Fig. 14.1
[99mTc]-MIBI (upper) and 15 min (middle) and 3 h (lower) [123I]-MIBG SPECT images and polar maps from a patient with inferior myocardial infarction. Both 15 min and 3 h [123I]-MIBG images show a larger inferior defect than [99mTc]-MIBI perfusion images. SA indicates short axis, VLA vertical long axis, HLA horizontal long axis
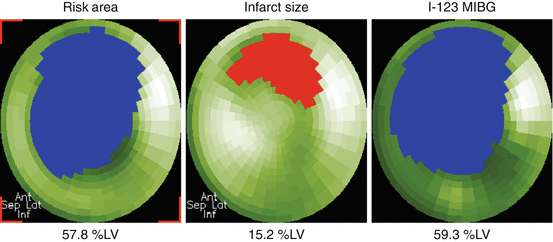
Fig. 14.2
Polar maps of myocardium at risk (left) and infarct size (center) and [123I]-MIBG (right) images from a patient with anterior myocardial infarction. Quantified defect areas on each polar map are filled with blue or red color, and measured defect sizes are expressed as the percentage of left ventricular myocardium (%LV)
Using PET and [11C]-mHED as a tracer for cardiac sympathetic presynaptic function, Allman et al. have demonstrated a more extensive area of [11C]-mHED abnormalities than that of blood flow, which is essentially similar to the observations reported using [123I]-MIBG single photon emission computed tomography (SPECT) (Allman et al. 1993). Although [11C]-mHED PET is technically more demanding, including the necessity for on-site cyclotron, it consistently yields better quality images and appears to be more suitable for assessing regional abnormalities than [123I]-MIBG SPECT as typically illustrated in Fig. 14.3 (Matsunari et al. 2010). Furthermore, although there is a close correlation between [123I]-MIBG heart-to-mediastinum (H/M) uptake ratio and [11C]-mHED retention (Matsunari et al. 2010), PET imaging using presynaptic sympathetic tracers such as [11C]-mHED or [11C]-epinephrine provides the possibility of absolute quantification of cardiac sympathetic innervation on regional basis by routine use of attenuation/scatter correction. This feature may become important when regional, rather than global, cardiac sympathetic innervation is of concern (Sasano et al. 2008). However, clinical experience with PET autonomic imaging is still limited, and therefore more work needs to be done to determine its clinical role in assessing myocardial infarction.
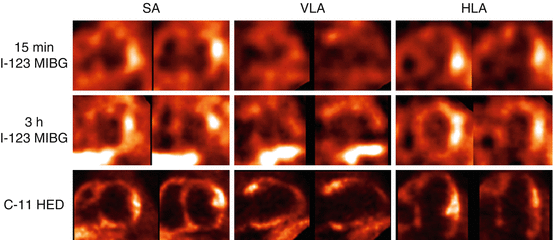
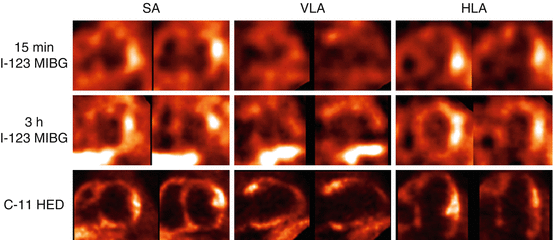
Fig. 14.3
[123I]-MIBG SPECT (upper and middle) and [11C]-mHED PET (lower) images from a male patient with anterior and inferior myocardial infarction. The quality of 15 min and 3 h [123I]-MIBG SPECT and [11C]-mHED PET images was judged as fair, poor, and good, respectively. In particular, the low myocardial tracer uptake and intense adjacent liver activity on the late [123I]-MIBG SPECT degrade the image quality. SA indicates short axis, VLA vertical long axis, HLA horizontal long axis
14.3 Autonomic Imaging in Remote Myocardium in Patients After Myocardial Infarction
After myocardial infarction, the left ventricular remodeling involves not only the infarcted but also non-infarcted myocardium. From a therapeutic viewpoint, attenuation of remodeling process is expected to result in reduced morbidity and better prognosis (Pfeffer and Braunwald 1991). Therefore, characterization of such non-infarcted myocardium is important as a target of medical treatments. To date, however, there is only limited data available in literature that focused on remote myocardium after myocardial infarction. A planar [123I]-MIBG imaging study in patients after acute myocardial infarction has demonstrated a faster myocardial [123I]-MIBG washout involving remote myocardium as compared with normal subjects, indicating increased sympathetic nerve tone (Bengel et al. 1999). Spyrou et al. performed PET imaging using [11C]-CGP-12177, a β-adrenergic receptor ligand, in patients soon after myocardial infarction and found that β-adrenergic receptor density was decreased in both infarcted and remote myocardia (Spyrou et al. 2002). They also found that the degree of β-adrenergic receptor density reduction was correlated with later left ventricular dilation. The downregulation of postsynaptic β-adrenergic density in remote myocardium was also confirmed in a PET study by Ohte et al. (2012). In a study using [123I]-MIBG SPECT by Sakata et al., patients with left ventricle (LV) dilatation had more severe [123I]-MIBG abnormality than those without left ventricular dilatation, suggesting the contribution of cardiac sympathetic neuronal function in left ventricular remodeling (Sakata et al. 2000). However, sympathetic innervation in non-infarcted myocardium could not be assessed in that study, because absolute quantification of tracer uptake was not possible with [123I]-MIBG imaging due to non-quantitative nature of SPECT, which usually relies on relative rather than absolute uptake values. Caldwell et al. performed PET imaging using [11C]-mHED and [11C]-CGP-12177 in patients with ischemic heart failure and found decreased presynaptic function combined with a small decrease in postsynaptic function (mismatch) in non-infarcted myocardium (Caldwell et al. 2008). Thus, autonomic dysfunction in non-infarcted myocardium may play a role in the pathogenesis of left ventricular remodeling.
14.4 Autonomic Imaging in Myocardial Ischemia Without Infarction
14.4.1 Stable Coronary Artery Disease
Although many of the studies on autonomic imaging in CHD mainly focused on patients after myocardial infarction, cardiac sympathetic nerve terminals are more sensitive to ischemia than myocardial cells as described earlier (Matsunari et al. 2000). Therefore, it is conceivable that ischemic sympathetic neuronal damage would occur even in the absence of infarction. This was confirmed by clinical studies demonstrating [123I]-MIBG defects exceeding perfusion defects in patients without a history of myocardial infarction (Guertner et al. 1993; Hartikainen et al. 1997). Similar results were observed using [11C]-mHED PET (Bulow et al. 2003). This concept was also supported by an experimental study by Luisi et al. using a pig model of chronic hibernation without infarction showing extensive defects in [11C]-mHED uptake in the area of hibernating but viable myocardium (Luisi et al. 2005).
14.4.2 Vasospastic Angina
Vasospastic angina is a form of angina characterized by a sudden spasm of one of the coronary arteries without significant stenosis. It is known that vasospastic angina is often associated with altered autonomic nervous activity (Ricci et al. 1979). Because coronary vasospasm causes a transient ischemia in that vascular territory, such an ischemic episode may cause reduction in [123I]-MIBG uptake despite preserved perfusion. The results of meta-analysis using Meta-DiSc software (Zamora et al. 2006) from seven published studies (Ha et al. 1998; Hirano et al. 2002; Inobe et al. 1997; Sakata et al. 1997; Takano et al. 1995; Taki et al. 1998; Watanabe et al. 2002a) that reported the diagnostic value of [123I]-MIBG imaging are summarized in Fig. 14.4. The pooled estimates of sensitivity and specificity were 77 % (95 % confident intervals: 70–82 %) and 77 % (95 % confident intervals: 70–83 %), respectively. The area under the summary receiver operating characteristic analysis (SROC) curve and its Q*-point were 89 ± 5% and 82 ± 5 %, respectively. However, both sensitivities and specificities had significant heterogeneity (P < 0.0001 for both), as is evident from the wide range (42–100 % for sensitivity and 40–100 % for specificity) and the high inconsistency index (90.8 % for sensitivity and 80.8 % for specificity). The heterogeneity in diagnostic performance is also evident from the wide 95 % confidence intervals in SROC curves. In a study by Sakata et al., the use of lower [123I]-MIBG washout rate rather than reduced [123I]-MIBG uptake as a marker of vasospastic angina significantly improved sensitivity and specificity from 42 to 76 % and 72 to 87 %, respectively, indicating that cardiac sympathetic nerve activity in vasospastic angina is suppressed probably because of the enhanced parasympathetic nerve activity (Sakata et al. 1997). Furthermore, a subsequent study from the same authors has demonstrated that high H/M ratio or lower washout rate was a marker of future cardiac events (Sakata et al. 2005). By contrast, Inobe et al. have demonstrated that the patients with higher disease activity were frequently associated with either the uptake reduction or the abnormally high washout of [123I]-MIBG (Inobe et al. 1997). Although these inconsistent published results could be explained in part by different patient characteristics such as disease severity and duration and by different methodologies among the studies (Ha et al. 1998; Hirano et al. 2002; Inobe et al. 1997; Sakata et al. 1997; Takano et al. 1995; Taki et al. 1998; Watanabe et al. 2002a), the exact underlying mechanisms are still unclear. Thus, the clinical use of autonomic imaging in vasospastic angina remains to be established by further work.
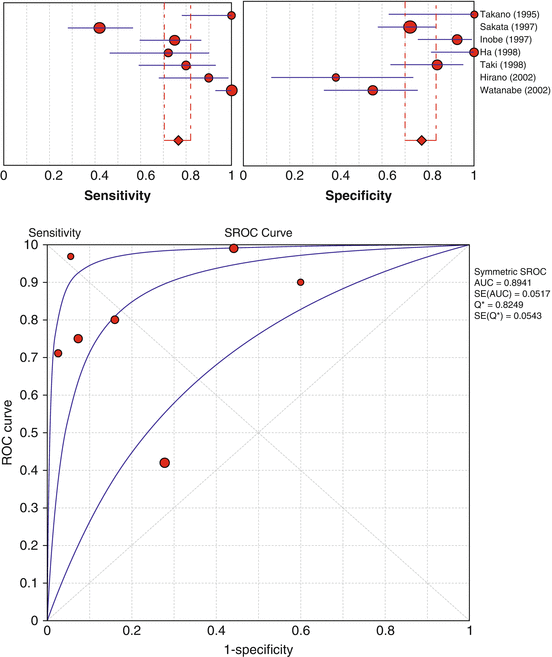
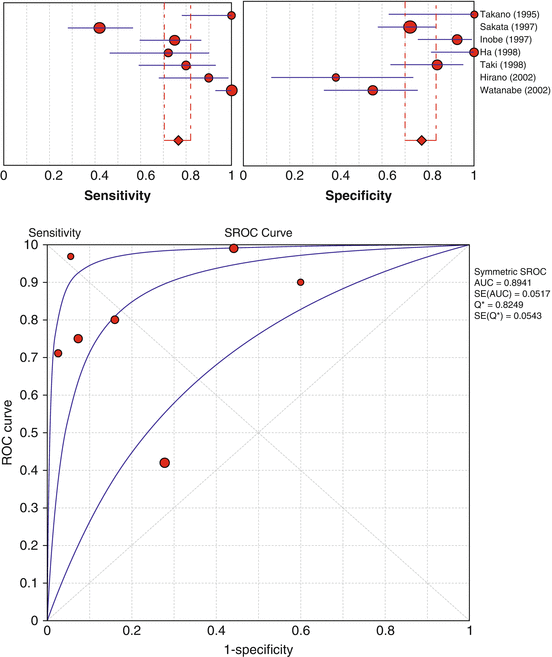
Fig. 14.4
Forrest plot of sensitivities (upper left) and specificities (upper right) from published [123I]-MIBG studies in the diagnostic performance of vasospastic angina on patient basis and summary receiver operating characteristic (SROC) curve (lower). Each red circle represents individual study in meta-analysis, with size of circle proportional to sample size of study. Red diamond denotes an estimate of pooled values with 95 % confidence intervals (CI) in red line. Blue lines in the forest plots represent 95 % CI of each study. Best-fit curve (middle curve) in the SROC curve lies between two curves that demarcate its 95 % CI
14.4.3 Silent Myocardial Ischemia
Silent myocardial ischemia is defined as evidence for ischemia without symptoms such as chest pain (Gutterman 2009). It is important to note that patients with silent myocardial ischemia often have a poor prognosis as compared with those with painful ischemia. Therefore, early detection and monitoring of patients with silent myocardial ischemia before hard cardiac events is clinically relevant. Although the exact mechanism is yet to be determined, impairment in cardiac sympathetic innervation is likely to be linked with the occurrence of silent myocardial ischemia. In this regard, autonomic imaging may play a role for characterization of silent ischemia. However, published results focusing on this topic using [123I]-MIBG imaging are inconclusive so far. In a study by Shakespeare et al. using delayed (4 h) [123I]-MIBG SPECT, no evidence of denervation was observed in silent ischemia (Shakespeare et al. 1993). Hartikainen et al. found that the patients with silent ischemia revealed smaller areas of viable but denervated myocardium than those with chest pain (Hartikainen et al. 1994). Studies by other groups (Langer et al. 1995; Matsuo et al. 1996; Shimonagata et al. 1995), however, have suggested a link between silent ischemia and abnormalities on [123I]-MIBG SPECT. A study by Langer et al. have demonstrated that diabetic patients with silent myocardial ischemia had evidence of a diffuse abnormality in [123I]-MIBG uptake (Langer et al. 1995). A subsequent study by Matsuo et al. showed decreased [123I]-MIBG uptake particularly in the inferior wall in patients with silent ischemia (Matsuo et al. 1996). All these studies were based on relatively small patient sample size, which may have contributed to the inconclusive results among the studies. It should also be noted that all these studies mainly relied on interpretation of [123I]-MIBG SPECT images, where accurate assessment of regional abnormalities may sometimes be difficult due to poor image quality (Matsunari et al. 2010). Thus, much work still needs to be done to determine the value of autonomic imaging to characterize silent myocardial ischemia.
14.5 Reinnervation After Myocardial Infarction or Ischemia
Restoration of cardiac catecholamine uptake and storage site (i.e., reinnervation) is known to occur after heart transplantation (Schwaiger et al. 1991), where postganglionic sympathetic nerve fibers of the donor heart are interrupted, resulting in complete denervation. It is also known that reinnervation following heart transplantation is partial and not consistently observed (Bengel et al. 2001). In an experimental dog study by Minardo et al., [123I]-MIBG scintigraphic images returned to normal at 14 weeks after myocardial infarction, which is consistent with the presence of reinnervation (Minardo et al. 1988). However, in a pig model of myocardial hibernation (Fallavollita et al. 2010), [11C]-mHED defects persisted despite functional improvement at 4 weeks after successful percutaneous coronary intervention or pravastatin therapy, indicating the lack of plasticity of sympathetic neurons within a short term. Likewise, conflicting results have been published as to whether and when reinnervation occurs after myocardial infarction as summarized in Table 14.1. Allman et al. performed [11C]-mHED PET in patients after myocardial infarction and did not observe changes in [11C]-mHED defect size between 1 week and 8 months (Allman et al. 1993). By contrast, Hartikainen et al. found partial reinnervation using [123I]-MIBG SPECT in peri-infarcted area during the follow-up period of 12 months (Hartikainen et al. 1996). In patients with stable coronary heart disease undergoing percutaneous coronary intervention, Guetner et al. performed [123I]-MIBG SPECT before and 3–4 months after the intervention and observed partial reinnervation in 5 of 16 (31 %) patients (Guertner et al. 1993). All of these studies were based on relatively small sample sizes and were performed more than a decade ago using older-generation cameras. It is also important to note that sympathetic dysinnervation after ischemic insult can be either functional (stunning), structural (anatomical denervation), or both (Fallavollita and Canty 2010), which may affect the likelihood and time course of reinnervation. Thus, whether and how reinnervation occurs as well as its clinical implications after myocardial infarction or ischemia remain to be determined by further studies.
Table 14.1
Summary of studies on reinnervation after acute myocardial infarction
Author | Publication year | Tracer | Number of patients | Imaging time points | Reinnervation | Remarks |
---|---|---|---|---|---|---|
Allman et al. | 1993 | [11C]-HED | 8 | 1 week to 8 months | No | |
Podio et al. | 1995 | [123I]-MIBG | 10 | 1 week to 30 months | No | |
Hartikainen et al. | 1996 | [123I]-MIBG | 13 | 3–12 months | Yes? | Only in peri-infarcted area |
Abe et al. | 1997 | [123I]-MIBG | 1 | 2 weeks to 12 months | Yes | A case report |
Fallen et al. | 1999 | [18F]-fluorodopamine | 10 | 2 weeks to 6 months | Yes | |
Simula et al. | 2000 | [123I]-MIBG | 15 | 1 week to 3 months | No |
14.6 Clinical Applications
14.6.1 Prognostic Value
There is an increasing body of evidence that autonomic imaging using [123I]-MIBG provides important prognostic information in patients with heart failure (Agostini et al. 2008; Jacobson et al. 2010; Kuwabara et al. 2011; Merlet et al. 1992; Nakata et al. 1998; Verberne et al. 2008). Patients with a reduced late H/M ratio or increased washout rate are likely to be associated with poor prognosis. Although there are only a few data available that have specifically looked at patients with coronary heart disease (Kasama et al. 2011; Wakabayashi et al. 2001), many published studies regarding prognostic value of [123I]-MIBG imaging included a considerable number of ischemic heart failure patients. In a large prospective study (ADMIRE-HF) by Jacobson et al. involving 961 patients, for example, 61 % of total study population had ischemic heart failure (Jacobson et al. 2010). Additionally, it is known that myocardial adrenergic nerve activity measured by [123I]-MIBG imaging is accelerated in proportion to severity of heart failure, independent of the underlying cause (Imamura et al. 1995). Furthermore, in a study by Kasama et al. that focused on patients with ST-segment elevation myocardial infarction, increased [123I]-MIBG washout rate was a significant predictor of cardiac events, independent of left ventricular ejection fraction (LVEF) (Kasama et al. 2011). Thus, it is possible that [123I]-MIBG imaging predicts prognosis in patients with ischemic heart disease as does in general heart failure population. However, it should be noted that the cutoff thresholds of H/M ratio to identify high-risk patients may not be identical between ischemic and nonischemic heart failure, as demonstrated in a study by Wakabayashi et al. (2001).
At present, data on the prognostic value of autonomic PET imaging in CHD is still limited. Pietila et al. performed [11C]-mHED PET imaging in 46 chronic heart failure (CHF) patients (72 % of total population were CHD) and found that a low [11C]-mHED retention was a predictor of poor prognosis (Pietila et al. 2001). Gaemperli et al. have found that reduced myocardial β-adrenergic receptor density measured by [11C]-CGP-12177 PET was associated with development of CHF during a median follow-up period of 12.7 years (Gaemperli et al. 2010). Thus, autonomic PET imaging also seems to be promising for risk stratification in CHD patients.
14.6.2 Therapy Monitoring and Prediction of Therapy Response
14.6.2.1 Medical
[123I]-MIBG imaging has been used for monitoring of medical therapies such as beta-blockers in heart failure patients (Agostini et al. 2000; Gerson et al. 2002; Kasama et al. 2003; Kramer et al. 1999; Lotze et al. 2001; Nakata et al. 2005; Toyama et al. 2003, 2008; Watanabe et al. 2002b; Yamazaki et al. 2001). Likewise, it should be possible that autonomic imaging can be used to monitor medical therapies in ischemic heart failure. In patients with acute myocardial infarction, Kasama et al. (2005, 2007) have demonstrated improved [123I]-MIBG defect score, H/M ratio, and washout rate after short- or long-term nicorandil therapy. [123I]-MIBG imaging has also been used to predict therapy response in heart failure patients (Choi et al. 2001; Fujimoto et al. 2004; Fukuoka et al. 1997; Kakuchi et al. 1999; Suwa et al. 1997), where those with higher H/M ratio and/or lower washout rate are likely to improve after treatment. However, there is only limited data available that specifically focused on the predictive power of [123I]-MIBG imaging in patients with coronary heart disease.
14.6.2.2 Cardiac Resynchronization Therapy
Cardiac resynchronization therapy (CRT) has emerged as an effective option to treat patients with drug-refractory heart failure and left ventricular desynchrony (Abraham et al. 2002; St John Sutton et al. 2003). In general heart failure population, [123I]-MIBG imaging is known to be useful in monitoring CRT response (Burri et al. 2008; Cha et al. 2008; Erol-Yilmaz et al. 2005; Higuchi et al. 2006; Shinohara et al. 2011) and in selecting patients who are likely to improve in response to CRT (Cha et al. 2011; Nishioka et al. 2007; Tanaka et al. 2012). Patients with significant response to CRT are likely to be associated with improved H/M ratio and slowed washout on [123I]-MIBG imaging after therapy, whereas those with no response are with no improvement in these imaging parameters. Furthermore, patients with preserved [123I]-MIBG H/M ratio and slower washout rate are likely to be associated with significant improvement in function after CRT. These concepts may also apply to the patients with ischemic heart failure. However, there are no studies that specifically looked at patients with ischemic heart failure, and therefore more work needs to be done to clarify the value of [123I]-MIBG imaging in this clinical setting.
14.6.2.3 Implantable Cardioverter Defibrillators (ICDs)
Sudden cardiac death (SCD) due to lethal arrhythmia represents an important cause of mortality in patients with CHD (Deo and Albert 2012). Implantable cardioverter defibrillators (ICDs) have emerged as novel devices to prevent SCD (Mirowski et al. 1980). Generally accepted criteria for ICD therapy include severely depressed LVEF (30–40 %) in patients with coronary heart disease, history of cardiac arrest due to ventricular arrhythmias, sustained ventricular tachycardias, positive electrophysiologic testing, and the like. However, many patients who die from SCD actually have a low-risk profile and are missed for diagnosis by the current LVEF-based criteria. Thus, we still need a better strategy to identify high-risk CHD patients for SCD who are most likely to benefit from ICD therapy. In an animal model of post-infarct ventricular tachycardia, Sasano et al. have demonstrated that the degree of perfusion/innervation mismatch was significantly correlated with the earliest site of ventricular activation during ventricular tachycardia (Sasano et al. 2008). In patients after acute myocardial infarction, Simoes et al. have demonstrated a significant correlation between the extent of sympathetically denervated but viable myocardium and electrophysiological parameters characterizing repolarization and depolarization (Simoes et al. 2004). Bax et al. performed [123I]-MIBG imaging and electrophysiologic testing in patients with a history of myocardial infarction and depressed LV function and found a significant relationship between late [123I]-MIBG defect score and inducibility of sustained ventricular arrhythmias (Bax et al. 2008). These observations suggest that autonomic imaging may have a role to identify high-risk CHD patients for SCD. This concept was further supported by subsequent [123I]-MIBG imaging studies involving patients with ICD placement, in whom detailed information on arrhythmic events is easily available (Boogers et al. 2010; Nishisato et al. 2010). Thus, although further prospective large studies are required, autonomic imaging seems to be useful to identify candidates for ICD placement in CHD.
14.6.2.4 Effects of Revascularization on Cardiac Sympathetic Innervation in Stable CHD
Although coronary revascularization is a powerful therapeutic option to treat patients with stable CHD, there are only limited data available that have focused on the effect of revascularization on cardiac sympathetic innervation. As described in earlier Sect. 14.5, inconsistent results have been published as to whether reinnervation occurs after coronary revascularization (Fallavollita et al. 2010; Guertner et al. 1993).
Transmyocardial laser revascularization (TMR) is another therapeutic option particularly for patients with severe CHD with refractory angina (Frazier et al. 1999). Although TMR alone may not necessarily improve survival rate, a combination of TMR and coronary revascularization is reportedly useful in relieving angina as compared to traditional revascularization therapy (Fihn et al. 2012). There have been several studies that investigated the effects of TMR on cardiac sympathetic innervation. Al-Sheikh et al. performed [11C]-mHED PET imaging before and after TMR and found that TMR causes LV cardiac sympathetic denervation without affecting myocardial blood flow at rest or during stress, suggesting that the improvement in angina may be due to sympathetic denervation (Al-Sheikh et al. 1999). Subsequent experimental (Le et al. 2007) and clinical (Beek et al. 2004; Muxi et al. 2003; Teresinska et al. 2004) studies using [123I]-MIBG have confirmed TMR-induced denervation, although one experimental study using swine model and autoradiography did not show significant reduction in [125I]-MIBG uptake 3 days after the treatment (Johnson et al. 2002). Furthermore, similar to those reported for coronary revascularization, whether reinnervation occurs after TMR is still a matter of discussion (Beek et al. 2004; Muxi et al. 2003; Teresinska et al. 2004). Thus, although regional denervation after TMR is likely to be related to angina relief, clinical value of autonomic imaging in this area remains to be determined.
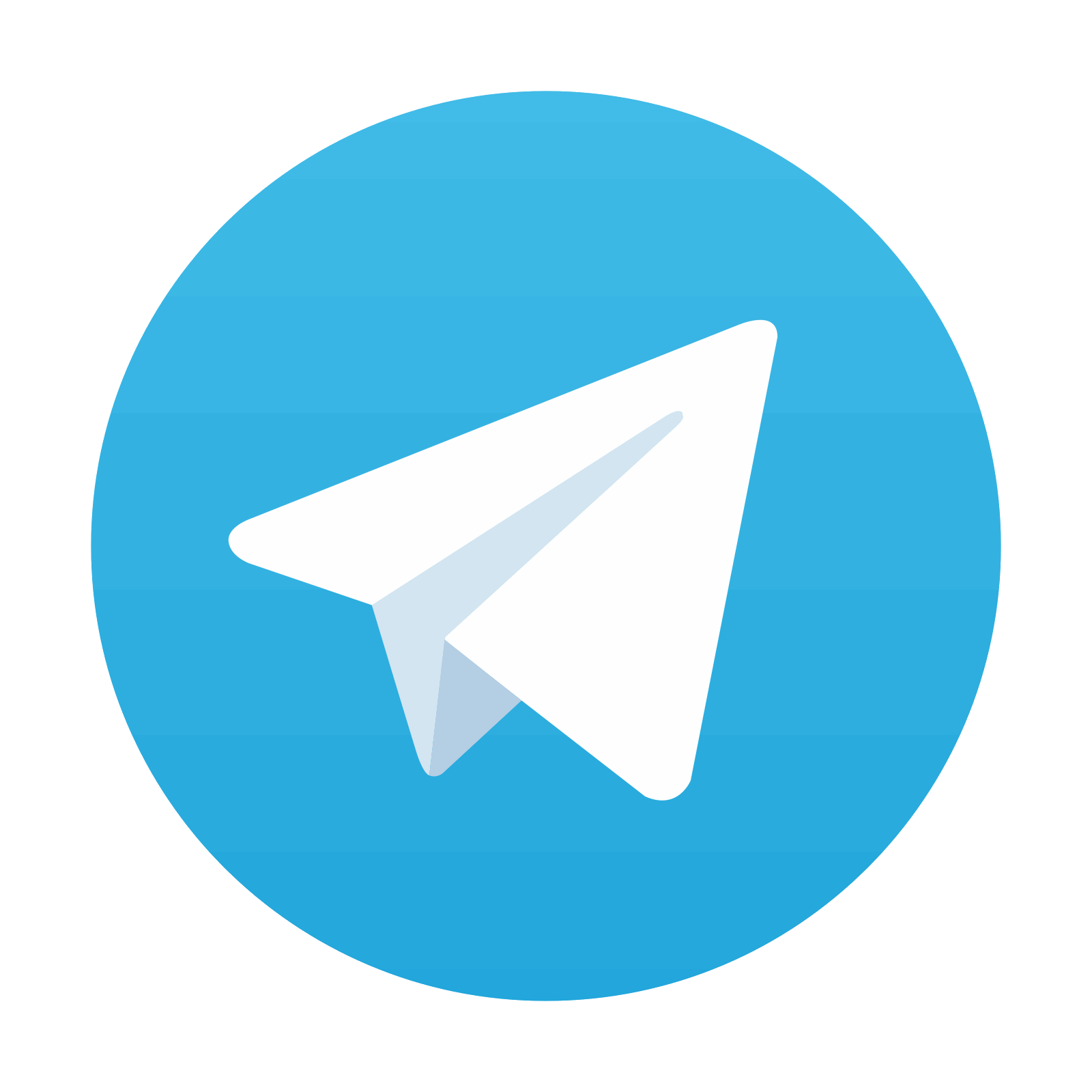
Stay updated, free articles. Join our Telegram channel

Full access? Get Clinical Tree
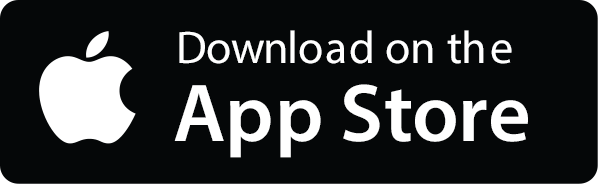
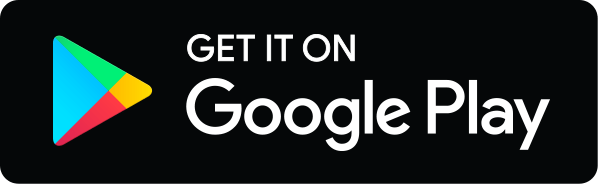