Chapter 94
Biologic Grafts
Jeffrey Kalish, Alik Farber
Based on a chapter in the seventh edition by Alik Farber
In the field of vascular surgery, the use of surgical bypass is fundamental to the treatment of a wide variety of arterial and venous disorders. In turn, the technical conduct and success of surgical bypass are directly dependent on the conduit used. The ideal conduit should be readily available, easy to handle, resistant to thrombosis and infection, durable, inexpensive, and should have characteristics similar to the vessel that it is replacing.
Although the perfect conduit does not exist, autogenous blood vessels are closest to the ideal. Autogenous arterial conduits, such as the internal mammary, radial, and gastroepiploic arteries, have been used with great success in the coronary circulation.1–3 The internal iliac and radial arteries have been used in the visceral vascular bed,4,5 and the superficial temporal artery has been used for extracranial-intracranial bypass.6 Unfortunately, short conduit length and invasive harvest have limited the use of autogenous arterial grafts to a relatively small number of clinical scenarios.
Autogenous vein has been the preferred conduit for infrainguinal bypass because long lengths of vein can be harvested, removal is inconsequential, and harvest complexity is minimal.7 Autogenous veins have been used for the bypass of upper extremity,8 carotid,9 coronary,1 and visceral10 arterial beds. They have also been preferentially used in the construction of arteriovenous fistulae (AVFs) for hemodialysis.11
Although autogenous vascular grafts perform well, there are multiple clinical situations in which these conduits are inadequate, unavailable, or improperly matched to the recipient vascular bed. These unmet demands led to the development of artificial grafts. Although multiple materials have been tried, polyethylene terephthalate (Dacron, DuPont, Wilmington, Del) and polytetrafluoroethylene (PTFE) have emerged as the standard materials for prosthetic vascular grafts. These grafts have been used with excellent success for the bypass of large vessels, such as the aorta and iliac arteries,12 and medium-sized vessels, such as the subclavian artery.9 Prosthetic grafts have also been used extensively for dialysis access11 and with mixed results for infrainguinal revascularization.13 Dacron and PTFE grafts offer “off-the-shelf” availability and a variety of sizes to permit replacement of even the largest vessels. In general, however, they cannot be used in infected fields, and compared with autogenous conduits, are at increased risk for infection, structural deterioration, and occlusion. Although patency rates are acceptable for aorto-iliac reconstruction because of high flow rates and low outflow resistance, bypass to smaller targets, such as the tibial arteries, is associated with low graft patency.
Limitations of autogenous and prosthetic grafts have fueled exploration for other potential conduits, and this investigative effort has led to the evaluation of biologic grafts for bypass. Biologic grafts, or biografts, are bypass conduits made of nonautogenous biologic vessels modified for use in clinical practice. Allografts or homografts refer to arteries or veins that are transplanted from one individual to another within the same species. Xenografts or heterografts are vessels transplanted from an individual of one species to an individual of another species.
Carrel14 was first to experiment with fresh allografts and xenografts in dogs during the first decade of the 20th century. The first recorded human use of allografts, obtained from casualties, occurred during World War I.15 In 1948, Gross et al16 described the first clinical series of fresh arterial allografts, and less than a decade later, Linton17 published his series of fresh venous allografts. Various methods of allograft cryopreservation were developed in the 1950s,17 refined in the 1970s,18,19 and finally standardized and commercialized in the late 1980s.20 In parallel, enzymatically treated and tanned bovine carotid artery (BCA) xenografts were evaluated and first described in a clinical setting in 1966.21 Application of similar techniques to human vessels led to development of the human umbilical vein (HUV) graft by Dardik and Dardik in 1976.22
Theoretically, biografts promise to be the optimal vascular conduit. They can potentially offer “off-the-shelf” availability, a wide variety of sizes, excellent handling characteristics, and patency rates similar to those of autogenous vessels. These attractive features prompted scientific investigation and clinical use of these conduits that has spanned the course of almost a century. Although this collective experience with an assortment of biografts in a variety of clinical settings led to specific clinical indications for their use, biologic grafts have failed to become the “Holy Grail” of vascular surgery.
Graft Properties
Fresh Vascular Allografts
Fresh arterial and venous allografts have been studied in animal experiments.14,23,24 In one canine model, fresh venous allografts had a patency rate of 69% at 20 months. Pathologic analysis of explanted veins revealed intimal proliferation, medial inflammation, medial degeneration, and periadventitial fibrosis.23 In another canine venous allograft experiment, dogs that were immunosuppressed with azathioprine demonstrated slightly better graft patency than did those that were not.24 Conversely, in a murine model, fresh venous allografts implanted in rats had excellent patency rates and minimal intimal thickening on histologic analysis.24
In humans, fresh venous allografts used for infrainguinal bypass had a failure rate of 55% in one series; failed grafts either occluded or became aneurysmal. Patency rates of allografts appeared to be higher in patients whose grafts were harvested from blood types ABO compatible donors.25 In another study, fresh arterial allografts placed in the aortic position were noted to be highly immunogenic, with evidence of both a humoral and cellular immune response.26 These animal and human data suggest that fresh vessel allografts initiate a host immune response. Furthermore, the patency of these grafts appears to vary among species.
Aside from their immunogenicity, the use of fresh vascular allografts in the clinical setting has been hampered by logistic factors. Scarce availability of fresh arteries and veins and a need to successfully store such vessels for future use have led to the development of a number of preservation and modification techniques. These techniques can be divided into those that involve preservation without a planned significant change in graft integrity and those in which the graft is intentionally chemically altered. Cryopreservation is the most common example of the preservation technique, whereas proteolytic enzymatic digestion and dialdehyde starch tanning are examples of the modification technique. In addition to creating a conduit that would be more readily available, there it is hoped that these techniques will inhibit the host immune response and thereby increase graft patency.27
Over the course of the past century, multiple vessel preservation techniques have been tested. Grafts were stored in a number of solutions, including nutrient broths,16 glycerol,28 and plasminate.29 A variety of storage temperatures ranging from room temperature to −70°C were tried.17,28 Finally, a number of adjunctive sterilization techniques, including ethyl dioxide and irradiation, were attempted.17 Early techniques focused on preservation without much regard to viability of the vascular tissue. Initial results with preserved vascular grafts were inconsistent, probably because significant cellular and structural damage occurred in many of these vessels and made them nonviable.25,30
Cryopreserved Allografts
Methods of Preparation
There is evidence that cryopreservation can result in significant cellular damage unless appropriate precautions are taken.31 During the cryopreservation process, the extracellular matrix freezes at a higher temperature than cellular cytoplasm. This leads to a vapor pressure gradient between the intracellular and extracellular components. When cooling occurs slowly, this gradient can result in cellular dehydration, whereas rapid cooling can lead to plasma membrane rupture. Work with cell suspensions, such as blood and semen, has revealed that certain substances, when added during the freezing process, can significantly improve cell viability.32 These substances, called cryoprotectants, include dimethylsulfoxide and glycerol. Their mechanism of action is to enter cellular cytoplasm and decrease the vapor pressure gradient that exists between the intracellular and extracellular components.20
Over the last 20 years, cryopreservation techniques have been optimized and commercialized. Important variables inherent in modern cryopreservation processes include the type and amount of cryoprotectant used, freezing rate, storage temperature, duration of storage, and additives used.20 The most common cryoprotectant in use today is dimethylsulfoxide at 10% to 20% dilution. The freezing rate varies among protocols, and there is some evidence that rapid freezing at 5°C/s may work best. Storage temperature may vary from −102°C to −196°C. The duration of cryopreservation may be important, and longer duration has been shown to have an adverse influence on vessel wall morphology but not on graft patency in one animal model.33 Finally, there is evidence that the addition of certain additives such as chondroitin sulfate to the storage solution enhances vein viability and function.34
Histology and Physiology
Cryopreserved arteries and veins are affected by both cryopreservation and immune rejection; a large body of research has been performed to define and dissect these processes from one another.
Cryopreservation has effects on the mechanical properties, histology, and physiology of the treated vessel. Elasticity and compliance of a vessel are important mechanical characteristics that affect its performance as a conduit. Changes in these properties lead to an increased difference in compliance between the conduit and host vessel, which can adversely affect graft patency. In vitro models comparing the mechanical properties of cryopreserved and freshly harvested arteries and veins reveal that cryopreservation does not significantly affect elasticity, contractility, compliance, and the mechanical buffering function of the treated vessel.19,35,36
Cryopreservation of blood vessels leads to changes in the intima, media, and adventitia. Although appropriate cryopreservation does not affect the gross morphology of the endothelial layer, histologic changes such as focal microvillous projection, cytoplasmic vacuolization, nuclear prominence, and interruption of tight junctions have been visualized.33,37 These changes increase with the duration of cryopreservation33,37 and lead to partial endothelial cell loss.37–39 Endothelial loss is significant when the cryopreserved graft is exposed to arterial flow. Although autogenous grafts re-establish an endothelial layer, only minimal re-endothelialization is observed in allografts.20,29 Because of a compromise in intimal integrity, cryopreserved grafts accumulate low-density lipoprotein cholesterol at an accelerated rate as measured in an ex-vivo organ perfusion system.40
Endothelial vasodilatory function, as measured by response to acetylcholine, thrombin, and calcium ionophore, appears to be retained, but is somewhat diminished, with cryopreservation.41 With regard to coagulation homeostasis, although cryopreservation of vein grafts is not associated with increased platelet deposition,41 it does cause decreased thrombomodulin activity.38 Fibrinolytic activity appears to be similar in both fresh and cryopreserved canine jugular veins, but this activity may be adversely affected by the duration of cryopreservation.37
The medial layer of cryopreserved vascular grafts appears to have grossly normal smooth muscle cells, although slight lysis and minimal mitochondrial edema were observed in a rabbit model. In that model, implantation of autologous veins into an arterial circuit led to the preservation of both smooth muscle cells and the elastic lamina. The smooth muscle cells displayed a synthetic, rather than a contractile, phenotype characterized by dilatation of the endoplasmic reticulum.33 Despite these findings, collagen synthesis in cryopreserved veins, but not fresh veins, was diminished in a canine model.39 Smooth muscle cells in cryopreserved canine saphenous autografts were noted to have a diminished relaxation response to nitric oxide,41 although contraction induced by norepinephrine, potassium chloride, and serotonin was unaltered.20
Immunology
Allogeneic implantation of cryopreserved vessels leads to a different histologic and physiologic picture than that seen with cryopreserved autologous grafts. These observed changes are caused by immune mechanisms. Endothelial loss, encountered when an allograft is exposed to arterial flow, is not appreciably reversed, and exposed subendothelial elements are noted on electron microscopy.20,29 Smooth muscle cell viability is lost,23,42 severe medial fibrosis and disruption of elastic fibers occur,23,29,42 and medial necrosis has been described.43 Significant lymphocytic infiltration of the media and adventitia has been observed.29 These alterations in vessel wall biology are not routinely observed with autologous conduits.43
Although it is well known that transplanted allograft and xenograft organs elicit an immune response, it was initially believed that the host-mediated immune response of transplanted vessel allografts was minor44,45 and could be successfully blunted by the cryopreservation process.27 Recent literature, however, suggests that vascular allografts do trigger a significant immune response.20,26 Endothelial cells present surface antigens that stimulate a cell-mediated immune response46 against the donor graft. An immunoglobulin-G–mediated humoral immune response to donor-specific antigens has been described.26,47 Transplanted canine venous allografts, but not autografts, demonstrated extensive medial fibrosis and lymphocytic infiltration consistent with immunologic rejection.48 In a human model, analysis of 22 explanted cryopreserved saphenous vein (CSV) allografts revealed moderate to severe intimal, medial, and adventitial inflammatory infiltrates. Immunohistochemical analysis demonstrated an abundance of activated T lymphocytes containing cytotoxic granules.49 In another experiment, cryopreservation did not alter antigenic expression and the immunologic response of a murine host to allograft transplantation in a number of studies.50,51 Chronic immunologic rejection clearly plays a role in allograft biology and appears to be responsible for both diminished patency of cryopreserved vascular grafts and the predilection of these grafts to aneurysmal degeneration.43
A number of investigators hypothesized that manipulating the host immune response to vascular allografts may attenuate immune rejection and improve graft patency. Matching of ABO blood groups was suggested by Ochsner et al,25 who noted improved patency of allografts transplanted to ABO-matched patients. In animal models, immunosuppression with cyclosporine has been demonstrated to diminish immunologic rejection of aortic43 and venous allografts.52 Azathioprine has likewise been shown to decrease the effects of rejection in venous allografts.24
Based on these findings, attempts were made to improve the results of allograft use in humans by modulating the host immune response. Carpenter and Tomaszewski,53 in a prospective, randomized trial of 40 CSV allografts implanted in patients treated with low-dose azathioprine, failed to show a significant improvement in graft patency at 1 year. Azathioprine immunosuppression, however, was associated with a decreased presence of T-lymphocyte cytotoxic granules in that study.49 In another small human trial, a combination of low-dose cyclosporine, azathioprine, prednisone, warfarin, aspirin, and vasodilators was used in patients who underwent CSV bypass. Grafts treated with this immunosuppressive regimen demonstrated increased patency rates. This regimen, however, was associated with an increased incidence of complications and graft aneurysmal degeneration.54 In one series of patients with prosthetic aortic infection, 10 of 30 patients who underwent aortic allograft replacement were concomitantly treated with cyclosporine. Although the measured humoral immune response was blunted in patients who received cyclosporine, no differences in graft patency or graft complication rates were appreciated.26 In contrast, Randon et al55 contended that a low-dose cyclosporine immunosuppressive regimen for lower extremity bypass using CSV was effective at reducing the risk of rejection while facilitating host cell repopulation of biograft endothelium.
Furthermore, an immunologic response evoked by a cryopreserved allograft can induce allosensitization, which may interfere with future organ transplantation. This mostly affects the use of cryopreserved femoral vein (CFV) allografts in hemodialysis access. A case-matched series of 20 patients who underwent creation of hemodialysis access with this graft demonstrated host allosensitization in all patients as measured by the panel-reactive antibody assay.56 Allosensitization, however, did not occur when the CFV graft was processed to remove cellular elements.57 Diminution of the immune response by removal of antigenic epitopes has led to multiple attempts to structurally modify biologic grafts.
Structurally Modified Biologic Grafts
In parallel with the development of cryopreservation techniques, further research was conducted to modify blood vessels so that an acceptable vascular substitute could be developed. The goal was to transform a harvested blood vessel into a durable nonimmunogenic graft that could be easily produced and stored. During early experiments in the 1950s, animal arteries were modified by enzymatic digestion of the musculoelastic portion of the vessel wall with ficin, a proteolytic enzyme isolated from figs, to remove immunologically reactive proteins. The resultant collagenous vascular skeleton was strengthened by collagen cross-linking through subsequent tanning with dialdehyde starch.21,58 This modified graft was then sterilized and stored in a 1% propylene oxide–50% ethanol solution.21
In the earliest experiments, modified BCA grafts were implanted as xenografts first in dogs and then in patients with symptomatic lower extremity occlusive disease. Although no graft ruptures had occurred at 3 years of follow-up, early neointimal hyperplasia and diminished patency were observed.21 An unacceptable late rate of graft infection and aneurysmal degeneration led to a change to glutaraldehyde-based tanning protocols.58,59
Bovine mesenteric veins (BMVs) have also been modified by a patented process of glutaraldehyde cross-linking and sterilized by γ radiation.60 Both BCAs and BMVs have been used as xenografts in a number of clinical applications.
HUV is a modified biologic conduit that was first evaluated in baboons22 in the early 1970s and subsequently used in humans22,61 in 1975. Umbilical vessels are uniform in caliber, valveless, and branchless. The umbilical vein was removed from the umbilical cord by a variety of techniques, including enzymatic digestion and mechanical stripping. After a rinsing process with a cold isotonic solution, this vein was tanned with glutaraldehyde. A polyester fiber mesh was then sutured in place about the length and outside circumference of the graft for added support.22
Although thrombosis and aneurysm formation were common in early experiments, tanning and external support modifications significantly reduced these complications. Increased adherence of platelets to the luminal surface of these grafts has been observed in a canine model.62 Histologic analysis of modified HUV grafts explanted from baboons revealed an early neutrophil and late macrophage response in the vicinity of the surrounding polyester mesh. The inner collagen layer appeared thickened and dense, but was free of significant inflammation.22 The reduced immunogenicity of this graft was hypothesized to be secondary to pretreatment with glutaraldehyde, which was thought to bind to graft histocompatibility antigen sites and thereby shielded them from the host immune response.63
Other Grafts
The search for an ideal blood vessel substitute led to the investigation of a number of nonconventional biologic grafts in animal models. Vascular prostheses fashioned from pericardium64 and small intestinal mucosa65 have been evaluated. Chemically modified human66 and bovine67 ureters have been used as vascular conduits with some success. Modified bovine ureters have been used clinically with acceptable patency rates in femoral to popliteal bypass in one small Australian series.68 In addition, a small randomized trial claimed clinical equivalence between bovine ureters and PTFE used for hemodialysis access in patients with no vein options.69
Clinical Use in Peripheral Vascular Surgery
Indication
Biologic grafts have been used in modern peripheral vascular surgery mostly in three distinct clinical settings: extremity bypass in the absence of suitable autogenous conduit, arteriovenous (AV) access for hemodialysis, and replacement of infected prosthetic grafts.
Extremity Bypass
Acute or chronic ischemia of an extremity is caused by a number of conditions, including atherosclerosis, trauma, embolization, and in-situ thrombosis. Treatment of extremity ischemia involves revascularization by endovascular or surgical techniques. During infrainguinal surgical bypass, the choice of conduit is crucial to success of the operation. The autogenous great saphenous vein has proven to be the preferred conduit for infrainguinal revascularization.70,71 When the autogenous great saphenous vein is not available, alternative autogenous conduits, such as an arm vein,72 the small saphenous vein,73 and the composite autogenous vein,74 have been used with good results. The ever-increasing age and complexity of patients with infrainguinal arterial occlusive disease has brought about increasingly frequent clinical scenarios in which the autogenous vein is not available and an alternative conduit must be found. Although prosthetic grafts have been used with moderate success above the knee, they have been disappointing when used for infrageniculate bypass.75,76 Distal modification of prosthetic grafts with a vein cuff or distal AVF may improve patency rates,77–79 but is more cumbersome.
Given the absence of reliable conduit options for infrageniculate bypass when a suitable autogenous vein is lacking, the feasibility of biologic grafts has been evaluated. In this setting, CSV allografts,80 cryopreserved femoro-popliteal artery (CFA) allografts,42 HUV grafts,81 BCA xenografts,58 and BMV xenografts82 have been used with varying degrees of success.
Arteriovenous Access
End-stage renal disease is a significant public health problem in the United States; its prevalence is increasing steadily, and it is forecast that by the year 2030, more than 2 million patients will be undergoing hemodialysis.83,84 Long-term hemodialysis is best performed through a surgically created AVF that connects the arterial and venous circulations via a conduit. The ideal AV conduit carries high flow for efficient dialysis, is superficial enough for easy access, is sufficiently durable to withstand multiple cannulations, allows rapid sealing of cannulation sites, and is resistant to infection, stenosis, and thrombosis. A mature, native vein AVF comes closest to the ideal, and its use is strongly encouraged.11 Unfortunately, many individuals lack suitable veins for native AVF construction because of small vein size, previous access procedures, or vein harvest for peripheral or coronary bypass. Furthermore, up to 60% of native AVFs fail to mature, and therefore, cannot be used successfully.85,86 Although prosthetic AV grafts are widely used, they have lower patency rates, require more frequent revision, and are at higher risk than vein AVFs for infection.87 The search for optimal hemodialysis access in patients who are not candidates for a native vein AVF has led to the use of biologic grafts. CFV allografts,88 BCA xenografts,89 bovine ureter xenografts,69 and BMV xenografts60 have been used in a variety of settings with variable results.
Replacement of Infected Prosthetic Grafts
Prosthetic graft infection, particularly when the aorta is involved, is one of the most dreaded complications in vascular surgery and is associated with high morbidity and mortality.90 Treatment of an infected aortic prosthesis includes excision of the infected segment and extra-anatomic prosthetic bypass90 or reconstruction with an antibiotic-soaked or antibiotic-bonded prosthetic graft,91 femoral vein,92 or aortic allograft.93 Extra-anatomic bypass and aortic ligation are associated with long operative times, risk of remote infection, bypass thrombosis, and aortic stump rupture.90,94 Antibiotic-soaked prosthetic grafts may work well for infections caused by relatively indolent Staphylococcus epidermidis, but are much less effective against more virulent organisms.91 Finally, use of the femoral vein for aortic reconstruction is tedious and associated with harvest-related complications.92
Cryopreserved aortic allografts offer “off-the-shelf” availability, good handling properties, and the potential for expeditious in-situ repair. Cryopreserved aortic allografts were more resistant than prosthetic grafts to S. epidermidis infection in a canine model.95 Resistance of vascular allografts to infection has led to the wide use of arterial allografts to treat aortoiliac infection,96 CFV allografts to treat infection involving prosthetic AV grafts,97 and CSV allografts to replace infected infrainguinal prosthetic bypass grafts.98
Biologic Graft Preparation
Cryopreserved Allografts
A number of tissue banks and commercial companies prepare, store, and supply cryopreserved blood vessels. Despite similarities in conduit preparation, many have proprietary cryopreservation protocols.99 The great saphenous vein, femoral vein, and arterial segments are harvested from multiorgan donors who are screened for an array of viral, bacterial, and fungal infections. Branches are suture ligated, and the allografts are sized with calibrated dilators. They are tested for presence of pathogens, rinsed in an antibiotic solution, placed in a proprietary cryoprotectant solution, and stored in the vapor phase of liquid nitrogen at −110°C to −196°C. Allografts are shipped and stored in a solution of dimethylsulfoxide at −96°C until needed. The vein, but not artery, is usually matched for ABO/Rh compatibility with the recipient to decrease the risk of rejection. At the start of the procedure, the allograft is rapidly thawed by submersion in a warm water bath at 37°C to 42°C for 20 minutes. After rinsing in a series of solutions provided by the manufacturer, it is ready for use.
CSV allografts are available in a number of lengths and diameters. Most commonly, the vein measures 3 to 5 mm in diameter. These grafts look, feel, and handle like the autogenous saphenous vein. During an infrainguinal bypass, the allograft is usually reversed and placed in a superficial tunnel for easy access.80 Postoperative surveillance was not considered useful in one large series.80
CFV allografts are usually less than 25 cm in length and have a diameter between 5 and 7 mm. They have most commonly been used in hemodialysis, particularly in the setting of prosthetic AV graft infection. When used for dialysis access, this allograft is appropriately reversed and tapered to a 5 mm diameter at the arterial anastomosis to decrease the incidence of ischemic steal syndrome.88 It is allowed to mature for 3 to 4 weeks before it is accessed for hemodialysis. Revision of these grafts is very difficult because of their thin wall and surrounding fibrosis.97
Arterial allografts have most frequently been used for aortic replacement in the setting of primary or prosthetic aortic infection. Given this clinical setting and the need to replace a large artery such as the aorta, these allografts have to withstand particularly hostile conditions. Technical modifications for the use of these allografts have been developed, including vigilance in following thawing instructions, use of appropriately long grafts, and construction of tension-free anastomoses, taking great care that suture ligation of branches is performed with polypropylene sutures that include the graft wall along with the branches, aggressive excision of infected tissue and wound drainage, circumferential anastomotic reinforcement with allograft strips, use of gentamicin-impregnated fibrin glue, and coverage of the graft with viable tissue, such as a pedicled omental or muscle flap.93,100
Structurally Modified Allografts
The HUV graft (Fig. 94-1) is shipped and stored in 50% ethanol and provided on a glass mandril. It is irrigated up to 10 times with a low-molecular-weight dextran–containing solution and rinsed with a high-concentration heparin solution (10,000 U/L). This graft does not tolerate traction or the application of standard vascular clamps. To avoid injury, it needs to be passed through a metal or plastic conduit during tunneling and preferably controlled with a tourniquet. To decrease the risk of pseudoaneurysm formation during suturing, both the vein and the Dacron mesh need to be incorporated into the suture line. Finally, infusion of low-molecular-weight dextran has been recommended in the early postoperative period by the manufacturer to decrease the risk of early thrombosis.
Manufacturing of the umbilical vein graft by Synovis Life Technologies, Inc. (St. Paul, Minn) stopped in May 2005 in compliance with new U.S. Food and Drug Administration (FDA) guidelines governing combination tissue–medical devices.101 To date, a next-generation HUV graft that will fulfill FDA regulations is not yet available, although developers are actively seeking corporate industry support for such a venture (Herbert Dardik, personal communication, December 13, 2012).
The BCA graft (Fig. 94-2) is supplied in a specially designed tube containing a proprietary solution of 1% propylene oxide in 40% aqueous ethyl alcohol. It is naturally compliant, soft, and relatively easy to use. It is presently available in 6-, 7-, and 8-mm diameters and 15- to 50-cm lengths (Artegraft; Artegraft, Inc., New Brunswick, NJ). The BMV graft (Fig. 94-3) is shipped in a sterile saline solution and is available in 6-mm diameters and 10- to 40-cm lengths (ProCol Vascular Bioprosthesis, Hancock Jaffe Laboratories, Irvine, Calif). This graft is compliant and handles much like a saphenous vein.60 Both xenografts require a series of rinsing steps in the operating room before use.
< div class='tao-gold-member'>
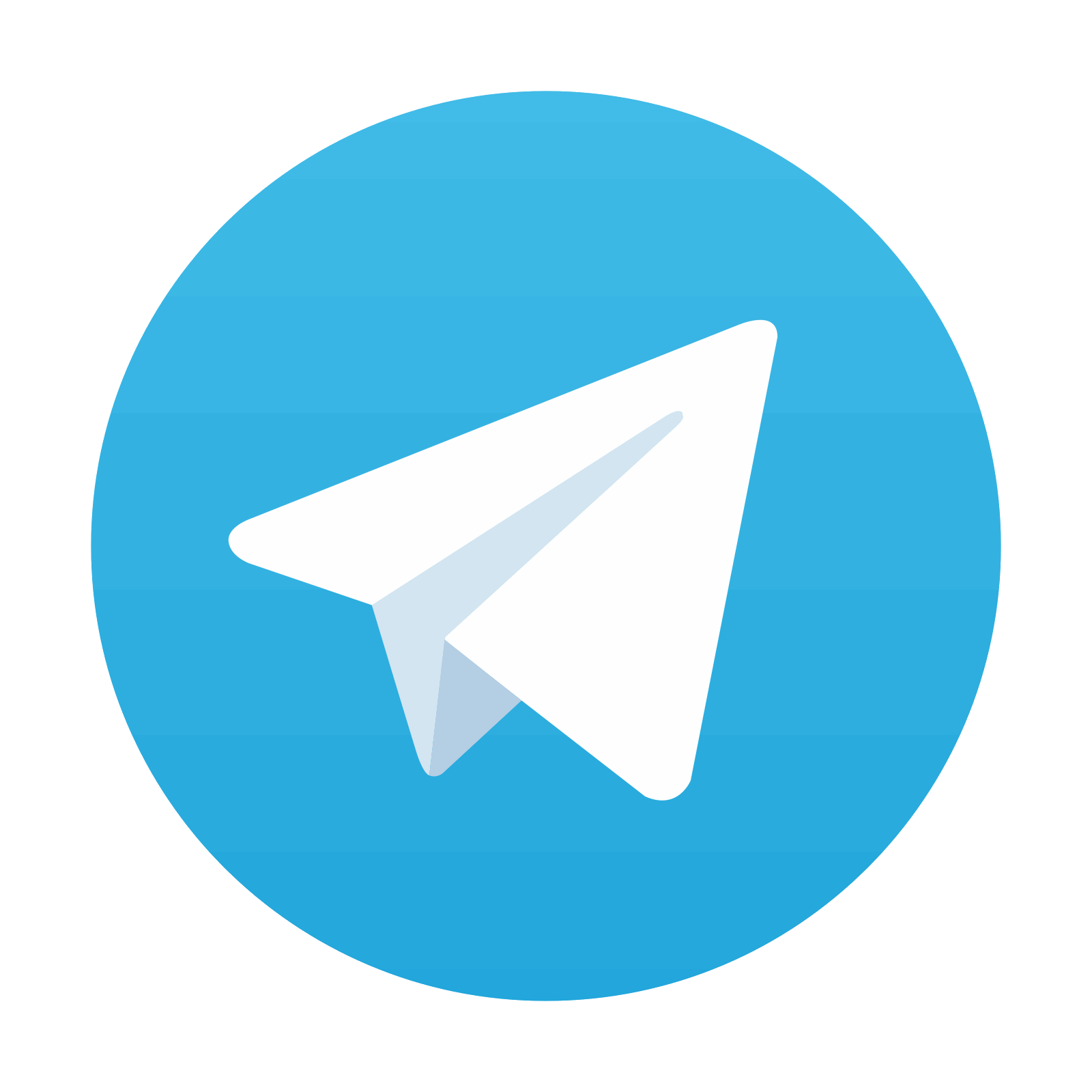
Stay updated, free articles. Join our Telegram channel

Full access? Get Clinical Tree
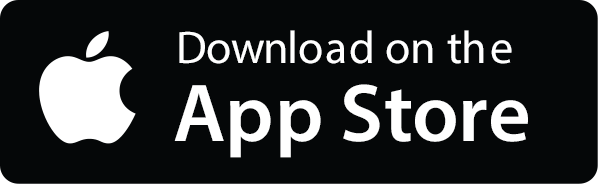
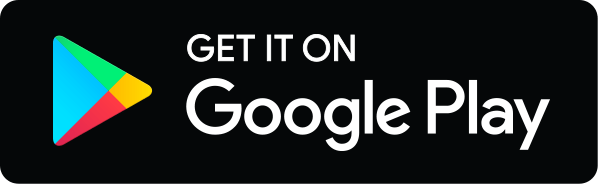