Chapter 15 Bronchodilators
Breathlessness and wheezing are cardinal symptoms of many respiratory diseases, and from the 19th century it was recognized that smoking cigarettes made from the leaves of the plant Atropa belladonna lessened the symptoms of asthma. This therapy was largely superseded when epinephrine (adrenaline) was purified in the early 20th century. Subsequently, synthetic analogues of epinephrine were developed, as was atropine, the first synthetic antimuscarinic agent. Both atropine and epinephrine could be added to organ baths containing airway smooth muscle, and their potency in relieving or preventing induced smooth muscle contraction could be measured. These developments led to the current understanding of the control of airway smooth muscle, as shown in Figure 15-1. Changes in airway smooth muscle length translate into changes in airway caliber—an especially important issue when airway diameter is reduced, as in chronic obstructive pulmonary disease (COPD) (Figure 15-2). In clinical practice, this aspect of pulmonary function is assessed indirectly as an increase in the forced expiratory volume in 1 second (FEV1) after drug administration. When a bronchodilator is given orally, the time to onset of action (i.e., improvement in lung function) is prolonged, reflecting the absorption and circulation of the drug; this time can be considerably shortened when treatment is administered by inhalation. Of note, not all drugs that increase FEV1 do so by changing airway smooth muscle activity; for example, antiinflammatory agents can reduce the mucosal thickening and thickening of the airway wall, thereby leading to improvements in FEV1. These processes take some time to develop, however, because agents such as corticosteroids have both nuclear and extranuclear effects on protein synthesis, which reduce the number of proinflammatory messenger molecules. Hence, for practical purposes, bronchodilators can be considered as those drugs with a proven direct effect on airway smooth muscle and a relatively rapid onset of effect (minutes to several hours) in clinical circumstances in which some measure of airway resistance is the marker for effectiveness.
Physiologic Basis for Bronchodilator Action
Under resting conditions in healthy people, the work of breathing performed by the respiratory muscles is relatively small. Inspiration is an active process, and sufficient flow has to be generated by overcoming respiratory resistive, elastive, and frictional loads to ensure adequate alveolar ventilation. Expiration is passive and ends when the expiratory recoil pressure of the lungs and the chest wall are balanced. There is little expiratory flow resistance within the bronchial tree, and expiratory flow limitation (no increase in flow despite increasing expiratory driving pressure) is detected only during the last part of the maximum forced expiration (Figure 15-3, top). Ventilation increases during exercise but not to the point at which flow limitation significantly limits performance.
Airway resistance is influenced significantly by the caliber of the bronchial tree (see Figure 15-2). In health, most of the resistance lies in the region of the larynx, with less than 20% coming from the periphery of the lung. The evidence points to resting airway smooth muscle tone that decreases physiologically during exercise to reduce the resistive work required when ventilation has to increase. Bronchodilator drugs abolish this smooth muscle tone in both healthy persons and patients with disease. In healthy people, the increase in FEV1 after administration of a β-agonist is between 50 and 120 mL, a value similar to that in patients with COPD, whose baseline FEV1 usually is much lower. By contrast, in patients with asthma, in which airway smooth muscle bulk is greater and resting muscle tone may be increased by indirect reflex mechanisms, the response to bronchodilator drugs is more dramatic, and substantial increases in lung function occur after the acute administration of a bronchodilator. The improvement in lung emptying that results from bronchodilation has important effects on the operating lung volume and hence on the work of breathing.
In general, wheezing reflects areas of local flow limitation within the airway. Expiratory flow limitation can occur in the absence of audible wheeze and contributes to the slow emptying of the lungs and the higher lung volumes that lead to breathlessness and chest tightness in obstructive lung disease. Expiratory flow limitation may be abolished completely after a bronchodilator drug in asthma (see Figure 15-3), although asthmatic symptoms often will recur when the effects of the bronchodilator wear off (see further on). In COPD, the absolute change in lung function is much smaller than in asthma, and flow limitation often persists, particularly in severe disease, although to a less severe degree. These subtle changes in lung mechanics, however, can produce clinically relevant changes in operating lung volumes, particularly in the end-expiratory lung volume, which is significantly elevated in many patients with COPD and constitutes is a good guide to the degree of exercise impairment experienced by affected persons. A further effect of bronchodilator drugs is to increase the threshold at which symptoms are induced. This increased threshold is important in the prevention of exercise-induced asthma and also in the reduction of symptoms produced more predictably by exercise, as occurs when patients with COPD undertake certain daily activities. This ability to increase baseline lung function and reduce the impact of external stimuli on the airways is likely to be important in explaining why bronchodilator drugs are associated with fewer exacerbations of airways disease when given in effective doses over the long term.
Pharmacologic Basis of Bronchodilator Action
β-Agonists
Based on the classical work of Ahlquist in defining different subtypes of adrenoreceptors, a range of relatively specific β-agonist agents were developed. Because β2-receptors are almost the only subtype expressed on human airway smooth muscle, it makes sense to use highly selective β-agonists and there is no place for nonselective agents in clinical practice today. The chemical structures of the principal β-agonists are shown in Figure 15-4.
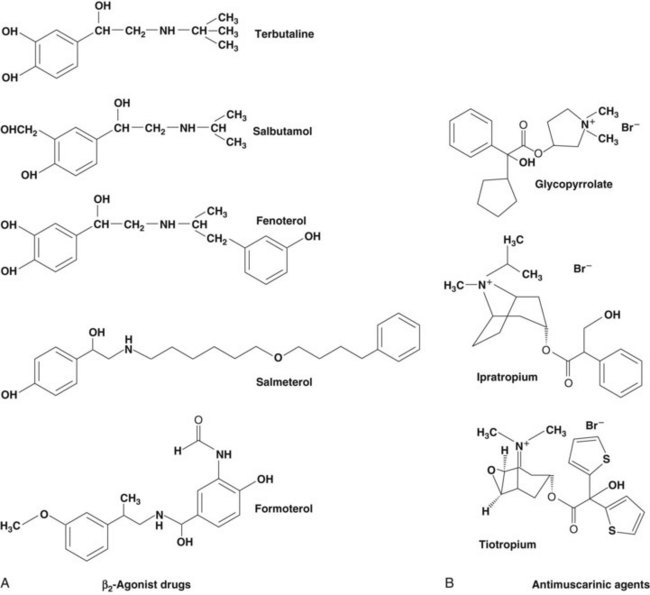
Figure 15-4 Chemical structures of commonly used bronchodilator drugs. A, β2-Agonists. B, Antimuscarinic drugs.
• A fall in the intracellular calcium ion (Ca2+) concentration by active removal of Ca2+ from the cell into intracellular stores
• Inhibition of phosphoinositide hydrolysis
• Direct inhibition of myosin light chain kinase
• Opening of large-conductance, calcium-activated potassium channels (KCa) that repolarize the smooth muscle cell and may stimulate the sequestration of Ca2+ into intracellular stores
• β2-Agonists act as functional antagonists and reverse bronchoconstriction, irrespective of the contractile agent. This is an important property, because multiple bronchoconstrictor mediators (inflammatory mediators and neurotransmitters) are released in asthma.
• Inhibition of mediator release from mast cells and other inflammatory cells
• Inhibitory effects on neutrophil migration and activation
• Reduction and prevention of microvascular leakage and, consequently, development of bronchial mucosal edema after exposure to mediators such as histamine and leukotrienes
• Increased mucus secretion from submucosal glands and ion transport across airway epithelium (effects that may enhance mucociliary clearance, thereby reversing the defect in clearance found in asthma)
• Reduction in neurotransmitter release from airway cholinergic nerves, thus reducing cholinergic reflex bronchoconstriction
• Inhibition of the release of bronchoconstrictor and inflammatory peptides, such as substance P, from sensory nerves
How relevant any of these effects are to the observed clinical effects in disease is hard to determine. They may be more important in preventing bronchoconstriction from other stimuli in asthmatic patients than in directly influencing airway dimensions. The immediate impact on airway smooth muscle remains the most important of these various mechanisms, as illustrated in Figure 15-5. Recognized polymorphisms of the β-receptor show variable responses to agonist drugs in vitro. Translation of these observations into clinically noticeable differences in response in the clinical setting has been difficult, however, and at present these observations remain primarily of academic interest.
Antimuscarinic Agents
Antimuscarinic agents are specific antagonists of muscarinic receptors and inhibit cholinergic nerve–induced bronchoconstriction. Muscarinic receptors bind to acetylcholine released after stimulation of the parasympathetic nerves. These nerves innervate the bronchi and small airways of the human bronchial tree but do not extend to the respiratory bronchioles and alveoli. The receptor has seven membrane-spanning loops, the third of which shows considerable heterogeneity—explaining the existence of several different functional variants. Many of these receptors (M1 to M3) occur in different sites and with rather different functions, as shown in Figure 15-6.
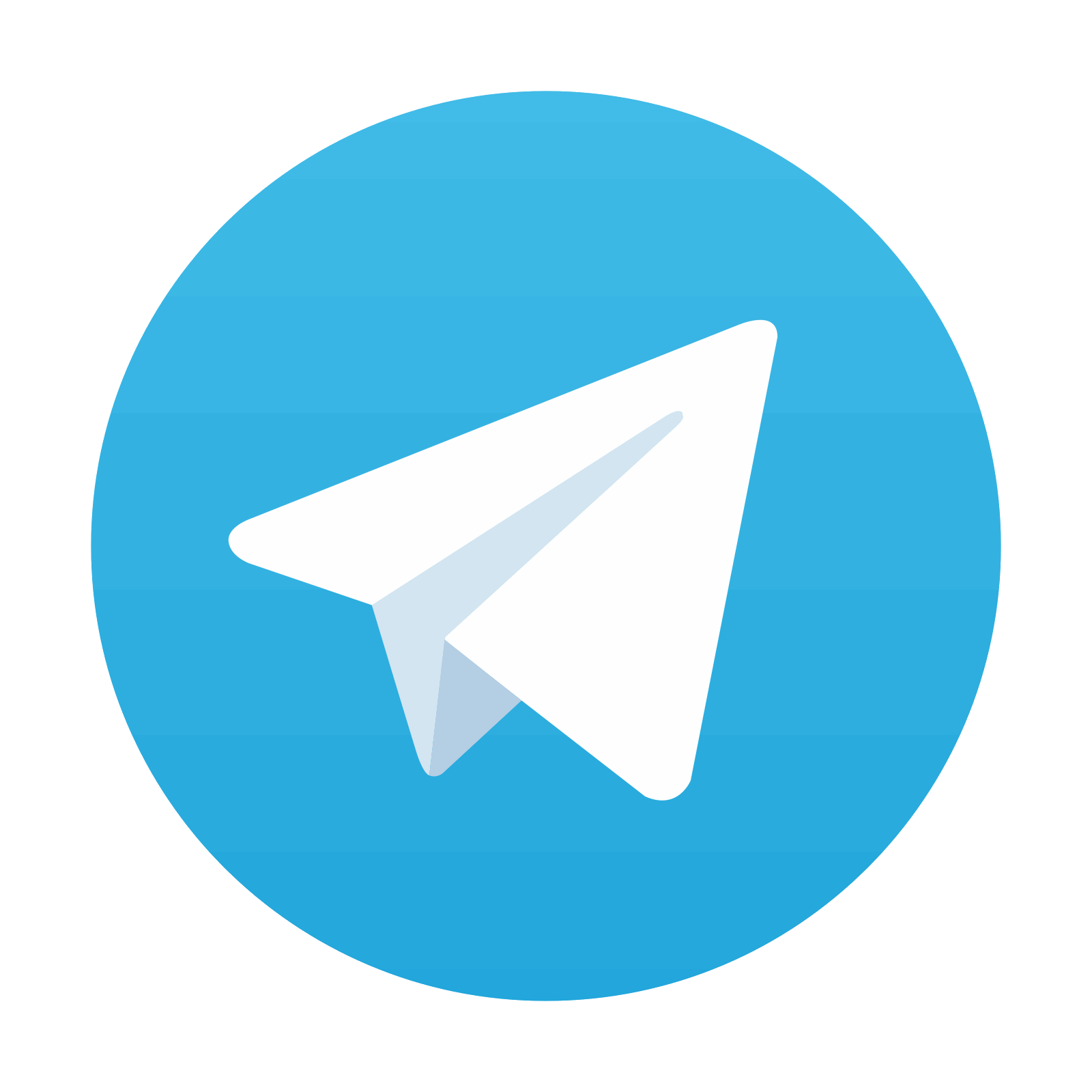
Stay updated, free articles. Join our Telegram channel

Full access? Get Clinical Tree
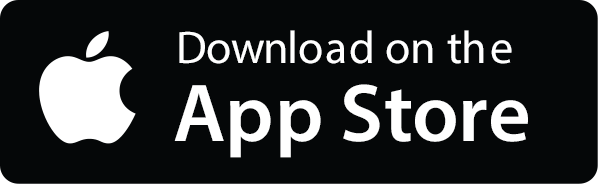
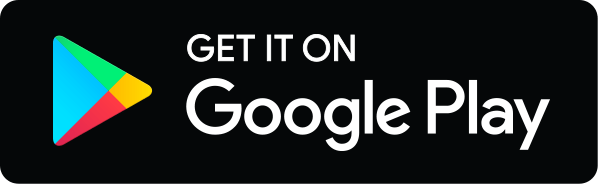