treated infants. Additionally, after a national vitamin A shortage, units reported no difference in BPD during the period when they were unable to provide this treatment.
One of the only pharmacologic approaches or supplements shown to reduce the risk for BPD is caffeine. A large-scale clinical trial (the Caffeine for Apnea of Prematurity, or CAP, trial) was initially developed to examine the effects of early and prolonged use of caffeine on late neurocognitive outcomes. Analysis of other endpoints related to this study suggests that caffeine significantly reduced the risk of BPD. This effect is more striking than the benefit previously observed with vitamin A treatment. Although mechanisms whereby caffeine reduced BPD risk are uncertain, treated infants also had a decreased need for surgical PDA closure, and respiratory support was discontinued earlier. A subgroup analysis of the CAP trial suggested that early initiation of caffeine (before DOL 2) provided greater reduction in BPD incidence than late initiation (DOL 3–10). This finding was replicated in three retrospective cohort studies. An ongoing RCT will compare early (DOL1) caffeine administration with late (DOL 2 or beyond).
Corticosteroid therapy, primarily directed at reducing lung inflammation, remains one of the most controversial areas of BPD care. Acute treatment with steroids improves lung mechanics and gas exchange and reduces inflammatory cells and mediators in tracheal samples of patients with BPD. Meta-analysis of randomized trials suggests that corticosteroids reduce chronic oxygen dependency at 28 days and 36 weeks PMA, both when given early (before 8 days) and late. However, there are significant concerns regarding increased mortality and adverse effects on head growth, neurodevelopmental outcomes, and lung structure, particularly with the use of dexamethasone. Other side effects include systemic hypertension, hyperglycemia, cardiac hypertrophy, intestinal perforation, poor somatic growth, sepsis, intestinal bleeding, and myocardial hypertrophy. Currently the routine use of early high-dose steroids in premature newborns is strongly discouraged, as reflected in editorial statements from the American Academy of Pediatrics. The adverse findings, however, are generally based on data from older studies that have used high doses of dexamethasone for prolonged periods of time, and many questions persist regarding the risk-benefit relationship with the use of other steroids for shorter study periods. The DART trial (Dexamethasone: A Randomized Trial) was designed to investigate if lower dose courses of dexamethasone would be safe; however, it was unable to recruit the target number of patients. The investigators showed improved extubation rates in the short term and no significant difference in long-term adverse outcomes; however, these results should be interpreted with caution given insufficient power to determine long-term safety. Doyle et al. published a meta-analysis of postnatal corticosteroid therapy and long-term outcomes, showing that the risk of death or neurodevelopment delay is inversely related to the risk of BPD. As a result, some centers advocate the use of steroids at lower doses and shorter durations (5–7 days) in ventilator-dependent infants with severe, persistent lung disease after 1–2 weeks of age. A recent study on prophylactic low-dose hydrocortisone therapy demonstrated a decreased incidence of BPD; however, it also showed a nonsignificant trend toward culture-proven sepsis. Interestingly, this study demonstrated sex-specific differences, with hydrocortisone showing greater benefit in female infants than males.
To avoid the adverse effects associated with systemic administration, steroids have also been given by inhalation. Although meta-analysis has shown no significant benefits using this route, a recent trial of early inhaled budesonide demonstrated significantly decreased rates of BPD. However, infants treated with budesonide showed a nonsignificant trend toward increased mortality and culture-proven sepsis, leaving uncertainty regarding the risk/benefit profile of inhaled steroids.
Owing to the association of U. urealyticum infection with the subsequent development of BPD, several studies have tried to determine whether treatment with macrolides would decrease the incidence of BPD. Although none of the individual studies demonstrated benefit, a meta-analysis showed a small decrease in the incidence of BPD in infants treated with azithromycin, with a number needed to treat (NNT) of 9.6 infants. Owing to the small sample size, heterogeneity of patients and unclear blinding in some of the trials, this has not yet become widespread in clinical practice and larger RCTs are needed. A subgroup analysis of one of the trials revealed a benefit in infants who were colonized with Ureaplasma.
In clinical studies, inhaled nitric oxide (iNO) therapy acutely lowered PVR and improved oxygenation in patients with PH in various settings, including premature infants with severe RDS and established BPD. Experimental data have demonstrated that iNO therapy may be lung-protective in several animal models of experimental BPD and, importantly, may be associated with increased alveolar and vascular growth. The potential role of iNO in the prevention of BPD has received considerable attention over the past decades, but the results of randomized trials for the prevention of BPD have yielded conflicting results. A large multicenter trial showed a 50% reduction in BPD and death in preterm infants who weighed more than 1000 g at birth. Another large multicenter study suggested that prolonged iNO therapy at higher doses reduces BPD when started before the second week of life and improves some respiratory outcomes during infancy. However, a third large-scale study showed no effects when administered to preterm infants with minimal respiratory disease.
Overall, these findings suggest that iNO therapy may lower the risk for BPD in some preterm infants. However, because of the differences between studies (including gestational age, onset and duration of therapy, dose, and related questions), recommendations for its routine use for the prevention of BPD were not supported at a recent NICHD-sponsored Consensus Conference.
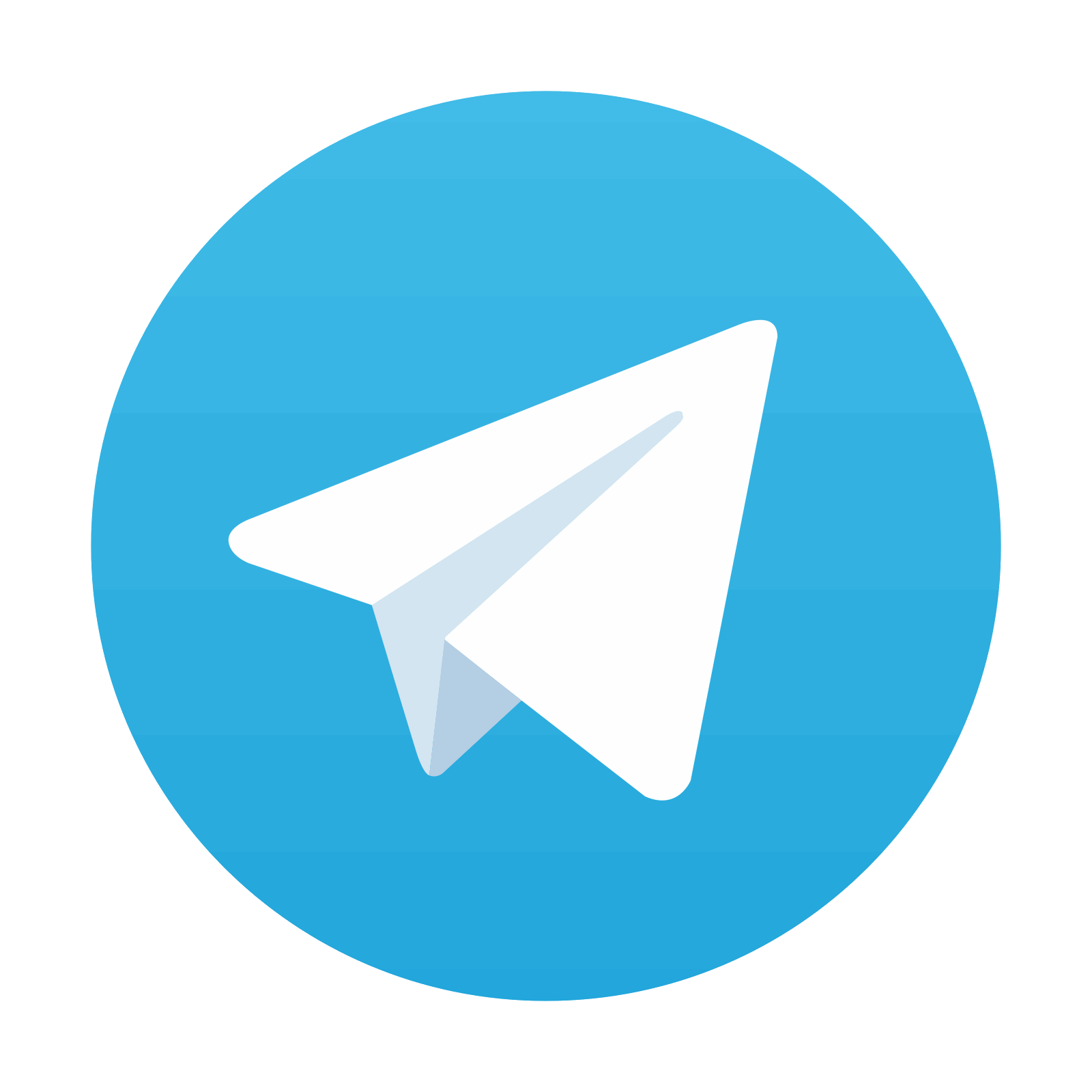
Stay updated, free articles. Join our Telegram channel

Full access? Get Clinical Tree
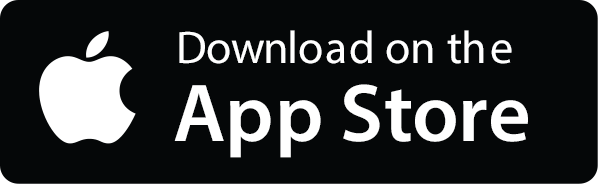
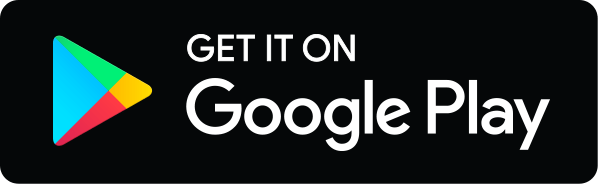