Lateral pressure exerted by the column of blood against the arterial wall is called the arterial pressure (blood pressure), and this pressure is referred to as the height of the column of blood supported by the force within a blood vessel. In a cardiac cycle, the highest pressure attained is the systolic pressure and the lowest pressure is the diastolic pressure. The equation “MAP – RAP = CO × TPR” is used to derive mean arterial pressure (MAP), where RAP is right arterial pressure, CO is cardiac output, and TPR is total peripheral resistance. Since RAP is very small, MAP (mm Hg) is the product of CO (liters per minute) and total peripheral resistance (mm Hg/liter/minute). The MAP is the geometric mean, and the calculation of MAP requires integration of pressure pulse.
An approximate estimate of MAP can be derived from the following equations:
Normal arterial pressure varies with age. It is approximately 70/50 mm Hg on the first day after birth, and gradually increases during the next several months to approximately 90/60 mm Hg. During subsequent years the rise is very slow and reaches 115/70 mm Hg at adolescence. There is a progressive increase in the pressure with age in the average population. The systolic pressure rises approximately 1 mm Hg/yr from 110 mm Hg at the age of 15 years. This probably reflects progressive reduction in arterial compliance. Diastolic pressure increases approximately 0.4 mm Hg/yr from 70 mm Hg at the age of 15 years. This rise probably reflects an increase in total peripheral resistance. The progressive increase in arterial pressure with age could also result from the effects of aging on the long-term blood pressure control mechanisms. Although average pressure in a population rises with age, the pressure never rises with age in certain people. MAP for a population is composed of individuals whose blood pressure does not change with advancing age and of individuals whose pressure increases with advancing age. There is no dividing line between normal and high blood pressure. An arbitrary level of normal blood pressure has been established to define those who have an increased risk of developing morbid cardiovascular events and/or clearly benefit from medical therapy. Arterial pressure is somewhat damaging. Life expectancy is inversely proportional to arterial pressures. The logic is to define hypertension at levels where treatment can provide benefits that outweigh risks. Males with normal diastolic pressure but elevated systolic pressure (> 158 mm Hg) have a 2.5-fold increase in cardiovascular mortality rates when compared to individuals with similar diastolic pressure but normal systolic pressure.
Arterial pressure is slightly higher in the right arm than in the left arm. Simultaneous measurement of blood pressure in both arms shows a difference of 10 mm Hg (both systolic and diastolic) in approximately 3% of normotensive and 6% of hypertensive subjects. However, when the measurements are not made simultaneously a difference of 10 mm Hg or more in systolic pressure is observed in 20% of normotensive and 30% of hypertensive individuals, and a difference in diastolic pressure of 10 mm Hg or more is observed in 10% of normotensive and 15% of hypertensive subjects. Although diastolic pressure is similar in the arms and thighs, systolic pressure is 10 to 40 mm Hg higher in thighs than in arms. The Joint National Committee on Prevention, Detection, Evaluation and Treatment of High Blood Pressure defines systolic and diastolic pressures less than 120 and 80 mm Hg, respectively, as normal.1 The guideline committee of the European Society of Hypertension—European Society of Cardiology defines systolic and diastolic pressures <120 mm Hg and <80 mm Hg, respectively as optimal; systolic pressure of 120 to 129, and diastolic pressure of 80 to 84 mm Hg as normal; and systolic pressure, 130 to 139, and diastolic pressure 85 to 89 mm Hg as high normal.2 Both guidelines consider a patient as hypertensive when the systolic and diastolic pressures are ≥140 mm Hg and ≥90 mm Hg, respectively.
Factors that determine systolic pressure include stroke volume, peak systolic rate of cardiac ejection, and arterial compliance (distensability of arterial wall). Systolic pressure increases with increases in stroke volume and peak systolic ejection rate, and a decrease in arterial compliance. Decreases in systolic pressure are associated with decreases in stroke volume and peak systolic ejection rate, and an increase in arterial compliance. Diastolic pressure is determined by total peripheral vascular resistance, heart rate, systolic pressure, and arterial elastic recoil. Increases in these parameters increase the diastolic pressure and vice versa. Loss of elastic recoil in the arterial wall that occurs with aging decreases the diastolic pressure.
Pulse pressure (PP) describes the oscillation around the MAP and is the difference between systolic and diastolic pressure. PP is influenced by the velocity of ventricular ejection, elastic properties of the arterial wall, the timing of the reflected waves, and the total peripheral vascular resistance. PP is approximately one-half of the diastolic pressure and is affected by various clinical situations (Table 8-1). A wide PP reflects systolic hypertension and increased central arterial stiffness. In hypertensives a wide PP is a reasonable surrogate for increased arterial stiffness. PP has both diagnostic and prognostic values. The mechanism underlying the prognostic effect of PP may be because of the fact that cardiovascular risk is strictly related to the pulsatile stress caused by large artery stiffness during systole. Arterial stiffness has two consequences: (a) It increases systolic blood pressure which in turn increases left ventricular afterload and mass and (b) it lowers diastolic pressure resulting in decreased coronary artery perfusion.
Increased | Decreased |
---|---|
|
|
Increased PP elevates left ventricular systolic wall stress, decreases coronary flow reserve, impairs left ventricular relaxation, and may lead to diastolic dysfunction. Increased PP is the major factor in the development of left ventricular hypertrophy with increased requirement for coronary flow. A decrease in diastolic pressure compromises oxygen supply by reducing coronary flow. Wide PP also leads to endothelial dysfunction with greater propensity for coronary atherosclerosis and rupture of unstable atherosclerotic plaques. Wide PP may simply serve as a marker for diffuse atherosclerosis. There is an independent relationship among PP, left ventricular mass index, carotid intima-media thickness, and carotid cross-sectional area. Elevated PP is an effective predictor of cardiovascular risk in hypertensive subjects.3 Wide PP is a powerful predictor of myocardial infarction in patients with hypertension. It is associated with aging, high left ventricular stroke volume, abnormal left ventricular function, cardiovascular mortality, and stroke. Wide PP has been identified as an independent predictor of coronary events4,5 and is independently associated with total mortality in the Balloon Angioplasty Revascularization Investigation.6 Systolic blood pressure is superior to diastolic blood pressure as a predictor of coronary artery disease risk after the age of 50 years. PP is a better predictor than systolic or diastolic blood pressure. Pulse wave velocity is a clinical surrogate for large arterial stiffness. Increased pulse wave reflections are associated with the presence and extent of coronary artery disease.7 Increased wave reflections are independently associated with increased risk for cardiovascular events in patients undergoing PCI.8
Blood pressure varies throughout the day and with various activities.
Diurnal variation—Blood pressure changes are associated with levels of arousal. On the onset of sleep, blood pressure falls gradually and reaches the lowest level (decrease of 15%–20%) after 2 hours. The blood pressure is higher between 10 am and 6 pm, and lower at night with a dip between midnight and 3 am. There is a slow and steady rise in blood pressure between 3 am and 6 am, and there is a sudden and steep rise shortly after awakening. The early morning blood pressure surge continues for approximately 4 to 6 hours.9 The early morning surge in blood pressure probably is because of an increase in sympathetic activity, and a rise in plasma renin and cortisol. The increase during the early hours of the morning could contribute to the high incidence of cerebral hemorrhage and myocardial infarction in these hours.10 Diurnal variation may be because of morning diurnal peaks in total blood volume, central blood volume, sympathetic activity, and plasma renin activity.11
Circadian rhythm in arterial pressure of patients with hypertension is similar to that of patients with normotension except that the entire blood pressure profile is shifted upward. Circadian rhythm for blood pressure is lost following an acute stroke. There is a paradoxical elevation of pressure during the first part of the night and the lowest pressure of the day in the morning in patients with idiopathic orthostatic hypotension.
Physical activity—Physical activity raises blood pressure. The type of activity determines the amount of rise in blood pressure. Talking raises blood pressure. Severe physical activity can raise arterial pressure to a level of 240 mm Hg.
Meals—There is an increase in the heart rate, a decrease in the diastolic pressure, and a little change in systolic pressure for 3 hours after a meal.12 There is a marked fall in both systolic and diastolic pressure in older individuals following a meal.
Seasonal variation—Blood pressure is approximately 5 to 9 mm Hg higher in winter than in summer in temperate climates. Possibly cold stimulates the vasoconstrictor area. Warmer climates are vasodilative. The systemic response to cold is vasoconstriction through a reflex mechanism.
Mental activity—Mental activity elevates blood pressure.
Sex—Systolic and diastolic pressures are lower in women than in men younger than 40 to 50 years, and are higher in women than in men older than 50 years. This difference could be because of the hormonal changes in women that take place at menopause.
Weight—Systolic and diastolic pressures are directly related to the weight of the individual.
Posture—In the erect position, cardiac output and thoracic arterial pressure decrease because of a decrease in venous return. There is a reflex increase in the heart rate and systemic vascular resistance resulting in an increase in both systolic and diastolic pressure, the increase being greater in the latter.
Pain—Headache and other types of chronic pain elevate arterial pressure.
Hypoglycemia—A rapid decrease in blood sugar stimulates the sympathoadrenal system and blood pressure rises. In some cases it might reach the range of 250/150 mm Hg.
Race and socioeconomic status—Blood pressures are higher in blacks than in whites, across all ages and for both sexes. Genetic and environmental factors, particularly socioeconomic, may be involved in this difference.
Arterial pressure moves blood throughout the body to provide nutrients to the tissue. Regulation of arterial pressure is essential to maintain tissue perfusion during various pathophysiologic conditions. Arterial pressure is regulated essentially by three mechanisms: (a) a rapidly acting pressure control mechanism, (b) an intermediate time-period pressure control mechanism, and (c) a long-term pressure control mechanism.
A brief review of the autonomic control of the cardiovascular system is described below.13,14,15 The organization of the vasomotor center and autonomic nervous system is shown in Figure 8-1. The vasomotor center is situated bilaterally in the medulla and lower third of the pons. It comprises three regions namely, vasoconstrictor, vasodilator, and sensory nucleus tractus solitarius (NTS). The vasoconstrictor region is located in the dorsolateral part of the medulla. Fibers from the vasoconstrictor regions descend and synapse in the intermediolateral gray matter of the spinal cord at different levels of the thoracolumbar region (T1 to L2 or L3). The fibers (preganglionic) then leave the cord to synapse with paravertebral sympathetic ganglia. Postganglionic sympathetic fibers from various ganglia innervate the arteries, veins, and heart. Norepinephrine released at the postganglionic nerve endings binds to alpha-adrenergic receptors on vascular smooth muscle cells to cause vasoconstriction.
Sympathetic nerve fibers innervate all the vessels except precapillary sphinctors, capillaries, and meta-arterioles. Sympathetic stimulation constricts the resistance vessels (arterioles) thus increasing the total vascular resistance. Constriction of capacitance vessels (venules and small veins) pushes the blood into the heart resulting in increased stroke volume. Sympathetic stimulation to the heart increases the heart rate and contraction, resulting in an increase in cardiac output. It should be pointed out that an increase in peripheral resistance raises blood pressure but decreases the organ perfusion. Acting on the heart, norepinephrine increases the heart rate, force of contraction, and conduction in the heart. The adrenergic receptors in the nodal regions and in the myocardium are predominantly of the beta types. The vasoconstrictor region is tonically active, fires continuously at a rate of one-half to two impulses per second, and maintains basal tone in the blood vessels. Stimulation of the vasoconstrictor region increases tonic activity and hence increases the frequency of impulse formation in the sympathetic nerves, resulting in vasoconstriction and increases in heart rate and force of cardiac contraction. On the other hand, inhibition of the vasoconstrictor region will have opposite effects. The vasoconstrictor region may show rhythmic changes in tonic activity resulting in oscillation of arterial pressure. Traube–Hering waves occur at the frequency of respiration and are caused by an increase in sympathetic activity during inspiration. Myer waves, on the other hand, are caused by oscillation of baroreceptor and chemoreceptor reflexes, and central nervous system (CNS) ischemic response.
The vasodepressor region is located caudal and ventromedial to the vasoconstrictor region, and produces decreases in blood pressure, heart rate, and myocardial contractility when stimulated.
The NTS in the medulla is the sensory termination of both vagal and glossopharyngeal nerves that transmit sensory signals from chemoreceptors, baroreceptors, and several other peripheral receptors. All the signals from the peripheral areas help control blood pressure. The NTS in the medulla controls autonomic functions such as peripheral arterial pressure, heart rate, and myocardial contractility.
The parasympathetic nervous system has two divisions: cranial (III, VII, IX, and X nerves) and sacral (second through fourth sacral nerves). Approximately 75% of all parasympathetic nerve fibers are in the vagus nerve that arises in the dorsal vagal nucleus in the medulla lateral to the hypoglossal nuclei. These fibers synapse with ganglia close to the organs. At the postganglionic nerve ending, acetycholine is released. Acetylcholine relaxes blood vessels and decreases the heart rate, myocardial contractility, and conduction in the atrioventricular node. The cranial division of parasympathetic fibers supply blood vessels of the heart and viscera, while fibers from the sacral division supply blood vessels of the genitalia, bladder, and large intestine.
The vasomotor center is affected by stimuli from other areas of the brain. The hypothalamus plays a crucial role in the control of blood pressure and cardiac function through behavioral and emotional changes. Stimulation of the anterior portion of the hypothalamus causes mild excitation of inhibition depending upon which part of the anterior portion of the hypothalamus is stimulated. In general, stimulation of the anterior portion of the hypothalamus produces hypotension and bradycardia, whereas stimulation of the posterolateral region of the hypothalamus produces a rise in arterial pressure and tachycardia. The hypothalamus contains a temperature-regulating center. Stimulation by cold application to the skin or cold blood reaching the hypothalamus results in constriction of skin vessels, while a warm stimulus causes cutaneous vasculation.
Stimulation of the motor cortex and premotor area can excite or inhibit the vasomotor center through the hypothalamus. Stimulation results in a vasopressor effect. Vasodilation and vasodepressor responses may occur as blushing or fainting in response to emotional stimuli.
Painful stimuli may result in pressor or repressor responses depending upon the magnitude and location of the stimuli. Distension of the abdominal viscera often evokes a vasodepressor response, while a painful stimulus on the body surface usually evokes a pressor response.
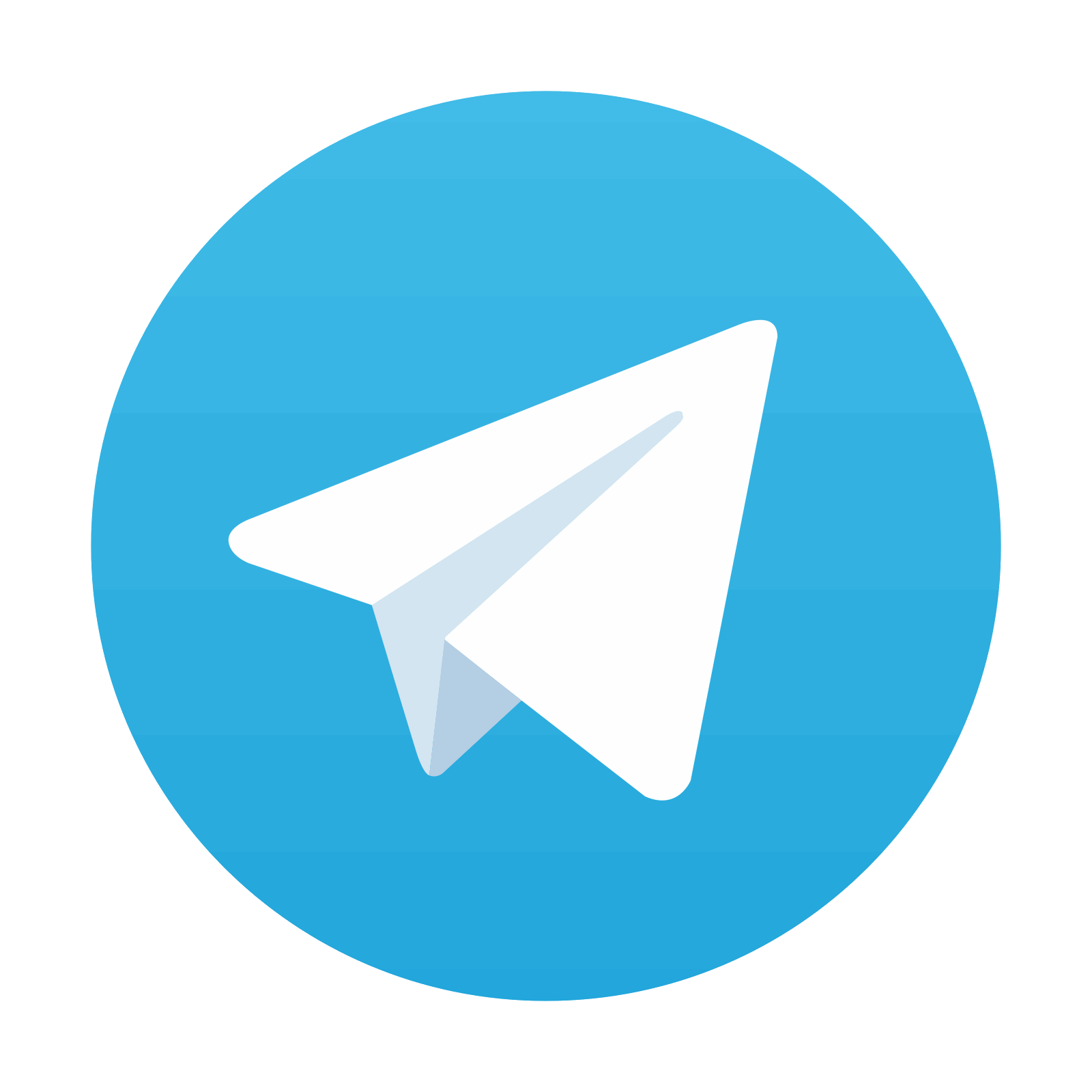
Stay updated, free articles. Join our Telegram channel

Full access? Get Clinical Tree
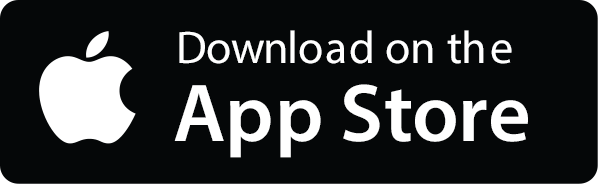
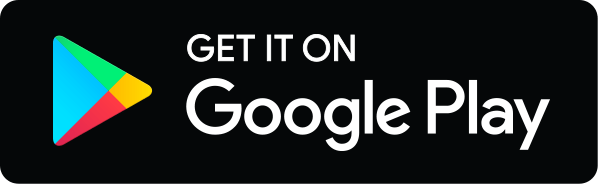